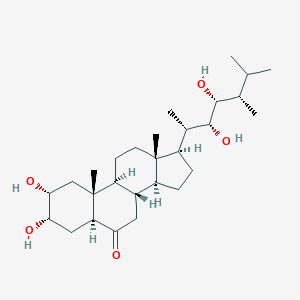
Castasterone
Übersicht
Beschreibung
Castasterone is a polyhydroxylated steroid hormone belonging to the brassinosteroid (BR) family, which plays critical roles in plant growth, development, and stress responses. It is synthesized from campesterol via the late C6-oxidation pathway, involving intermediates such as 6-deoxothis compound and 6α-hydroxythis compound . This compound serves as a direct precursor to brassinolide, the most biologically active BR, through a Baeyer-Villiger oxidation catalyzed by cytochrome P450 enzymes (e.g., CYP85A3 in tomato and CYP85A2 in Arabidopsis) . Unlike brassinolide, this compound itself exhibits bioactivity in regulating processes like cell elongation, vascular differentiation, and stress tolerance . Its levels fluctuate under environmental stresses, such as temperature extremes and heavy metal exposure, highlighting its adaptive role .
Vorbereitungsmethoden
Synthetic Preparation via Stigmasterol Derivative
Retrosynthetic Strategy and Key Intermediate
The synthesis of castasterone from stigmasterol (3) involves a convergent strategy centered on a C-22 aldehyde intermediate (7). The retrosynthetic pathway (Scheme 1) prioritizes stereochemical control at C-22, C-23, and C-24 through Sharpless epoxidation and cuprate-mediated epoxide opening . Stigmasterol, a readily available phytosterol, is first converted into aldehyde 7 via established oxidation and protection steps .
Propenyllithium Addition and Sharpless Epoxidation
The critical step involves the addition of (E)-1-propenyllithium to aldehyde 7, yielding allylic alcohol 8. Subsequent Sharpless asymmetric epoxidation using (L)-(+)-diethyl tartrate (DET) as a chiral inducer generates epoxy alcohol 15 with 63% diastereoselectivity (Table 1) . The reaction employs tert-butyl hydroperoxide (t-BuOOH) and molecular sieves in dichloromethane at −20°C, achieving an 87% yield of the threo-epoxide 15 alongside its erythro counterpart (16) .
Table 1: Diastereoselectivity in Sharpless Epoxidation of 8
Parameter | Value/Outcome |
---|---|
Chiral ligand | (L)-(+)-Diethyl tartrate |
Temperature | −20°C |
Diastereomeric ratio | 63:37 (threo:erythro) |
Yield of 15 | 63% (based on starting material) |
Cuprate-Mediated Epoxide Opening
The threo-epoxy alcohol 15 undergoes regioselective ring-opening with isopropylmagnesium chloride in the presence of catalytic CuCN. This step installs the C-24 isopropyl group with inversion of configuration, affording diol 17 in 63% yield (Table 2) . The reaction selectively targets the less hindered C-24 due to steric effects, minimizing competing attack at C-22 .
Table 2: Regioselectivity in Epoxide Opening of 15
Reagent | Selectivity (C-24:C-22) | Yield of 17 |
---|---|---|
iPrMgCl + CuCN | 5.7:1 | 63% |
Hydrolysis and Final Product Isolation
Diol 17 is hydrolyzed under acidic conditions (HCl/THF/H₂O) to yield this compound (2) with >95% purity. The overall yield from stigmasterol is 22%, making this one of the most efficient synthetic routes reported .
Natural Isolation from Zea mays Pollen
Extraction and Solvent Fractionation
This compound is isolated from corn pollen through sequential solvent extraction. Fresh pollen (330 g) is ground and extracted with 80% aqueous acetone, followed by partitioning into chloroform to concentrate brassinosteroids . The chloroform-soluble fraction is subjected to silicic acid chromatography, yielding three bioactive fractions (Fr. a, b, c) .
Chromatographic Purification
Fraction a is further purified via Sephadex LH-20 chromatography (MeOH eluent) and reverse-phase HPLC (Nucleosil 5C18 column, acetonitrile/H₂O). Active fractions are crystallized from aqueous acetonitrile, yielding this compound identified by NMR signals at δ 0.69 (H₃-18), 0.76 (H₃-19), and 3.56–3.72 (H-22, H-23) .
