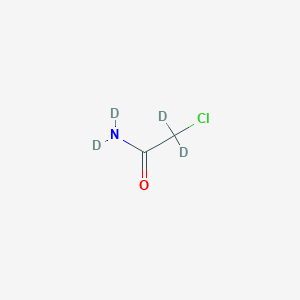
2-chloro-N,N,2,2-tetradeuterioacetamide
Übersicht
Beschreibung
“2-chloro-N,N,2,2-tetradeuterioacetamide” is a chemical compound with the molecular formula C2H4ClNO. It is a synthetic amide .
Synthesis Analysis
While specific synthesis methods for “2-chloro-N,N,2,2-tetradeuterioacetamide” were not found, a related compound, N-(substituted phenyl)-2-chloroacetamides, has been synthesized and studied . The synthesis of these compounds involved the use of an alkylating reagent .
Molecular Structure Analysis
The molecular structure of “2-chloro-N,N,2,2-tetradeuterioacetamide” consists of two carbon atoms, four hydrogen atoms, one chlorine atom, one nitrogen atom, and one oxygen atom.
Physical And Chemical Properties Analysis
The molecular weight of “2-chloro-N,N,2,2-tetradeuterioacetamide” is 97.54 g/mol. Other physical and chemical properties specific to this compound were not found.
Wissenschaftliche Forschungsanwendungen
Biomedical Applications
2-Chloroacetamide-d4: is utilized in the biomedical field due to its properties as an amide compound. It’s used in the synthesis of polyacrylamide, which is a material commonly employed in biomedical applications . The compound’s stability and reactivity make it suitable for creating hydrogels that can be used in drug delivery systems and tissue engineering.
Drug Delivery Systems
In drug delivery, 2-Chloroacetamide-d4 plays a crucial role in the development of controlled release formulations . Its chemical structure allows for the creation of polymer networks that can encapsulate drugs and release them at a controlled rate, which is essential for maintaining therapeutic drug levels in the body.
Wastewater Treatment
The compound is also applied in wastewater treatment processes. It acts as a precursor in the formation of polymers that are used as flocculants. These polymers help in the aggregation of contaminants, making them easier to remove from wastewater .
Heavy Metal Ion Removal
2-Chloroacetamide-d4: is involved in the synthesis of chelating agents that are effective in heavy metal ion removal from water bodies. This application is particularly important in the treatment of industrial effluents where heavy metal contamination is a significant concern .
Thermal Property Modification
Research has shown that 2-Chloroacetamide-d4 can be treated to modify its thermal properties. This includes changes in melting temperature and thermal decomposition temperature, which can be beneficial in various industrial applications where thermal stability is required .
Surface Area Analysis
The surface area of 2-Chloroacetamide-d4 can be altered through specific treatments, which is a valuable characteristic in catalysis and adsorption processes. An increased surface area can enhance the reactivity of a catalyst or the efficiency of an adsorbent .
Antimicrobial Applications
Derivatives of 2-Chloroacetamide-d4 have been studied for their antimicrobial properties. These compounds can be synthesized to combat drug-resistant pathogens, providing a new avenue for the development of antimicrobial agents .
Anticancer Research
Additionally, 2-Chloroacetamide-d4 derivatives are being explored for their potential in anticancer drug development. The ability to synthesize compounds that can target cancerous cells while avoiding drug resistance is a promising area of research .
Wirkmechanismus
Target of Action
The primary target of 2-Chloroacetamide-d4, also known as 2-chloro-N,N,2,2-tetradeuterioacetamide, is the enzyme responsible for the elongation of very-long-chain fatty acids (VLCFAs) in plants . VLCFAs play a crucial role in various biological processes, including membrane integrity, signaling, and energy storage .
Mode of Action
2-Chloroacetamide-d4 inhibits the VLCFA elongase, thereby disrupting the biosynthesis of VLCFAs . This inhibition is believed to occur through the compound’s ability to bind covalently to enzymes, coenzymes, or metabolic intermediates containing sulfhydryl (-SH) groups .
Biochemical Pathways
The inhibition of VLCFA elongase by 2-Chloroacetamide-d4 affects the biosynthesis of VLCFAs, leading to a disruption in the normal functioning of the plant cells . This disruption can affect various downstream processes, including lipid biosynthesis and other metabolic processes requiring coenzyme A .
Pharmacokinetics
Deuterium, a stable isotope of hydrogen, has been incorporated into drug molecules as tracers for quantitation during the drug development process .
Result of Action
The inhibition of VLCFA elongase by 2-Chloroacetamide-d4 leads to a disruption in the biosynthesis of VLCFAs, which can result in the inhibition of early plant development . This makes 2-Chloroacetamide-d4 effective as a herbicide in both uplands and paddy fields .
