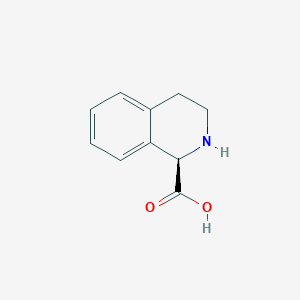
(R)-1,2,3,4-Tetrahydroisoquinoline-1-carboxylic acid
Übersicht
Beschreibung
(R)-1,2,3,4-Tetrahydroisoquinoline-1-carboxylic acid (abbreviated as (R)-THIQ-1-COOH) is a chiral tetrahydroisoquinoline (THIQ) derivative featuring a carboxylic acid group at the 1-position of the isoquinoline scaffold. This compound is synthesized via diastereoselective methods such as the Petasis reaction followed by Pomeranz–Fritsch–Bobbitt cyclization (Scheme 11, ). Key spectral data (1H/13C NMR, IR, MS) confirm its structural integrity, with optical rotation values ([α]D–) indicating its enantiomeric purity. (R)-THIQ-1-COOH is commercially available (CAS 151004-93-2) and serves as a critical intermediate in medicinal chemistry due to its constrained conformation, which mimics natural amino acids and enhances receptor binding.
Vorbereitungsmethoden
Enzymatic Kinetic Resolution via Immobilized Lipase Catalysis
A landmark approach involves the enzymatic resolution of racemic esters using immobilized lipase in a circulating fluidized bed reactor (CFBR). This method, disclosed in a 2022 patent application , employs racemic ethyl (R,S)-1,2,3,4-tetrahydroisoquinoline-1-carboxylate as the substrate. The lipase selectively hydrolyzes the (S)-enantiomer, leaving the (R)-ester intact, which is subsequently isolated and hydrolyzed to R-Tic.
Key Features:
-
Reactor Design : The CFBR system enhances enzyme-substrate contact through continuous circulation between an external reservoir and a reaction column packed with immobilized lipase (e.g., Candida antarctica Lipase B).
-
Stereoselectivity : The lipase exhibits >99% enantiomeric excess (e.e.) for the (S)-ester, ensuring high optical purity of the residual (R)-ester .
-
Efficiency Metrics :
-
Enzyme/substrate ratio: 1:50 (w/w)
-
Reaction time: 12–24 hours
-
Yield: 85–92% (R-Tic)
-
Optical purity: 98–99% e.e.
-
This method’s scalability and mild reaction conditions (30–40°C, pH 7.0–7.5) make it industrially viable. However, the requirement for specialized equipment (CFBR) and enzyme immobilization expertise limits its accessibility for small-scale laboratories.
Asymmetric Pictet-Spengler Cyclization
The Pictet-Spengler reaction offers a direct route to tetrahydroisoquinoline scaffolds. A diastereoselective variant reported by Thieme Connect utilizes hexafluoroacetone-protected L-phenylalanine and glyoxylic acid to form (1S,3S)-1,2,3,4-tetrahydroisoquinoline-1,3-dicarboxylic acid. Adapting this for R-Tic synthesis requires chiral inversion or alternate starting materials.
Reaction Optimization:
-
Diastereoselectivity : The hexafluoroacetone group induces a syn-periplanar transition state, favoring cis-diastereomers (d.r. >20:1) .
-
Modifications for R-Tic :
-
Substituting L-phenylalanine with D-phenylalanine reverses configuration at C1.
-
Post-cyclization decarboxylation selectively removes the C3 carboxyl group.
-
Data Table: Asymmetric Pictet-Spengler Parameters
Starting Material | Chiral Auxiliary | Temperature | Diastereomeric Ratio (d.r.) | Yield (%) |
---|---|---|---|---|
D-Phenylalanine | Hexafluoroacetone | 0°C | 18:1 (1R,3S) | 78 |
L-Phenylalanine | Hexafluoroacetone | 0°C | 22:1 (1S,3S) | 82 |
While this method achieves high stereocontrol, the need for expensive fluorinated solvents and multi-step purification complicates large-scale implementation.