Table 3: Key NMR Signals of this compound
Proton | Chemical Shift (δ) | Multiplicity |
---|---|---|
H₃-18 | 0.69 | Singlet |
H₃-19 | 0.76 | Singlet |
H-22 | 3.56 | Doublet |
H-23 | 3.72 | Doublet |
Spectroscopic Validation
GC/MS analysis of the methaneboronate derivative confirms the identity of this compound, showing a molecular ion at m/z 512 [M]⁺ and characteristic fragments at m/z 497 [M−CH₃]⁺ and 456 [M−C₄H₈]⁺ .
Comparative Analysis of Methods
Yield and Scalability
The synthetic route offers higher scalability (22% overall yield) compared to natural isolation, which is limited by pollen availability (~0.001% w/w) . However, natural isolation avoids complex stereochemical steps.
Purity and Stereochemical Control
Synthetic this compound achieves >95% purity due to precise stereochemical guidance during Sharpless epoxidation and cuprate addition . Natural extracts require rigorous chromatography to separate analogs like typhasterol and teasterone .
Analyse Chemischer Reaktionen
Arten von Reaktionen: 24-Epicastasteron durchläuft verschiedene chemische Reaktionen, darunter Oxidation, Reduktion und Substitution. Diese Reaktionen sind unerlässlich, um die Struktur der Verbindung zu modifizieren und ihre biologische Aktivität zu verbessern.
Häufige Reagenzien und Bedingungen:
Oxidation: Häufige Oxidationsmittel wie Kaliumpermanganat oder Chromtrioxid werden verwendet, um sauerstoffhaltige funktionelle Gruppen einzuführen.
Reduktion: Reduktionsmittel wie Natriumborhydrid oder Lithiumaluminiumhydrid werden verwendet, um die gewünschte Stereochemie zu erreichen.
Hauptprodukte: Die Hauptprodukte, die aus diesen Reaktionen gebildet werden, umfassen verschiedene Derivate von 24-Epicastasteron, die jeweils einzigartige biologische Aktivitäten aufweisen. Diese Derivate werden häufig in der wissenschaftlichen Forschung verwendet, um die Auswirkungen der Verbindung auf das Pflanzenwachstum und die Pflanzenentwicklung zu untersuchen .
Wissenschaftliche Forschungsanwendungen
Pharmaceutical Applications
1.1. Anti-Cancer Properties
Recent research has demonstrated that castasterone exhibits significant cytotoxic effects on human small-cell lung cancer (SCLC) cells. In a study, CAS was shown to be effective against both drug-sensitive (H69) and multi-drug resistant (VPA17) SCLC cells, with an IC50 of approximately 1.0 μM for both cell lines. Notably, CAS did not exhibit cytotoxicity toward normal lung epithelial cells (BEAS-2), indicating its potential selectivity for cancer cells .
Table 1: Cytotoxicity of this compound in Different Cell Lines
Cell Line | IC50 (μM) | Sensitivity |
---|---|---|
H69 (SCLC) | 1.0 | Drug-sensitive |
VPA17 (SCLC) | 1.0 | Multi-drug resistant |
BEAS-2 | >40 | Normal epithelial |
1.2. Reversal of Drug Resistance
This compound has been shown to reverse multi-drug resistance in SCLC cells. When combined with common chemotherapeutic agents like etoposide and doxorubicin, CAS exhibited synergistic effects, enhancing the efficacy of these drugs . This suggests that CAS may act through different biochemical pathways than traditional chemotherapy agents.
Table 2: Combination Studies with this compound
Drug Combination | Combination Index (CI) |
---|---|
CAS + Etoposide | 0.77 |
CAS + Doxorubicin | 0.85 |
CAS + Epibrassinolide | 1.07 |
Agricultural Applications
2.1. Stress Tolerance
This compound plays a crucial role in enhancing plant tolerance to abiotic stresses such as salinity and heavy metal toxicity. Studies have indicated that seed soaking with this compound can activate enzymatic defense systems in plants like Brassica juncea, leading to increased antioxidant activity and polyphenol accumulation under stress conditions .
Table 3: Effects of this compound on Antioxidant Enzymes
Treatment | Superoxide Dismutase (SOD) Activity | Polyphenol Oxidase Activity | Catalase Activity |
---|---|---|---|
Control | Baseline | Baseline | Baseline |
This compound | Increased | Increased | Increased |
2.2. Growth Enhancement
In addition to stress tolerance, this compound has been shown to enhance growth parameters in various crops by promoting cell elongation and division . Its application can lead to improved yield and quality of agricultural products.