Action Environment
The action, efficacy, and stability of 2-Chloroacetamide-d4 can be influenced by various environmental factors. It’s important to note that 2-Chloroacetamide-d4 is used in various environments, including agriculture, glues, paints, and coatings .
Safety and Hazards
While specific safety and hazard information for “2-chloro-N,N,2,2-tetradeuterioacetamide” was not found, a related compound, 2-Chloro-N,N-diethylethylamine hydrochloride, has been studied. It is considered hazardous and can cause skin and eye irritation, respiratory irritation, and genetic defects .
Eigenschaften
IUPAC Name |
2-chloro-N,N,2,2-tetradeuterioacetamide | |
---|---|---|
Source | PubChem | |
URL | https://pubchem.ncbi.nlm.nih.gov | |
Description | Data deposited in or computed by PubChem | |
InChI |
InChI=1S/C2H4ClNO/c3-1-2(4)5/h1H2,(H2,4,5)/i1D2/hD2 | |
Source | PubChem | |
URL | https://pubchem.ncbi.nlm.nih.gov | |
Description | Data deposited in or computed by PubChem | |
InChI Key |
VXIVSQZSERGHQP-BGOGGDMHSA-N | |
Source | PubChem | |
URL | https://pubchem.ncbi.nlm.nih.gov | |
Description | Data deposited in or computed by PubChem | |
Canonical SMILES |
C(C(=O)N)Cl | |
Source | PubChem | |
URL | https://pubchem.ncbi.nlm.nih.gov | |
Description | Data deposited in or computed by PubChem | |
Isomeric SMILES |
[2H]C([2H])(C(=O)N([2H])[2H])Cl | |
Source | PubChem | |
URL | https://pubchem.ncbi.nlm.nih.gov | |
Description | Data deposited in or computed by PubChem | |
Molecular Formula |
C2H4ClNO | |
Source | PubChem | |
URL | https://pubchem.ncbi.nlm.nih.gov | |
Description | Data deposited in or computed by PubChem | |
DSSTOX Substance ID |
DTXSID90481541 | |
Record name | Microcide-d4 | |
Source | EPA DSSTox | |
URL | https://comptox.epa.gov/dashboard/DTXSID90481541 | |
Description | DSSTox provides a high quality public chemistry resource for supporting improved predictive toxicology. | |
Molecular Weight |
97.54 g/mol | |
Source | PubChem | |
URL | https://pubchem.ncbi.nlm.nih.gov | |
Description | Data deposited in or computed by PubChem | |
Product Name |
2-Chloroacetamide-d4 | |
CAS RN |
122775-20-6 | |
Record name | Acetamide-N,N,2,2-d4, 2-chloro- | |
Source | CAS Common Chemistry | |
URL | https://commonchemistry.cas.org/detail?cas_rn=122775-20-6 | |
Description | CAS Common Chemistry is an open community resource for accessing chemical information. Nearly 500,000 chemical substances from CAS REGISTRY cover areas of community interest, including common and frequently regulated chemicals, and those relevant to high school and undergraduate chemistry classes. This chemical information, curated by our expert scientists, is provided in alignment with our mission as a division of the American Chemical Society. | |
Explanation | The data from CAS Common Chemistry is provided under a CC-BY-NC 4.0 license, unless otherwise stated. | |
Record name | Microcide-d4 | |
Source | EPA DSSTox | |
URL | https://comptox.epa.gov/dashboard/DTXSID90481541 | |
Description | DSSTox provides a high quality public chemistry resource for supporting improved predictive toxicology. | |
Haftungsausschluss und Informationen zu In-Vitro-Forschungsprodukten
Bitte beachten Sie, dass alle Artikel und Produktinformationen, die auf BenchChem präsentiert werden, ausschließlich zu Informationszwecken bestimmt sind. Die auf BenchChem zum Kauf angebotenen Produkte sind speziell für In-vitro-Studien konzipiert, die außerhalb lebender Organismen durchgeführt werden. In-vitro-Studien, abgeleitet von dem lateinischen Begriff "in Glas", beinhalten Experimente, die in kontrollierten Laborumgebungen unter Verwendung von Zellen oder Geweben durchgeführt werden. Es ist wichtig zu beachten, dass diese Produkte nicht als Arzneimittel oder Medikamente eingestuft sind und keine Zulassung der FDA für die Vorbeugung, Behandlung oder Heilung von medizinischen Zuständen, Beschwerden oder Krankheiten erhalten haben. Wir müssen betonen, dass jede Form der körperlichen Einführung dieser Produkte in Menschen oder Tiere gesetzlich strikt untersagt ist. Es ist unerlässlich, sich an diese Richtlinien zu halten, um die Einhaltung rechtlicher und ethischer Standards in Forschung und Experiment zu gewährleisten.