Chiral Pool Synthesis from Amino Acid Precursors
Japanese Patent JPH09194463A outlines a chiral pool strategy starting from L-phenylalanine. The protocol involves:
-
Cyclization : L-Phenylalanine reacts with formaldehyde in concentrated HCl at 50°C for 24 hours, yielding L-Tic hydrochloride.
-
Neutralization : Treatment with ammonium hydroxide liberates free L-Tic (99% e.e.).
For R-Tic synthesis, substituting L-phenylalanine with D-phenylalanine generates the D-configuration. However, commercial scarcity of D-phenylalanine necessitates resolution steps, increasing costs.
Process Data:
-
Cyclization Conditions :
-
Solvent: 37% HCl
-
Temperature: 50°C
-
Time: 24 hours
-
-
Yield : 75% (hydrochloride salt), 95% after neutralization .
This method’s reliance on natural amino acids ensures stereochemical fidelity but faces economic barriers due to D-phenylalanine’s limited availability.
Chemoenzymatic Hybrid Approaches
Combining chemical synthesis with enzymatic resolution addresses limitations of standalone methods. A representative workflow involves:
-
Racemic Synthesis : Prepare (R,S)-Tic via Bischler-Napieralski cyclization of phenethylamine derivatives.
-
Enzymatic Resolution : Use lipase to hydrolyze the (S)-enantiomer ester, as described in .
Advantages:
-
Flexibility : Compatible with diverse starting materials (e.g., ketoamides, Grignard reagents) .
-
Cost-Effectiveness : Racemic precursors are synthetically accessible, reducing reliance on chiral auxiliaries.
Comparative Efficiency:
Method | Starting Material | Optical Purity (% e.e.) | Overall Yield (%) |
---|---|---|---|
Enzymatic Resolution | Racemic ester | 98–99 | 85–92 |
Chiral Pool | D-Phenylalanine | 99 | 70–75 |
Asymmetric Cyclization | D-Phenylalanine | 95 | 65–78 |
Industrial-Scale Considerations
Cost-Benefit Analysis
-
Enzymatic Methods : High upfront costs for immobilized enzymes and CFBR systems are offset by reusable catalysts (>10 cycles without activity loss) .
-
Chiral Pool Synthesis : Low chemical complexity but constrained by D-phenylalanine pricing (~$500/g vs. $5/g for L-isomer).
Environmental Impact
Analyse Chemischer Reaktionen
Petasis Reaction for Oxazinone Intermediate Formation
The Petasis multicomponent reaction is critical for constructing the stereochemical framework. This reaction combines three components:
Reaction Conditions :
-
Solvent: Dichloromethane (DCM)
-
Temperature: Room temperature
Stereochemical Outcome :
Pomeranz–Fritsch–Bobbitt Cyclization
This acid-catalyzed cyclization converts oxazinone intermediates into the tetrahydroisoquinoline core:
Key Steps :
-
Hydrogenolysis : Removal of the benzyl group from oxazinone 48 using catalytic hydrogenation ( ).
-
Cyclization : Treatment with HCl in ethanol/water induces ring closure ( ).
Outcome :
Transamination via D-Amino Acid Oxidase
-
Catalyst : D-Amino acid oxidase from Fusarium solani (FsDAAO) ( )
-
Substrate : Racemic 6,7-dimethoxy-1,2,3,4-tetrahydroisoquinoline-1-carboxylic acid
Grignard Addition–Alkylation Sequence
-
Substrates :
-
Steps :
Comparative Reaction Data
Critical Analysis of Stereochemical Control
-
(R)-Phenylglycinol serves as a chiral auxiliary in the Petasis reaction, directing trans-diastereoselectivity ( ).
-
Recrystallization of intermediates (e.g., 41 ) enhances enantiopurity to >90% e.e. ( ).
-
Competing pathways in cyclization are minimized by rigid oxazinone intermediates ( ).