Biotechnological Applications
3.1. Metabolic Pathways
Research into the metabolic pathways involving this compound has revealed its role as a precursor to brassinolide, the most active BR involved in plant growth regulation . Understanding these pathways can facilitate biotechnological interventions aimed at enhancing BR production in economically important crops.
Case Studies
Case Study 1: this compound in Cancer Treatment
A recent study highlighted the efficacy of this compound in reversing drug resistance in SCLC cells by inducing apoptosis through DNA fragmentation and modulation of β-catenin levels . This provides a promising avenue for developing new cancer therapies that incorporate plant-derived compounds.
Case Study 2: Enhancing Crop Resilience
In agricultural trials, this compound application significantly improved the growth and yield of Brassica juncea under copper stress conditions, showcasing its potential as a natural growth regulator in sustainable farming practices .
Wirkmechanismus
The mechanism of action of 24-Epicastasterone involves its interaction with specific receptors in plant cells, leading to the activation of various signaling pathways. These pathways regulate the expression of genes involved in cell elongation, division, and differentiation. The compound also influences the levels of key phytohormones, promoting growth and stress tolerance. The molecular targets of 24-Epithis compound include receptors and enzymes involved in the biosynthesis and signaling of auxins, salicylic acid, jasmonic acid, and abscisic acid .
Vergleich Mit ähnlichen Verbindungen
Brassinosteroids share structural similarities but differ in functional groups, biosynthesis, and biological activity. Below is a detailed comparison of castasterone with key analogs:
This compound vs. Homothis compound
- Structural Differences : Homothis compound has an additional ethyl group at the C24 position, derived from sitosterol instead of campesterol .
- Biosynthesis : this compound originates from campesterol, while homothis compound is synthesized from sitosterol via analogous pathways .
- Bioactivity :
- This compound is more hydrophilic due to fewer polar groups, enabling better membrane permeability and chloroplast localization .
- Homothis compound dominates in barley and wheat under standard conditions but accumulates less under cold stress compared to this compound .
- In model membranes, homothis compound reduces membrane fluidity more significantly than this compound, affecting stress adaptation .
This compound vs. Brassinolide
- Structure: Brassinolide contains a lactone ring at C6, absent in this compound .
- Biosynthesis: Brassinolide is synthesized from this compound via CYP85A enzymes. Arabidopsis mutants lacking these enzymes exhibit dwarfism unless supplemented with brassinolide .
- Bioactivity: Brassinolide is 100–1,000× more potent in promoting stem elongation and leaf expansion . this compound retains partial activity; in tomato, it functions as the primary bioactive BR during vegetative growth, while brassinolide is critical for fruit development .
This compound vs. 24-Epibrassinolide (EBR)
- Structure : EBR is a synthetic BR analog with a modified side chain.
- EBR upregulates antioxidant enzymes (e.g., SOD, CAT) more effectively than this compound in stress responses .
This compound vs. 28-Homodolichosterone
- Biosynthetic Link : 28-Homodolichosterone is a precursor to this compound in Arabidopsis, converted via light-regulated pathways involving DWARF1 .
- Bioactivity : this compound exhibits higher root growth inhibition activity, suggesting it is the more bioactive end product .
Data Tables
Table 1: Structural and Functional Comparison of Brassinosteroids
Table 2: Cytotoxicity and Stress Response Data
Key Research Findings
- Stress Adaptation: Cold hardening (5°C) increases this compound levels in barley by 3-fold, while heat (27°C) reduces it . this compound application mitigates copper toxicity in Brassica juncea by enhancing antioxidant enzymes (e.g., SOD, CAT) and polyphenol metabolism .
- Cancer Research :
- Membrane Interactions :
- This compound integrates into lipid bilayers more efficiently than homothis compound, altering membrane fluidity and ion adsorption .
Biologische Aktivität
Castasterone (CAS) is a significant plant steroid hormone belonging to the brassinosteroid (BR) family, which plays crucial roles in plant growth, development, and stress responses. This article explores the biological activity of this compound, focusing on its mechanisms of action, effects on various plant species, and potential therapeutic applications in human health, particularly in cancer treatment.
Overview of this compound
First isolated from insect galls on chestnut trees in 1982, this compound is recognized for its potent biological activities. It serves as a precursor to epibrassinolide (EB), another active BR, and is involved in various physiological processes such as cell elongation, division, and differentiation. This compound is present in many plant species and contributes to their adaptation to environmental stresses.
