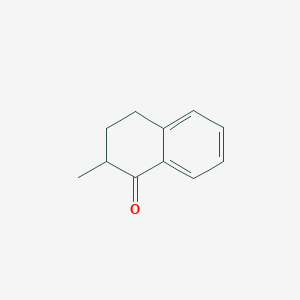
2-Methyl-1-Tetralon
Übersicht
Beschreibung
2-Methyl-1-tetralone is a member of the class of tetralins, which are bicyclic aromatic hydrocarbons. It is characterized by the presence of a methyl group at the second position and a ketone group at the first position of the tetralin structure.
Wissenschaftliche Forschungsanwendungen
Synthesis and Reactivity
2-Methyl-1-tetralone can be synthesized through the methylation of 1-tetralone followed by dehydrogenation using a palladium on carbon (Pd/C) catalyst . Its reactivity includes undergoing enantioselective hydrogenation catalyzed by 1,4-diamine-ruthenium(II) complexes, which is crucial for producing various derivatives .
Chemistry
2-Methyl-1-tetralone serves as a precursor for synthesizing various organic compounds, including pharmaceuticals and agrochemicals. It is particularly important in the production of fine chemicals and complex molecules .
Application Area | Details |
---|---|
Organic Synthesis | Starting material for pharmaceuticals and agrochemicals. |
Fine Chemicals | Used in the production of complex organic molecules. |
Biology
In biological research, this compound has been identified as a biochemical reagent. It has been shown to interact with the SARS-CoV-2 main protease (Mpro), where it forms a covalent bond that could inhibit viral replication . This interaction highlights its potential role in drug development against viral infections.
Biological Interaction | Mechanism |
---|---|
SARS-CoV-2 Mpro | Forms a covalent bond through Michael addition, potentially inhibiting virus replication. |
Medicine
2-Methyl-1-tetralone is utilized as an intermediate in drug synthesis, particularly for compounds exhibiting anti-inflammatory, antirheumatic, and antidiabetic properties. Its derivatives have been studied for their pharmacological effects and therapeutic potentials .
Case Studies
- Enantioselective Hydrogenation : Research demonstrated that 2-methyl-1-tetralone undergoes enantioselective hydrogenation to yield specific chiral products. This reaction is significant for synthesizing drugs that require precise stereochemistry .
- SARS-CoV-2 Protease Binding : A study revealed that the compound binds covalently to the catalytic site of Mpro, providing insights into its potential as a therapeutic agent against COVID-19 . The study utilized X-ray crystallography to elucidate the binding mechanism.
- Oxidation Studies : Investigations into the oxidation of 2-methyl-1-tetralone indicated its susceptibility to atmospheric oxygen, leading to the formation of hydroperoxides. This property is critical for understanding its stability and reactivity under various conditions .
Wirkmechanismus
Target of Action
2-Methyl-1-tetralone is a biochemical reagent that can be used as a biological material or organic compound for life science related research . It has been found to interact with the SARS-CoV-2 main protease (Mpro), a key enzyme in the replication of the SARS-CoV-2 virus .
Mode of Action
The compound has been observed to undergo a catalytic cleavage when interacting with the SARS-CoV-2 main protease (Mpro). This cleavage results in the formation of 2-methylene-1-tetralone and tyramine. The catalytic Cys145 subsequently binds covalently in a Michael addition to the methylene carbon atom of 2-methylene-1-tetralone .
Biochemical Pathways
The compound is involved in the enzymatic cofactorless addition of O2 to an organic substrate, a process that promotes charge-transfer between the two partners, inducing intersystem crossing between the triplet and singlet states involved in the process .
Pharmacokinetics
Information on the pharmacokinetics of 2-Methyl-1-tetralone is currently limited. It’s known that the compound undergoes enantioselective hydrogenation catalyzed by 1,4-diamine-ruthenium (ii) complexes .
Result of Action
The result of the action of 2-Methyl-1-tetralone is the formation of a covalent bond with the SARS-CoV-2 main protease (Mpro), which could potentially inhibit the replication of the virus .
Action Environment
The action of 2-Methyl-1-tetralone can be influenced by the local polarity in the environment. For instance, in imidazol-based ionic liquids (ILs), the local polarity represented by the ET(30) value or the chemical shift of α-proton at the substrate was found to be a critical factor influencing the reaction rate .