Functionalization and Derivatives
While direct functionalization of the tetrahydroisoquinoline core is less documented, the carboxylic acid moiety enables:
Wissenschaftliche Forschungsanwendungen
Anticancer Activity
Recent studies have highlighted the potential of 1,2,3,4-tetrahydroisoquinoline-3-carboxylic acid derivatives as anti-cancer agents. For instance, a series of substituted derivatives were developed that demonstrated significant binding affinities to Bcl-2 and Mcl-1 proteins, which are crucial in regulating apoptosis in cancer cells. One compound from this series was shown to induce cell apoptosis and activate caspase-3 in Jurkat cells in a dose-dependent manner . This suggests that these derivatives could serve as promising candidates for new anticancer therapies.
Neurological Disorders
The compound has also been investigated for its potential role in treating neurological disorders. For example, certain tetrahydroisoquinoline derivatives are known to act as inhibitors of catechol-O-methyltransferase (COMT), which is involved in the metabolism of neurotransmitters like dopamine. This inhibition can enhance dopamine levels and is beneficial in conditions such as Parkinson's disease .
Chemoenzymatic Synthesis
The synthesis of enantiomerically pure 1,2,3,4-tetrahydroisoquinoline carboxylic acids has been achieved through chemoenzymatic methods using D-amino acid oxidase from Fusarium solani. This method allows for the efficient deracemization of racemic mixtures with high enantiomeric excess (up to 99%) and good yields (up to 82%) . Such methodologies are crucial for producing chiral compounds that are often required in pharmaceuticals.
Diversity-Oriented Synthesis
The diversity-oriented synthesis (DOS) approach has been applied to create a library of 1,2,3,4-tetrahydroisoquinoline-3-carboxylic acid derivatives. These derivatives serve as core structural elements in various biologically active compounds and peptide-based drugs. The synthesis employs various reactions such as the Pictet–Spengler reaction and Diels–Alder cycloaddition to generate a diverse range of analogs .
Case Studies
Wirkmechanismus
L-Ascorbic acid 2-phosphate (magnesium) exerts its effects through several mechanisms:
Antioxidant Properties: It acts as an antioxidant, scavenging free radicals and protecting cells from oxidative damage.
Cofactor for Enzymes: The compound serves as a cofactor for Fe(II) 2-oxoglutarate dioxygenase enzymes, promoting the activity of ten-eleven translocation (TET) enzymes involved in DNA demethylation.
Cellular Uptake: Its stability and slow hydrolysis allow for sustained release of ascorbic acid within cells, enhancing its biological effects.
Vergleich Mit ähnlichen Verbindungen
Comparison with Structural and Functional Analogs
Positional Isomers: 1-Carboxylic vs. 3-Carboxylic Acid Derivatives
The position of the carboxylic acid group on the THIQ scaffold significantly impacts biological activity and synthetic routes:
Key Differences :
- Stereochemical Impact : (R)-THIQ-1-COOH and its 3-carboxylic counterpart (Tic) exhibit divergent biological roles. For example, Tic derivatives show potent inhibition of anti-apoptotic proteins (Ki = 5.2 µM against Bcl-2), whereas (R)-THIQ-1-COOH is primarily utilized in synthetic routes for alkaloids.
- Synthesis: (R)-THIQ-1-COOH is synthesized via Petasis reaction and cyclization, while Tic derivatives often employ Castagnoli–Cushman reactions or Schotten-Baumann acylation.
Enantiomeric Comparisons
Enantiomeric purity is critical for biological activity. For example:
- (R)-THIQ-1-COOH : Obtained via enzymatic dynamic kinetic resolution (CAL-B catalysis) with 98% ee.
- (S)-THIQ-1-COOH : Less commonly reported, but accessible through subtilisin Carlsberg-catalyzed hydrolysis (93% ee).
- (S)-THIQ-3-COOH (Tic) : Widely used in peptide synthesis due to its role as a Phe analog.
Biological Relevance : Enantiomers of THIQ derivatives exhibit distinct receptor interactions. For instance, (R)-THIQ-3-COOH shows negligible activity in Bcl-2 inhibition compared to its (S)-counterpart.