1. Cytotoxic Effects in Cancer Cells
Recent studies have demonstrated that this compound exhibits cytotoxic effects on small-cell lung cancer (SCLC) cells. Research indicated that this compound has an IC50 value of approximately 1.0 μM for both drug-sensitive (H69) and multi-drug resistant (VPA17) SCLC cell lines. Notably, this compound did not exhibit cytotoxicity on normal lung epithelial cells, suggesting a tumor-specific action .
- Table 1: Cytotoxicity of this compound on SCLC Cells
Cell Line | IC50 (μM) | Cytotoxicity on Normal Cells |
---|---|---|
H69 | 1.0 | No effect (IC50 > 40 μM) |
VPA17 | 1.0 | No effect (IC50 > 40 μM) |
The mechanism behind its cytotoxicity may involve the induction of apoptosis through the activation of the Wnt signaling pathway, evidenced by a significant reduction in β-catenin levels following treatment with this compound .
2. Enhancement of Plant Stress Tolerance
This compound has been shown to enhance tolerance to abiotic stresses such as salinity and heavy metal toxicity. In studies involving Brassica juncea, this compound application improved the plant's antioxidant defense system under copper stress by increasing the activities of superoxide dismutase (SOD), catalase (CAT), and polyphenol oxidase (PPO) .
- Table 2: Antioxidative Enzyme Activities in Brassica juncea
Treatment | SOD Activity (%) | CAT Activity (%) | PPO Activity (%) |
---|---|---|---|
Control | Baseline | Baseline | Baseline |
Copper Stress | Increased | Increased | Increased |
This compound + Cu | Significantly Increased | Significantly Increased | Significantly Increased |
The study highlighted that this compound not only mitigated oxidative stress but also promoted the accumulation of phenolic compounds such as caffeic acid and chlorogenic acid, which are vital for plant defense mechanisms .
Case Study 1: this compound in Cancer Therapy
A study evaluated the effects of this compound on SCLC cells, revealing its potential as a therapeutic agent. The findings showed that pretreatment with this compound restored drug sensitivity in resistant cell lines and demonstrated synergistic effects when combined with conventional chemotherapy agents like etoposide and doxorubicin .
- Table 3: Synergistic Effects of this compound with Chemotherapy
Drug Combination | Effect on Drug Sensitivity |
---|---|
This compound + Etoposide | Restored sensitivity |
This compound + Doxorubicin | Restored sensitivity |
This suggests that this compound may play a role in overcoming multi-drug resistance in cancer therapy.
Case Study 2: Enhancing Crop Resilience
In agricultural research, this compound has been applied to enhance crop resilience against environmental stresses. For instance, lettuce primed with this compound showed improved resistance to salt stress by modulating phenolic content and antioxidant capacity .
Q & A
Q. What experimental approaches are used to elucidate the biosynthetic pathways of castasterone in plants?
Basic Research Question
this compound biosynthesis involves both campestanol-dependent and campestanol-independent pathways. Key methodologies include:
- GC-MS analysis to identify intermediates like 6-deoxothis compound and teasterone in plant tissues .
- In vitro enzyme assays with crude plant extracts to trace sequential conversions (e.g., campesterol → campesta-3-one → 6-deoxocathasterone) .
- Mutant studies (e.g., Arabidopsis CYP85A2 knockout) to confirm the absence of brassinolide and validate this compound as the terminal bioactive BR .
Q. How can researchers accurately quantify this compound and its isomers in plant tissues?
Basic Research Question
Quantification requires:
- Deuterated internal standards (e.g., [²H₆]-castasterone) for precise calibration in GC-MS or LC-MS .
- High-resolution chromatography to separate stereoisomers (e.g., 3-epithis compound vs. This compound) using dansyl-aminophenylboronate derivatives .
- Validation via SIM-MS (Selected Ion Monitoring) to detect molecular ions (e.g., m/z 805 for this compound) and avoid co-elution artifacts .
Q. What methodological strategies are employed to study this compound’s role in plant growth regulation?
Basic Research Question
- Hormonal profiling in BR-deficient mutants (e.g., barley 522DK) to correlate this compound levels with phenotypes like dwarfism .
- Exogenous application assays (e.g., root elongation tests) using synthetic this compound to assess dose-dependent growth responses .
- Transcriptional analysis of BR-responsive genes (e.g., DWF4, CPD) to map signaling pathways .