Biochemische Analyse
Biochemical Properties
2-Methyl-1-tetralone has been found to undergo enantioselective hydrogenation catalyzed by 1,4-diamine-ruthenium (II) complexes This suggests that it interacts with these complexes in a biochemical context
Molecular Mechanism
The molecular mechanism of 2-Methyl-1-tetralone involves its enantioselective hydrogenation catalyzed by 1,4-diamine-ruthenium (II) complexes This suggests that it may exert its effects at the molecular level through binding interactions with these complexes
Vorbereitungsmethoden
Synthetic Routes and Reaction Conditions: The preparation of 2-Methyl-1-tetralone can be achieved through several synthetic routes. One common method involves the methylation of 1-tetralone followed by subsequent dehydrogenation. This process typically uses a palladium on carbon (Pd/C) catalyst . Another method involves a one-pot reaction where 1-tetralone undergoes hydrogen drawing and methylation using methyl trifluoroacetate, methyl p-benzenesulfonate, or methyl bromide. The product is then collected through reduced pressure distillation .
Industrial Production Methods: In industrial settings, the production of 2-Methyl-1-tetralone often involves the use of continuous-flow synthesis techniques. These methods allow for better control over reaction conditions and higher yields. For instance, the flow reduction process can be carried out in a stainless steel coil reactor at elevated temperatures and pressures .
Analyse Chemischer Reaktionen
Types of Reactions: 2-Methyl-1-tetralone undergoes various chemical reactions, including:
Oxidation: The compound can be oxidized to form hydroperoxides and other oxygenated derivatives.
Substitution: The compound can undergo substitution reactions, particularly at the methyl group, to form various derivatives.
Common Reagents and Conditions:
Oxidation: Common oxidizing agents include atmospheric oxygen and hydrogen peroxide.
Reduction: Catalysts such as palladium on carbon (Pd/C) and 1,4-diamine-ruthenium (II) complexes are frequently used.
Substitution: Reagents like methyl trifluoroacetate and methyl bromide are used for methylation reactions.
Major Products:
Oxidation: Hydroperoxides and hydroxylated derivatives.
Reduction: Enantioselective hydrogenation products.
Substitution: Various methylated and substituted derivatives.
Vergleich Mit ähnlichen Verbindungen
1-Tetralone: Similar in structure but lacks the methyl group at the second position.
6-Methoxy-1-tetralone: Contains a methoxy group at the sixth position instead of a methyl group.
1-Benzosuberone: A related compound with a different ring structure.
Uniqueness: 2-Methyl-1-tetralone is unique due to its specific substitution pattern, which imparts distinct chemical reactivity and biological activity. Its methyl group at the second position and ketone group at the first position make it a versatile intermediate in organic synthesis.
Biologische Aktivität
2-Methyl-1-tetralone is a compound that has garnered interest in various fields due to its diverse biological activities. This article explores its pharmacological properties, synthesis, and potential applications, supported by research findings and data tables.
Chemical Structure and Synthesis
2-Methyl-1-tetralone is a member of the tetralone family, characterized by a bicyclic structure containing a ketone functional group. It can be synthesized through several methods, including the Mannich reaction involving 1-tetralone and formaldehyde in the presence of secondary amines . The synthesis process is of particular interest due to its efficiency and the potential for producing derivatives with enhanced biological activity.
Antimicrobial and Antifungal Properties
Research indicates that tetralone derivatives, including 2-methyl-1-tetralone, exhibit significant antimicrobial and antifungal activities. For instance, studies have shown that certain tetralones can inhibit the growth of various pathogens. The mechanism of action often involves interaction with microbial enzymes or cell membranes, leading to cell death or inhibition of growth .
Table 1: Antimicrobial Activity of Tetralones
Compound | Microorganism | Activity (IC50) |
---|---|---|
2-Methyl-1-tetralone | Staphylococcus aureus | 5 ppm |
2-Methyl-1-tetralone | Escherichia coli | Not active |
Scytalone | MRSA | Not active |
Anticancer Potential
The anticancer properties of 2-methyl-1-tetralone have also been explored. A study demonstrated that this compound could inhibit the proliferation of KB cell lines, with IC50 values ranging from 1 to 5 ppm . The underlying mechanisms are thought to involve apoptosis induction and cell cycle arrest, although specific pathways are still under investigation.