Substituted Derivatives
Substituents on the THIQ core modulate pharmacological properties:
Synthetic Flexibility : The Petasis reaction allows introduction of diverse boronic acids and glyoxylic derivatives, enabling tailored substitution patterns.
Medicinal Chemistry
Biologische Aktivität
(R)-1,2,3,4-Tetrahydroisoquinoline-1-carboxylic acid (THIQCA) is a compound of significant interest in medicinal chemistry due to its diverse biological activities. This article explores its biological properties, focusing on its mechanisms of action, therapeutic potential, and synthesis methods.
Chemical Structure and Synthesis
THIQCA belongs to the tetrahydroisoquinoline family, characterized by a bicyclic structure that includes a saturated isoquinoline ring. The synthesis of THIQCA and its derivatives has been achieved through various methods, including:
- Pictet–Spengler Reaction : A classical method for synthesizing tetrahydroisoquinolines.
- Chemoenzymatic Approaches : Utilizing enzymes like D-amino acid oxidase for the kinetic resolution of racemic mixtures to obtain enantiomerically pure compounds .
- Diversity-Oriented Synthesis : This approach allows for the generation of a variety of derivatives with potential biological activity .
Anticancer Properties
THIQCA has shown promising anticancer activity through several mechanisms:
- Bcl-2 Inhibition : Studies indicate that THIQCA derivatives can inhibit Bcl-2 family proteins, which are crucial for regulating apoptosis in cancer cells. For instance, compound 11t demonstrated an IC50 value of 5.2 µM against Bcl-2 and induced apoptosis in Jurkat cells in a dose-dependent manner .
- Chloride Transport Enhancement : THIQCA diamides have been identified as enhancers of chloride transport in cells with mutant cystic fibrosis transmembrane conductance regulator (CFTR) proteins, improving cellular function significantly .
The biological activity of THIQCA can be attributed to several key mechanisms:
- Apoptosis Induction : Compounds derived from THIQCA activate caspase pathways leading to programmed cell death, which is vital for eliminating cancer cells.
- Protein Binding Affinity : THIQCA exhibits high binding affinities to target proteins involved in cell survival and proliferation, such as Bcl-2 and Mcl-1, while showing minimal interaction with Bcl-xL .
- Antimicrobial Activity : Some derivatives have demonstrated inhibition against bacterial enzymes like New Delhi metallo-β-lactamase (NDM-1), indicating potential as antibiotics .
Case Studies
Several studies have highlighted the efficacy of THIQCA and its derivatives:
- Study on Apoptosis : A series of substituted 1,2,3,4-tetrahydroisoquinoline-3-carboxylic acids were tested for their ability to induce apoptosis in cancer cell lines. Results showed that certain compounds significantly activated caspase-3 and reduced cell viability .
Compound | IC50 (µM) | Mechanism |
---|---|---|
11t | 5.2 | Bcl-2 Inhibition |
12a | <10 | Chloride Transport Enhancement |
Q & A
Basic Research Questions
Q. What are the primary synthetic routes for (R)-1,2,3,4-tetrahydroisoquinoline-1-carboxylic acid, and how do they differ in efficiency?
The compound is synthesized via catalytic hydrogenation of isoquinoline-1-carboxylic acid (Reissert reaction followed by hydrolysis) . Alternative methods include sodium borohydride reduction of intermediates derived from homoveratrylamine and glyoxylic acid, though yields vary (0–20%) . Recent protocols utilize lipase-catalyzed kinetic resolution (KR) or dynamic kinetic resolution (DKR) with Candida antarctica lipase B to enantioselectively resolve racemic mixtures in organic solvents . For constrained analogues, coupling reactions with Boc-protected intermediates (e.g., Boc-(R)-1,2,3,4-tetrahydroisoquinoline-3-carboxylic acid) are employed using DCC/HOBt activation .
