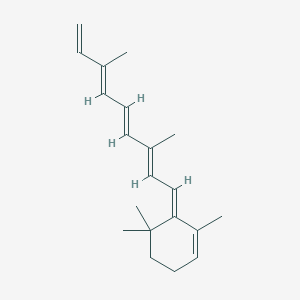
Anhydrovitamin A
Übersicht
Beschreibung
Anhydrovitamin A refers to a group of dehydrated derivatives of vitamin A (retinol) formed via acid-catalyzed dehydration or enzymatic processes. These compounds are structurally characterized by the loss of a water molecule, resulting in conjugated polyene systems with distinct spectral and chemical properties. Key forms include this compound₁ (anhydroretinol) and this compound₂ (3-hydroxyanhydroretinol).
- Structural Features: this compound₁ (C₂₀H₂₈O) is derived from retinol through dehydration, forming a conjugated hexaene system . this compound₂ (C₂₀H₂₈O₂) contains an additional hydroxyl group at the C-3 position, confirmed via UV-Vis, IR, NMR, and mass spectrometry . Natural this compound₂ in freshwater fish (e.g., Bagarius bagarius) is further converted to 3-dehydroretinol (retinene₂) .
- Synthesis and Natural Occurrence: Synthesized chemically by treating retinol or dehydroretinol with acids (e.g., HCl or toluenesulfonic acid) . Found in fish liver oils, particularly in freshwater species like Saccobranchus and Wallago attu, where enzymatic dehydration of 3-hydroxyretinol occurs .
- Spectroscopic Properties: UV-Vis maxima: this compound₁ (371 nm), A₂ (350, 368, 390 nm) in ethanol . Antimony trichloride reaction: A₁ produces a green color (λₘₐₓ 693 nm), while A₂ yields a stronger absorbance (E₁% 1884 at 693 nm) .
Vorbereitungsmethoden
Synthetic Routes and Reaction Conditions: Anhydrovitamin A is synthesized by treating vitamin A alcohol with a strong mineral acid in an anhydrous solvent . This reaction causes the vitamin A molecule to lose a water molecule, forming this compound. The process involves ionization of vitamin A in the presence of acid, followed by a shift of the positive charge to a carbon atom in the ionone ring, and finally, the molecule regains its electrical neutrality by losing a proton .
Industrial Production Methods: Industrial production of this compound follows similar synthetic routes but on a larger scale. The process requires careful control of reaction conditions to ensure high yield and purity of the final product. The use of advanced chromatographic techniques and recrystallization helps in achieving the desired purity levels .
Analyse Chemischer Reaktionen
Dehydration Reactions
Anhydrovitamin A forms via acid-catalyzed dehydration of vitamin A alcohol or acetate:
-
Ethanol-HCl method : Vitamin A2 treated with ethanolic HCl yields crystalline anhydrovitamin A2 (λ_max 350, 370, 392 nm) .
-
p-Toluenesulfonic acid method : Refluxing vitamin A in benzene with catalytic acid produces this compound (λ_max 389, 368, 350 nm) .
-
UV irradiation : Exposure of vitamin A acetate in acetone to UV light (365–366 nm) generates this compound, confirmed by identical UV spectra (λ_max 388, 366, 344 nm) and Carr-Price reaction peaks (620 nm) .
Table 1: Comparative Dehydration Methods
Oxidative Transformations
Anhydrovitamin A2 undergoes oxidation to retinene2 (vitamin A2 aldehyde):
-
MnO₂ oxidation : Passing a light-petroleum solution through MnO₂ converts 6% of anhydrovitamin A2 to retinene2 .
-
Mechanism : Proposed allylic rearrangement forms a carbonium ion intermediate, followed by oxidation at the terminal methylene group .
Structural Characterization
Key spectroscopic data for anhydrovitamin A2:
Table 2: Spectroscopic Properties
Derivatization Reactions
-
Acetylation : Treatment with acetyl chloride/pyridine forms an acetyl ester (λ_max 330, 350, 368 nm), reversible via saponification .
-
Chromatography : Adsorbs strongly on deactivated alumina, eluting with 8% ether in light petroleum .
Stability and Degradation
-
Solvent effects : Degrades faster in acetone under UV than in petroleum ether .
-
Thermal stability : Dehydration via acid reflux (benzene, 2 hr) confirms thermal lability .
Proposed Reaction Mechanism
The MnO₂-mediated oxidation involves:
-
Adsorption-induced hydroxyl group elimination.
-
Carbonium ion formation (C-3 positive charge).