Case Study: Inhibition of Cancer Cell Lines
A notable case study involved treating human cancer cell lines with varying concentrations of 2-methyl-1-tetralone. Results indicated a dose-dependent response in cell viability, suggesting potential for further development as an anticancer agent.
The biological activity of 2-methyl-1-tetralone can be attributed to its ability to interact with various biological targets. For instance, it has been noted for its inhibitory effects on cytochrome P450 enzymes, which play a crucial role in drug metabolism and synthesis of steroid hormones . The selectivity towards specific isoforms (e.g., CYP24A1) has implications for its use in treating conditions related to steroid metabolism.
Table 2: Enzyme Inhibition by Tetralones
Compound | Enzyme | IC50 |
---|---|---|
2-Methyl-1-tetralone | CYP24A1 | 16.3 μM |
Compound A | MAO-B | <7.8 μM |
Future Directions and Applications
Given its promising biological activities, further research into the pharmacological applications of 2-methyl-1-tetralone is warranted. Potential areas include:
- Pharmaceutical Development : Exploring formulations that leverage its antimicrobial and anticancer properties.
- Agricultural Use : Investigating its efficacy as a plant growth regulator or pesticide analog.
- Synthetic Chemistry : Developing more efficient synthetic routes for producing analogs with enhanced activity.
Eigenschaften
IUPAC Name |
2-methyl-3,4-dihydro-2H-naphthalen-1-one | |
---|---|---|
Source | PubChem | |
URL | https://pubchem.ncbi.nlm.nih.gov | |
Description | Data deposited in or computed by PubChem | |
InChI |
InChI=1S/C11H12O/c1-8-6-7-9-4-2-3-5-10(9)11(8)12/h2-5,8H,6-7H2,1H3 | |
Source | PubChem | |
URL | https://pubchem.ncbi.nlm.nih.gov | |
Description | Data deposited in or computed by PubChem | |
InChI Key |
GANIBVZSZGNMNB-UHFFFAOYSA-N | |
Source | PubChem | |
URL | https://pubchem.ncbi.nlm.nih.gov | |
Description | Data deposited in or computed by PubChem | |
Canonical SMILES |
CC1CCC2=CC=CC=C2C1=O | |
Source | PubChem | |
URL | https://pubchem.ncbi.nlm.nih.gov | |
Description | Data deposited in or computed by PubChem | |
Molecular Formula |
C11H12O | |
Source | PubChem | |
URL | https://pubchem.ncbi.nlm.nih.gov | |
Description | Data deposited in or computed by PubChem | |
DSSTOX Substance ID |
DTXSID00936032 | |
Record name | 2-Methyl-3,4-dihydronaphthalen-1(2H)-one | |
Source | EPA DSSTox | |
URL | https://comptox.epa.gov/dashboard/DTXSID00936032 | |
Description | DSSTox provides a high quality public chemistry resource for supporting improved predictive toxicology. | |
Molecular Weight |
160.21 g/mol | |
Source | PubChem | |
URL | https://pubchem.ncbi.nlm.nih.gov | |
Description | Data deposited in or computed by PubChem | |
CAS No. |
1590-08-5 | |
Record name | 2-Methyl-1-tetralone | |
Source | CAS Common Chemistry | |
URL | https://commonchemistry.cas.org/detail?cas_rn=1590-08-5 | |
Description | CAS Common Chemistry is an open community resource for accessing chemical information. Nearly 500,000 chemical substances from CAS REGISTRY cover areas of community interest, including common and frequently regulated chemicals, and those relevant to high school and undergraduate chemistry classes. This chemical information, curated by our expert scientists, is provided in alignment with our mission as a division of the American Chemical Society. | |
Explanation | The data from CAS Common Chemistry is provided under a CC-BY-NC 4.0 license, unless otherwise stated. | |
Record name | 1,2,3,4-Tetrahydro-2-methylnaphthalen-1-one | |
Source | ChemIDplus | |
URL | https://pubchem.ncbi.nlm.nih.gov/substance/?source=chemidplus&sourceid=0001590085 | |
Description | ChemIDplus is a free, web search system that provides access to the structure and nomenclature authority files used for the identification of chemical substances cited in National Library of Medicine (NLM) databases, including the TOXNET system. | |
Record name | 2-Methyl-3,4-dihydronaphthalen-1(2H)-one | |
Source | EPA DSSTox | |
URL | https://comptox.epa.gov/dashboard/DTXSID00936032 | |