Q. How does light regulate this compound biosynthesis, and what experimental designs are used to investigate this?
Advanced Research Question
Light modulates BR activity via:
- Gene expression studies (e.g., qRT-PCR of DWF1 in Arabidopsis) to show upregulation under light, enhancing 28-homodolichosterone → this compound conversion .
- Enzyme kinetics assays under varying light conditions to measure substrate turnover rates .
- Mutant complementation (e.g., 35S-DWF1 overexpression) to confirm light-dependent pathway activation .
Q. What experimental challenges arise when analyzing this compound’s metabolic interconversion with brassinolide?
Advanced Research Question
Key challenges include:
- Isotopic tracer studies using [²⁶,²⁸-²H₆]-castasterone to track brassinolide synthesis in Arabidopsis and tomato, requiring stringent MS validation to avoid false positives .
- Enzyme specificity assays (e.g., recombinant CYP734A7) to distinguish this compound hydroxylation from epimerization .
- Competitive inhibition tests to resolve overlapping substrate preferences (e.g., brassinolide vs. This compound binding to CYP734A7) .
Q. How do BR-biosynthetic mutants inform the study of this compound’s cross-talk with other phytohormones?
Advanced Research Question
- Multi-hormonal profiling in mutants (e.g., barley HvCPD) under stress to quantify interactions between this compound, ABA, and salicylic acid .
- Time-course experiments to assess how this compound depletion alters auxin transport or ethylene biosynthesis .
- Transcriptome co-expression networks to identify shared regulatory nodes (e.g., BZR1 transcription factors) .
Q. What methodologies are used to investigate this compound’s role in abiotic stress tolerance?
Advanced Research Question
- Antioxidant enzyme assays (e.g., SOD, CAT) in Brassica juncea exposed to copper stress, with this compound-treated vs. control groups .
- Drought stress experiments measuring homothis compound/castasterone ratios in barley DH lines via HPLC .
- Metabolomic profiling to link this compound-induced polyphenol accumulation with ROS scavenging .
Q. How can researchers resolve contradictions in this compound accumulation data across genotypes or environmental conditions?
Advanced Research Question
- Genotype-specific enzyme activity assays (e.g., CYP90D2 in wheat calli) to explain divergent homo-/castasterone ratios .
- Environmental perturbation studies (e.g., temperature acclimation) with hormonal time-series sampling .
- Statistical frameworks (ANOVA with Tukey’s HSD) to validate differences in BR levels under variable growth conditions .
Q. What genetic engineering approaches are used to modulate this compound levels for functional studies?
Advanced Research Question
- Overexpression of CYP85A1 in tomato to enhance 6-deoxothis compound → this compound conversion, validated via PCR and hormonal profiling .
- CRISPR/Cas9 knockout of CYP734A7 to reduce this compound catabolism and study its accumulation .
- Heterologous expression of BdDET2 or BdCYP90B1 in Brachypodium to reconstitute BR pathways .
Q. How do researchers design experiments to study temperature-dependent this compound signaling?
Advanced Research Question
- Controlled environment trials with acclimation phases (e.g., 5°C vs. 27°C) and hormonal extraction at multiple timepoints .
- Thermal stability assays of BR enzymes (e.g., DET2) to assess activity loss under heat stress .
- Cross-species comparisons (e.g., Arabidopsis vs. barley) to identify conserved or divergent regulatory mechanisms .