-
Allylic rearrangement and terminal oxidation:
This synthesis of reactions highlights this compound’s role as a retro-dehydration product with distinct reactivity, bridging vitamin A metabolism and synthetic derivatization pathways. Further studies are needed to confirm the tentative 3-hydroxyanhydroretinol structure .
Wissenschaftliche Forschungsanwendungen
Anhydrovitamin A hat mehrere Anwendungen in der wissenschaftlichen Forschung:
Chemie: Es wird als Modellverbindung verwendet, um das Verhalten von Sesquiterpenen und deren Derivaten zu untersuchen.
Biologie: Die Forschung konzentriert sich auf seine potenziellen biologischen Aktivitäten und Wechselwirkungen mit zellulären Komponenten.
Medizin: Studien untersuchen seine potenziellen therapeutischen Anwendungen, einschließlich seiner Rolle bei der Sehkraft und der Regulierung des Immunsystems.
Industrie: Es wird bei der Herstellung verschiedener Vitamin-A-Derivate und verwandter Verbindungen verwendet
5. Wirkmechanismus
Der Wirkmechanismus von this compound beinhaltet seine Wechselwirkung mit spezifischen molekularen Zielstrukturen und Signalwegen. Es wird angenommen, dass es seine Wirkungen entfaltet, indem es an Kernrezeptoren bindet und die Genexpression reguliert. Diese Interaktion beeinflusst verschiedene zelluläre Prozesse, darunter Wachstum, Differenzierung und Immunantwort .
Ähnliche Verbindungen:
Vitamin A (Retinol): Die Stammverbindung, aus der this compound gewonnen wird.
Isothis compound: Ein strukturelles Isomer mit ähnlichen Eigenschaften.
Subvitamin A: Eine weitere verwandte Verbindung, die in Fischleberölen vorkommt.
Einzigartigkeit: this compound ist einzigartig aufgrund seiner spezifischen Struktur mit sechs konjugierten Doppelbindungen, die ihm besondere chemische und biologische Eigenschaften verleihen. Seine Fähigkeit, verschiedene chemische Reaktionen einzugehen, und seine potenziellen biologischen Aktivitäten machen es zu einer Verbindung von großem Interesse in der wissenschaftlichen Forschung .
Wirkmechanismus
The mechanism of action of anhydrovitamin A involves its interaction with specific molecular targets and pathways. It is believed to exert its effects by binding to nuclear receptors and regulating gene transcription. This interaction influences various cellular processes, including growth, differentiation, and immune response .
Vergleich Mit ähnlichen Verbindungen
Structural and Spectral Differences
Analytical Differentiation
- Polarography : this compound can be distinguished from vitamin A acetate via derivative oscillographic voltammetry due to differences in kinetic basicity .
- Resonance Raman Spectroscopy : this compound’s spectrum (77 K in hexane) closely matches retinal’s protonated Schiff base, aiding in structural identification .
- Chromatography : this compound₂’s hydroxyl group increases adsorption on alumina, enabling separation from A₁ .
Key Research Findings and Implications
- Synthetic Utility: this compound is used to quantify retinol via dehydration-based assays, leveraging its distinct spectral properties .
- Dietary Significance: 3,4-didehydroretinol (A₂) in small fish is proposed as a sustainable dietary source to combat vitamin A deficiency .
- Pharmacological Potential: Despite weak antitubercular activity, structural analogs of this compound inspire synthetic retinoids for dermatological and oncological applications .
Data Table: Comparative Analysis of Vitamin A Derivatives
Property | This compound₁ | This compound₂ | Retinol (A₁) | 3,4-Didehydroretinol (A₂) | Retinene |
---|---|---|---|---|---|
Molecular Formula | C₂₀H₂₈O | C₂₀H₂₈O₂ | C₂₀H₃₀O | C₂₀H₂₈O | C₂₀H₂₈O |
Key Functional Groups | None | 3-OH | -OH | 3,4-diene | -CHO |
UV-Vis λₘₐₓ (nm) | 371 | 350, 368, 390 | 325 | 350 | 387 |
Biological Role | Metabolic byproduct | Provitamin A₂ | Vision, immunity | Vitamin A₂ precursor | Vision |
Natural Source | Marine fish oils | Freshwater fish oils | Animal tissues | Freshwater fish | Retinal cells |
Biologische Aktivität
Anhydrovitamin A, also known as retinol or vitamin A, is a fat-soluble vitamin crucial for various biological functions. Its biological activity extends beyond its well-known role in vision and growth; it plays significant roles in cellular signaling, immune function, and gene expression. This article reviews the biological activity of this compound, supported by relevant research findings, case studies, and data tables.