Q. How is enantiomeric purity ensured during synthesis?
Kinetic resolution via Candida antarctica lipase B selectively hydrolyzes one enantiomer in organic solvents, achieving high enantiomeric excess (ee) for the (R)-enantiomer . DKR further enhances efficiency by dynamically racemizing the substrate during hydrolysis. Chiral chromatography or optical resolution with (+)-tartaric acid are alternatives for isolating specific diastereomers .
Q. What analytical techniques are critical for structural validation?
X-ray crystallography and DFT calculations are used to confirm spiroheterocyclic conformations and hydrogen-bonding patterns . NMR spectroscopy (e.g., H, C) resolves stereochemistry, as demonstrated in studies of acylated derivatives (e.g., 2-oleoyl-1,2,3,4-tetrahydroisoquinoline-1-carboxylic acid) . HRMS ensures molecular weight accuracy .
Advanced Research Questions
Q. How do computational methods (DFT, ab initio) aid in understanding conformational constraints?
DFT studies reveal that the tetrahydroisoquinoline scaffold adopts rigid, helical, or β-bend conformations, mimicking natural amino acids like phenylalanine or phenylglycine . These constraints enhance peptide stability and receptor binding specificity, as seen in opioid receptor antagonists .
Q. What strategies address low yields in oxidative cleavage reactions during synthesis?
Oxidative cleavage of olefinic substituents using RuO or O often fails, but KMnO-NaIO systems successfully generate N-acetyl tetrahydroisoquinoline-1-carboxylic acid . Solvent optimization (e.g., DMSO/water mixtures) improves reaction rates and stability of intermediates .
Q. How does the compound’s bioactivity relate to its structural analogues?
As a conformationally constrained phenylglycine analogue, it enhances mitochondrial activity and apoptosis induction in hepatocellular carcinoma cells when modified with indolylacrylamide groups . Phosphonic/phosphinic acid derivatives act as hydrolysis-resistant transition-state mimics, improving bioavailability and selectivity .
Q. What contradictions exist in biological activity data across studies?
Q. How are 1,3-dipolar cycloaddition reactions applied to functionalize the core scaffold?
Mesoionic oxazolium 5-oxides (munchnones) derived from the compound undergo regiospecific cycloaddition with acetylenic dipolarophiles (e.g., dimethyl acetylenedicarboxylate), yielding pyrrolo[2,1-a]isoquinolines. Photocyclization of these products generates indolizinophenanthrenes, useful in photodynamic therapy research .
Q. Methodological Considerations
Q. How do solvent and pH conditions impact stability in peptide conjugation?
In DMSO, the compound’s derivatives (e.g., Tic-peptides) undergo spontaneous 2,5-dioxopiperazine formation, accelerating degradation. Aqueous buffers (pH 7–9) stabilize the backbone but may require antioxidants to prevent oxidation of methoxy or hydroxyl substituents .
Q. What are best practices for handling hygroscopic or light-sensitive derivatives?
Store derivatives under inert gas (N/Ar) in sealed, desiccated containers. Avoid prolonged exposure to UV light, which triggers photocyclization or racemization . For acylated variants (e.g., oleoyl derivatives), use cold-chain storage to prevent hydrolysis .