Description | DSSTox provides a high quality public chemistry resource for supporting improved predictive toxicology. | |
Record name | 1,2,3,4-tetrahydro-2-methylnaphthalen-1-one | |
Source | European Chemicals Agency (ECHA) | |
URL | https://echa.europa.eu/substance-information/-/substanceinfo/100.014.966 | |
Description | The European Chemicals Agency (ECHA) is an agency of the European Union which is the driving force among regulatory authorities in implementing the EU's groundbreaking chemicals legislation for the benefit of human health and the environment as well as for innovation and competitiveness. | |
Explanation | Use of the information, documents and data from the ECHA website is subject to the terms and conditions of this Legal Notice, and subject to other binding limitations provided for under applicable law, the information, documents and data made available on the ECHA website may be reproduced, distributed and/or used, totally or in part, for non-commercial purposes provided that ECHA is acknowledged as the source: "Source: European Chemicals Agency, http://echa.europa.eu/". Such acknowledgement must be included in each copy of the material. ECHA permits and encourages organisations and individuals to create links to the ECHA website under the following cumulative conditions: Links can only be made to webpages that provide a link to the Legal Notice page. | |
Retrosynthesis Analysis
AI-Powered Synthesis Planning: Our tool employs the Template_relevance Pistachio, Template_relevance Bkms_metabolic, Template_relevance Pistachio_ringbreaker, Template_relevance Reaxys, Template_relevance Reaxys_biocatalysis model, leveraging a vast database of chemical reactions to predict feasible synthetic routes.
One-Step Synthesis Focus: Specifically designed for one-step synthesis, it provides concise and direct routes for your target compounds, streamlining the synthesis process.
Accurate Predictions: Utilizing the extensive PISTACHIO, BKMS_METABOLIC, PISTACHIO_RINGBREAKER, REAXYS, REAXYS_BIOCATALYSIS database, our tool offers high-accuracy predictions, reflecting the latest in chemical research and data.
Strategy Settings
Precursor scoring | Relevance Heuristic |
---|---|
Min. plausibility | 0.01 |
Model | Template_relevance |
Template Set | Pistachio/Bkms_metabolic/Pistachio_ringbreaker/Reaxys/Reaxys_biocatalysis |
Top-N result to add to graph | 6 |
Feasible Synthetic Routes
Q1: What is the molecular formula and weight of 2-methyl-1-tetralone?
A1: 2-Methyl-1-tetralone has the molecular formula C11H12O and a molecular weight of 160.21 g/mol.
Q2: How is 2-methyl-1-tetralone used in studies of enantioselective protonation?
A2: 2-Methyl-1-tetralone serves as a model substrate for exploring enantioselective protonation reactions. Researchers utilize the achiral enolate of 2-methyl-1-tetralone to test the effectiveness of chiral proton sources in generating enantiomerically enriched (R)- or (S)-2-methyl-1-tetralone. [, , , , , ]
Q3: What chiral reagents have shown promise in achieving enantioselective protonation of 2-methyl-1-tetralone?
A3: Several chiral reagents have demonstrated potential in this area:
- TADDOLs: Specifically, (R,R)-TADDOLs bearing naphthalen-1-yl groups have yielded (S)-2-methyl-1-tetralone with impressive selectivity, reaching up to 99.5% e.e. []
- Chiral Sulfonamides: C2-symmetric tris-sulfonamides and bis-sulfonamides have successfully produced enantiomerically enriched (R)- and (S)-2-methyl-1-tetralone, respectively. [, , ]
- Chiral β-Hydroxyethers: These compounds, particularly those with 3,5-dichloro substitution, have shown good enantiocontrol, achieving up to 90% e.e. []
- Chiral Phenolic Additives: Oxazoline-based additives have facilitated the synthesis of (+)-(R)-2-methyl-1-tetralone with up to 29% e.e. []
- Amino Alcohols: These have been used in conjunction with Pd/C catalysts for the enantioselective decarboxylation of 2-carboxy-2-methyl-1-tetralone, yielding enantioenriched 2-methyl-1-tetralone. [, ]
Q4: How have computational methods been employed to study 2-methyl-1-tetralone?