Overview of this compound
This compound is primarily recognized for its role as a precursor to retinoic acid, a potent signaling molecule involved in regulating gene expression. It is derived from dietary sources and is essential for maintaining various physiological functions.
Mechanisms of Biological Activity
- Gene Regulation : this compound influences gene expression by activating nuclear receptors known as retinoic acid receptors (RARs). These receptors bind to retinoic acid and regulate transcription of genes involved in cell differentiation and proliferation .
- Cell Signaling : Recent studies have identified this compound as a co-factor for protein kinase C (PKC) and other serine/threonine kinases. This binding facilitates redox activation, linking the one-electron redox chemistry of cytochrome c with the two-electron chemistry of PKC . This unique interaction underscores the compound's role in mitochondrial energetics and cellular signaling pathways.
- Immune Function : this compound is vital for maintaining immune system integrity. It enhances the differentiation of T cells and promotes the production of antibodies, thereby improving immune responses against infections .
Case Study 1: Vitamin A Deficiency in Rats
A study on rats demonstrated that oral administration of 5,6-monoepoxyvitamin A significantly alleviated symptoms of vitamin A deficiency. The compound was metabolized similarly to vitamin A aldehyde, promoting growth and restoring normal physiological functions .
Treatment Type | Effect on Vitamin A Deficiency |
---|---|
Oral Administration | Alleviated symptoms |
Intraperitoneal Injection | Accumulation in liver |
Subcutaneous Injection | Restored growth |
Case Study 2: Retinoic Acid and Immune Response
Research indicated that retinoic acid derived from this compound enhances T cell differentiation. In a controlled experiment, mice receiving retinoic acid showed improved antibody production compared to those with no supplementation .
Biological Potency
The biological potency of this compound is significant. For instance, 5,6-monoepoxyvitamin A was found to possess a biological potency 108% that of all-trans vitamin A acetate when tested in vitamin A-deficient rats . This finding suggests that derivatives of this compound may offer enhanced therapeutic benefits.
Toxicology and Safety
Although this compound is essential for health, excessive intake can lead to toxicity. Studies indicate that while acute oral toxicity is low at normal dietary levels, chronic overconsumption can exceed tolerable upper intake levels (UL), leading to adverse effects such as liver damage and teratogenicity .
Q & A
Basic Research Questions
Q. What analytical methods are most reliable for identifying and quantifying Anhydrovitamin A in biological samples?
- Methodological Answer : High-performance liquid chromatography (HPLC) coupled with UV-Vis or mass spectrometry is widely used due to its sensitivity to retinoid isomers. For quantification, calibration with standardized reference materials (e.g., crystalline this compound) is critical to avoid interference from degradation products. Internal standards like retinyl acetate can improve accuracy .
- Experimental Design : Include validation steps such as spike-and-recovery tests and inter-laboratory comparisons to ensure reproducibility. Sample preparation should minimize light and oxygen exposure to prevent isomerization .
Q. How can researchers differentiate this compound from structurally similar retinoids during synthesis?
- Methodological Answer : Infrared (IR) spectroscopy and nuclear magnetic resonance (NMR) are essential for structural confirmation. Key spectral markers include C=O stretching vibrations (IR) and proton shifts associated with conjugated double bonds (NMR). Chromatographic separation (e.g., silica gel column chromatography) followed by crystallography can isolate pure this compound from byproducts like retro-vitamin A .
- Data Validation : Compare retention times and spectral data with published reference libraries (e.g., Budowski & Bondi, 1957) .
Q. What are the challenges in detecting this compound in vivo, and how can they be addressed?
- Methodological Answer : Low bioavailability and rapid metabolism necessitate sensitive detection methods. Radiolabeled tracers (e.g., ³H- or ¹⁴C-Anhydrovitamin A) can track metabolic pathways. Liver homogenate assays combined with liquid scintillation counting are recommended for quantifying tissue-specific uptake .
- Experimental Pitfalls : Subcutaneous or oral administration in model organisms (e.g., rats) may yield negligible liver storage, requiring alternative endpoints like growth assays or histological analysis of epithelial tissues .
Advanced Research Questions
Q. How do metabolic pathways explain the limited bioactivity of this compound compared to vitamin A?
- Methodological Answer : this compound lacks the hydroxyl group required for direct conversion to retinoic acid. Instead, its bioactivity depends on hepatic hydroxylation to derivatives like 4-hydroxy-Anhydrovitamin A, which may transiently bind to retinol-binding proteins (RBPs). Use knockout models (e.g., RBP4⁻/⁻ mice) to isolate its metabolic fate .