Eigenschaften
IUPAC Name |
(1R)-1,2,3,4-tetrahydroisoquinoline-1-carboxylic acid | |
---|---|---|
Source | PubChem | |
URL | https://pubchem.ncbi.nlm.nih.gov | |
Description | Data deposited in or computed by PubChem | |
InChI |
InChI=1S/C10H11NO2/c12-10(13)9-8-4-2-1-3-7(8)5-6-11-9/h1-4,9,11H,5-6H2,(H,12,13)/t9-/m1/s1 | |
Source | PubChem | |
URL | https://pubchem.ncbi.nlm.nih.gov | |
Description | Data deposited in or computed by PubChem | |
InChI Key |
OXFGRWIKQDSSLY-SECBINFHSA-N | |
Source | PubChem | |
URL | https://pubchem.ncbi.nlm.nih.gov | |
Description | Data deposited in or computed by PubChem | |
Canonical SMILES |
C1CNC(C2=CC=CC=C21)C(=O)O | |
Source | PubChem | |
URL | https://pubchem.ncbi.nlm.nih.gov | |
Description | Data deposited in or computed by PubChem | |
Isomeric SMILES |
C1CN[C@H](C2=CC=CC=C21)C(=O)O | |
Source | PubChem | |
URL | https://pubchem.ncbi.nlm.nih.gov | |
Description | Data deposited in or computed by PubChem | |
Molecular Formula |
C10H11NO2 | |
Source | PubChem | |
URL | https://pubchem.ncbi.nlm.nih.gov | |
Description | Data deposited in or computed by PubChem | |
DSSTOX Substance ID |
DTXSID40424903 | |
Record name | (1R)-1,2,3,4-Tetrahydroisoquinoline-1-carboxylic acid | |
Source | EPA DSSTox | |
URL | https://comptox.epa.gov/dashboard/DTXSID40424903 | |
Description | DSSTox provides a high quality public chemistry resource for supporting improved predictive toxicology. | |
Molecular Weight |
177.20 g/mol | |
Source | PubChem | |
URL | https://pubchem.ncbi.nlm.nih.gov | |
Description | Data deposited in or computed by PubChem | |
CAS No. |
151004-93-2 | |
Record name | (1R)-1,2,3,4-Tetrahydroisoquinoline-1-carboxylic acid | |
Source | EPA DSSTox | |
URL | https://comptox.epa.gov/dashboard/DTXSID40424903 | |
Description | DSSTox provides a high quality public chemistry resource for supporting improved predictive toxicology. | |
Retrosynthesis Analysis
AI-Powered Synthesis Planning: Our tool employs the Template_relevance Pistachio, Template_relevance Bkms_metabolic, Template_relevance Pistachio_ringbreaker, Template_relevance Reaxys, Template_relevance Reaxys_biocatalysis model, leveraging a vast database of chemical reactions to predict feasible synthetic routes.
One-Step Synthesis Focus: Specifically designed for one-step synthesis, it provides concise and direct routes for your target compounds, streamlining the synthesis process.
Accurate Predictions: Utilizing the extensive PISTACHIO, BKMS_METABOLIC, PISTACHIO_RINGBREAKER, REAXYS, REAXYS_BIOCATALYSIS database, our tool offers high-accuracy predictions, reflecting the latest in chemical research and data.
Strategy Settings
Precursor scoring | Relevance Heuristic |
---|---|
Min. plausibility | 0.01 |
Model | Template_relevance |
Template Set | Pistachio/Bkms_metabolic/Pistachio_ringbreaker/Reaxys/Reaxys_biocatalysis |
Top-N result to add to graph | 6 |
Feasible Synthetic Routes
Haftungsausschluss und Informationen zu In-Vitro-Forschungsprodukten
Bitte beachten Sie, dass alle Artikel und Produktinformationen, die auf BenchChem präsentiert werden, ausschließlich zu Informationszwecken bestimmt sind. Die auf BenchChem zum Kauf angebotenen Produkte sind speziell für In-vitro-Studien konzipiert, die außerhalb lebender Organismen durchgeführt werden. In-vitro-Studien, abgeleitet von dem lateinischen Begriff "in Glas", beinhalten Experimente, die in kontrollierten Laborumgebungen unter Verwendung von Zellen oder Geweben durchgeführt werden. Es ist wichtig zu beachten, dass diese Produkte nicht als Arzneimittel oder Medikamente eingestuft sind und keine Zulassung der FDA für die Vorbeugung, Behandlung oder Heilung von medizinischen Zuständen, Beschwerden oder Krankheiten erhalten haben. Wir müssen betonen, dass jede Form der körperlichen Einführung dieser Produkte in Menschen oder Tiere gesetzlich strikt untersagt ist. Es ist unerlässlich, sich an diese Richtlinien zu halten, um die Einhaltung rechtlicher und ethischer Standards in Forschung und Experiment zu gewährleisten.