A4: Quantum chemical calculations, particularly at the (U)B3LYP/6-31G(d) level of theory, have been instrumental in unraveling the oxidation mechanism of the enol form of 2-methyl-1-tetralone by triplet oxygen. [] Additionally, DFT calculations have been employed to investigate the conformational flexibility of 2-hydroxy-2-methyl-1-tetralone oxime. []
Q5: How do structural modifications of 2-methyl-1-tetralone derivatives impact their biological activity?
A5: Research on aminotetralone analogues, specifically 1-amino-1-methyl-2-tetralone and 2-amino-2-methyl-1-tetralone, reveals distinct effects on locomotor activity in mice. The 2-amino derivative increased activity, whereas the 1-amino derivative had a depressant effect. This highlights the significance of substituent position on the tetralone scaffold for biological activity. []
Q6: What is known about the stability of 2-methyl-1-tetralone under different conditions?
A6: While specific stability data for 2-methyl-1-tetralone might be limited in the provided research, one study [] highlights its susceptibility to oxidation in the presence of atmospheric oxygen. This finding suggests the need for storage under inert conditions. Additionally, the formation of 2-hydroperoxy-2-methyl-1-tetralone upon reaction with oxygen underscores the potential instability of its enol form.
Q7: What analytical techniques are commonly used to study reactions involving 2-methyl-1-tetralone?
A7: Various spectroscopic techniques, including NMR, UV, and IR spectroscopy, have been crucial for monitoring the kinetics of reactions involving 2-methyl-1-tetralone and its derivatives. [] X-ray crystallography has also been employed to determine the absolute configuration of reaction products, such as the diastereomeric β-hydroxy sulfoximine derived from 2-fluoro-2-methyl-1-tetralone. []
Q8: Are there publicly available resources for researchers studying 2-methyl-1-tetralone?
A8: Yes, raw diffraction data for the crystal structure of the SARS-CoV-2 main protease bound to 2-methyl-1-tetralone is available. [] This resource can aid in developing antiviral drugs targeting the SARS-CoV-2 main protease.
Q9: What are some historical milestones in the research on 2-methyl-1-tetralone?
A9: An early milestone was the determination of the absolute configuration of cis- and trans-2-methyl-1-tetralols and 2-methyl-1-tetralone. [] This fundamental work laid the groundwork for subsequent stereochemical studies involving these compounds.
Q10: How does the study of 2-methyl-1-tetralone bridge different scientific disciplines?
A10: The research on 2-methyl-1-tetralone exemplifies interdisciplinary synergy. For instance, the use of this compound in developing enantioselective catalytic processes combines aspects of organic chemistry, catalysis, and stereochemistry. [, , , , , , , , , ] Additionally, investigations into its interactions with the SARS-CoV-2 main protease highlight the intersection of synthetic chemistry, structural biology, and medicinal chemistry. [, ]
Haftungsausschluss und Informationen zu In-Vitro-Forschungsprodukten
Bitte beachten Sie, dass alle Artikel und Produktinformationen, die auf BenchChem präsentiert werden, ausschließlich zu Informationszwecken bestimmt sind. Die auf BenchChem zum Kauf angebotenen Produkte sind speziell für In-vitro-Studien konzipiert, die außerhalb lebender Organismen durchgeführt werden. In-vitro-Studien, abgeleitet von dem lateinischen Begriff "in Glas", beinhalten Experimente, die in kontrollierten Laborumgebungen unter Verwendung von Zellen oder Geweben durchgeführt werden. Es ist wichtig zu beachten, dass diese Produkte nicht als Arzneimittel oder Medikamente eingestuft sind und keine Zulassung der FDA für die Vorbeugung, Behandlung oder Heilung von medizinischen Zuständen, Beschwerden oder Krankheiten erhalten haben. Wir müssen betonen, dass jede Form der körperlichen Einführung dieser Produkte in Menschen oder Tiere gesetzlich strikt untersagt ist. Es ist unerlässlich, sich an diese Richtlinien zu halten, um die Einhaltung rechtlicher und ethischer Standards in Forschung und Experiment zu gewährleisten.