- Data Contradictions : While liver storage assays show negligible retention, growth promotion in deficient rats suggests indirect activity via metabolites. Resolve this by profiling urinary metabolites via LC-MS/MS .
Q. What experimental designs can resolve discrepancies in reported biological potency of this compound?
- Methodological Answer : Standardize bioassays using controlled diets and genetically homogenous animal cohorts. For example, vaginal smear assays in vitamin A-deficient rats reveal transient normalization of epithelial cells after high-dose this compound administration, but potency estimates vary due to rapid depletion. Combine multiple endpoints (e.g., growth rate, liver histology) to triangulate activity .
- Critical Analysis : Earlier studies (e.g., Shantz et al., 1943) reported ~0.4% potency relative to vitamin A, but later work found no liver storage. Reconcile these by distinguishing between direct activity and metabolite-mediated effects .
Q. How can AI-driven data analysis improve the reliability of this compound research?
- Methodological Answer : Machine learning algorithms (e.g., neural networks) can identify patterns in complex datasets, such as correlating spectral data with bioactivity. For example, train models on published LC-MS datasets to predict metabolite stability or toxicity .
- Implementation : Use open-source tools like Python’s Scikit-learn for regression analysis of dose-response curves. Ensure transparency by sharing raw data and code repositories .
Q. What structural modifications could enhance this compound’s stability for therapeutic applications?
- Methodological Answer : Introduce electron-withdrawing groups (e.g., fluorine) at the C4 position to reduce oxidative degradation. Computational chemistry tools (e.g., DFT simulations) can predict bond dissociation energies and guide synthesis. Validate stability via accelerated aging tests under UV light .
- Experimental Validation : Compare half-lives of modified analogs using HPLC-UV under standardized conditions (pH 7.4, 37°C) .
Q. Tables for Reference
Table 1 : Comparative Bioactivity of Vitamin A Derivatives in Rat Models
Table 2 : Recommended Analytical Techniques for this compound Research
Technique | Application | Sensitivity Limit | Key Advantage |
---|---|---|---|
HPLC-UV | Quantification in serum | 0.1 ng/mL | High reproducibility |
LC-MS/MS | Metabolite profiling | 0.01 ng/mL | Structural specificity |
¹H-NMR | Structural confirmation | 1 µg sample | Non-destructive |
Q. Guidelines for Rigorous Research
- Data Quality : Adhere to Greenfield & Southgate (1992) guidelines for method documentation, including sampling protocols and recovery rates .
- Ethical Compliance : Use institutional animal care protocols (IACUC) for in vivo studies, especially when testing high-dose effects .
- Open Science : Share datasets via repositories like Zenodo to enable meta-analyses and reduce publication bias .
Eigenschaften
IUPAC Name |
(6E)-6-[(2E,4E,6E)-3,7-dimethylnona-2,4,6,8-tetraenylidene]-1,5,5-trimethylcyclohexene | |
---|---|---|
Source | PubChem | |
URL | https://pubchem.ncbi.nlm.nih.gov | |
Description | Data deposited in or computed by PubChem | |
InChI |
InChI=1S/C20H28/c1-7-16(2)10-8-11-17(3)13-14-19-18(4)12-9-15-20(19,5)6/h7-8,10-14H,1,9,15H2,2-6H3/b11-8+,16-10+,17-13+,19-14- | |
Source | PubChem | |
URL | https://pubchem.ncbi.nlm.nih.gov | |
Description | Data deposited in or computed by PubChem | |
InChI Key |
FWNRILWHNGFAIN-OYUWDNMLSA-N | |
Source | PubChem | |
URL | https://pubchem.ncbi.nlm.nih.gov | |
Description | Data deposited in or computed by PubChem | |
Canonical SMILES |
CC1=CCCC(C1=CC=C(C)C=CC=C(C)C=C)(C)C | |
Source | PubChem | |
URL | https://pubchem.ncbi.nlm.nih.gov | |
Description | Data deposited in or computed by PubChem | |
Isomeric SMILES |
CC\1=CCCC(/C1=C\C=C(/C)\C=C\C=C(/C)\C=C)(C)C | |
Source | PubChem | |
URL | https://pubchem.ncbi.nlm.nih.gov | |
Description | Data deposited in or computed by PubChem | |
Molecular Formula |
C20H28 | |
Source | PubChem | |
URL | https://pubchem.ncbi.nlm.nih.gov | |
Description | Data deposited in or computed by PubChem | |
DSSTOX Substance ID |
DTXSID101123105 | |
Record name | (6E)-6-[(2E,4E,6E)-3,7-Dimethyl-2,4,6,8-nonatetraen-1-ylidene]-1,5,5-trimethylcyclohexene | |
Source | EPA DSSTox | |