Eigenschaften
IUPAC Name |
(2R,3S,5S,8S,9S,10R,13S,14S,17R)-17-[(2S,3R,4R,5R)-3,4-dihydroxy-5,6-dimethylheptan-2-yl]-2,3-dihydroxy-10,13-dimethyl-1,2,3,4,5,7,8,9,11,12,14,15,16,17-tetradecahydrocyclopenta[a]phenanthren-6-one | |
---|---|---|
Source | PubChem | |
URL | https://pubchem.ncbi.nlm.nih.gov | |
Description | Data deposited in or computed by PubChem | |
InChI |
InChI=1S/C28H48O5/c1-14(2)15(3)25(32)26(33)16(4)18-7-8-19-17-11-22(29)21-12-23(30)24(31)13-28(21,6)20(17)9-10-27(18,19)5/h14-21,23-26,30-33H,7-13H2,1-6H3/t15-,16+,17+,18-,19+,20+,21-,23+,24-,25-,26-,27-,28-/m1/s1 | |
Source | PubChem | |
URL | https://pubchem.ncbi.nlm.nih.gov | |
Description | Data deposited in or computed by PubChem | |
InChI Key |
VYUIKSFYFRVQLF-QONPFPPSSA-N | |
Source | PubChem | |
URL | https://pubchem.ncbi.nlm.nih.gov | |
Description | Data deposited in or computed by PubChem | |
Canonical SMILES |
CC(C)C(C)C(C(C(C)C1CCC2C1(CCC3C2CC(=O)C4C3(CC(C(C4)O)O)C)C)O)O | |
Source | PubChem | |
URL | https://pubchem.ncbi.nlm.nih.gov | |
Description | Data deposited in or computed by PubChem | |
Isomeric SMILES |
C[C@@H]([C@H]1CC[C@@H]2[C@@]1(CC[C@H]3[C@H]2CC(=O)[C@@H]4[C@@]3(C[C@H]([C@H](C4)O)O)C)C)[C@H]([C@@H]([C@H](C)C(C)C)O)O | |
Source | PubChem | |
URL | https://pubchem.ncbi.nlm.nih.gov | |
Description | Data deposited in or computed by PubChem | |
Molecular Formula |
C28H48O5 | |
Source | PubChem | |
URL | https://pubchem.ncbi.nlm.nih.gov | |
Description | Data deposited in or computed by PubChem | |
Molecular Weight |
464.7 g/mol | |
Source | PubChem | |
URL | https://pubchem.ncbi.nlm.nih.gov | |
Description | Data deposited in or computed by PubChem | |
CAS No. |
80736-41-0 | |
Record name | Castasterone | |
Source | Human Metabolome Database (HMDB) | |
URL | http://www.hmdb.ca/metabolites/HMDB0301789 | |
Description | The Human Metabolome Database (HMDB) is a freely available electronic database containing detailed information about small molecule metabolites found in the human body. | |
Explanation | HMDB is offered to the public as a freely available resource. Use and re-distribution of the data, in whole or in part, for commercial purposes requires explicit permission of the authors and explicit acknowledgment of the source material (HMDB) and the original publication (see the HMDB citing page). We ask that users who download significant portions of the database cite the HMDB paper in any resulting publications. | |
Retrosynthesis Analysis
AI-Powered Synthesis Planning: Our tool employs the Template_relevance Pistachio, Template_relevance Bkms_metabolic, Template_relevance Pistachio_ringbreaker, Template_relevance Reaxys, Template_relevance Reaxys_biocatalysis model, leveraging a vast database of chemical reactions to predict feasible synthetic routes.
One-Step Synthesis Focus: Specifically designed for one-step synthesis, it provides concise and direct routes for your target compounds, streamlining the synthesis process.
Accurate Predictions: Utilizing the extensive PISTACHIO, BKMS_METABOLIC, PISTACHIO_RINGBREAKER, REAXYS, REAXYS_BIOCATALYSIS database, our tool offers high-accuracy predictions, reflecting the latest in chemical research and data.
Strategy Settings
Precursor scoring | Relevance Heuristic |
---|---|
Min. plausibility | 0.01 |
Model | Template_relevance |
Template Set | Pistachio/Bkms_metabolic/Pistachio_ringbreaker/Reaxys/Reaxys_biocatalysis |
Top-N result to add to graph | 6 |
Feasible Synthetic Routes
Haftungsausschluss und Informationen zu In-Vitro-Forschungsprodukten
Bitte beachten Sie, dass alle Artikel und Produktinformationen, die auf BenchChem präsentiert werden, ausschließlich zu Informationszwecken bestimmt sind. Die auf BenchChem zum Kauf angebotenen Produkte sind speziell für In-vitro-Studien konzipiert, die außerhalb lebender Organismen durchgeführt werden. In-vitro-Studien, abgeleitet von dem lateinischen Begriff "in Glas", beinhalten Experimente, die in kontrollierten Laborumgebungen unter Verwendung von Zellen oder Geweben durchgeführt werden. Es ist wichtig zu beachten, dass diese Produkte nicht als Arzneimittel oder Medikamente eingestuft sind und keine Zulassung der FDA für die Vorbeugung, Behandlung oder Heilung von medizinischen Zuständen, Beschwerden oder Krankheiten erhalten haben. Wir müssen betonen, dass jede Form der körperlichen Einführung dieser Produkte in Menschen oder Tiere gesetzlich strikt untersagt ist. Es ist unerlässlich, sich an diese Richtlinien zu halten, um die Einhaltung rechtlicher und ethischer Standards in Forschung und Experiment zu gewährleisten.