URL | https://comptox.epa.gov/dashboard/DTXSID101123105 | |
Description | DSSTox provides a high quality public chemistry resource for supporting improved predictive toxicology. | |
Molecular Weight |
268.4 g/mol | |
Source | PubChem | |
URL | https://pubchem.ncbi.nlm.nih.gov | |
Description | Data deposited in or computed by PubChem | |
CAS No. |
1224-78-8 | |
Record name | (6E)-6-[(2E,4E,6E)-3,7-Dimethyl-2,4,6,8-nonatetraen-1-ylidene]-1,5,5-trimethylcyclohexene | |
Source | CAS Common Chemistry | |
URL | https://commonchemistry.cas.org/detail?cas_rn=1224-78-8 | |
Description | CAS Common Chemistry is an open community resource for accessing chemical information. Nearly 500,000 chemical substances from CAS REGISTRY cover areas of community interest, including common and frequently regulated chemicals, and those relevant to high school and undergraduate chemistry classes. This chemical information, curated by our expert scientists, is provided in alignment with our mission as a division of the American Chemical Society. | |
Explanation | The data from CAS Common Chemistry is provided under a CC-BY-NC 4.0 license, unless otherwise stated. | |
Record name | Anhydrovitamin A | |
Source | ChemIDplus | |
URL | https://pubchem.ncbi.nlm.nih.gov/substance/?source=chemidplus&sourceid=0001224788 | |
Description | ChemIDplus is a free, web search system that provides access to the structure and nomenclature authority files used for the identification of chemical substances cited in National Library of Medicine (NLM) databases, including the TOXNET system. | |
Record name | Anhydrovitamin A | |
Source | DrugBank | |
URL | https://www.drugbank.ca/drugs/DB02914 | |
Description | The DrugBank database is a unique bioinformatics and cheminformatics resource that combines detailed drug (i.e. chemical, pharmacological and pharmaceutical) data with comprehensive drug target (i.e. sequence, structure, and pathway) information. | |
Explanation | Creative Common's Attribution-NonCommercial 4.0 International License (http://creativecommons.org/licenses/by-nc/4.0/legalcode) | |
Record name | (6E)-6-[(2E,4E,6E)-3,7-Dimethyl-2,4,6,8-nonatetraen-1-ylidene]-1,5,5-trimethylcyclohexene | |
Source | EPA DSSTox | |
URL | https://comptox.epa.gov/dashboard/DTXSID101123105 | |
Description | DSSTox provides a high quality public chemistry resource for supporting improved predictive toxicology. | |
Record name | ANHYDROVITAMIN A | |
Source | FDA Global Substance Registration System (GSRS) | |
URL | https://gsrs.ncats.nih.gov/ginas/app/beta/substances/235BBF3K97 | |
Description | The FDA Global Substance Registration System (GSRS) enables the efficient and accurate exchange of information on what substances are in regulated products. Instead of relying on names, which vary across regulatory domains, countries, and regions, the GSRS knowledge base makes it possible for substances to be defined by standardized, scientific descriptions. | |
Explanation | Unless otherwise noted, the contents of the FDA website (www.fda.gov), both text and graphics, are not copyrighted. They are in the public domain and may be republished, reprinted and otherwise used freely by anyone without the need to obtain permission from FDA. Credit to the U.S. Food and Drug Administration as the source is appreciated but not required. | |
Retrosynthesis Analysis
AI-Powered Synthesis Planning: Our tool employs the Template_relevance Pistachio, Template_relevance Bkms_metabolic, Template_relevance Pistachio_ringbreaker, Template_relevance Reaxys, Template_relevance Reaxys_biocatalysis model, leveraging a vast database of chemical reactions to predict feasible synthetic routes.
One-Step Synthesis Focus: Specifically designed for one-step synthesis, it provides concise and direct routes for your target compounds, streamlining the synthesis process.
Accurate Predictions: Utilizing the extensive PISTACHIO, BKMS_METABOLIC, PISTACHIO_RINGBREAKER, REAXYS, REAXYS_BIOCATALYSIS database, our tool offers high-accuracy predictions, reflecting the latest in chemical research and data.
Strategy Settings
Precursor scoring | Relevance Heuristic |
---|---|
Min. plausibility | 0.01 |
Model | Template_relevance |
Template Set | Pistachio/Bkms_metabolic/Pistachio_ringbreaker/Reaxys/Reaxys_biocatalysis |
Top-N result to add to graph | 6 |
Feasible Synthetic Routes
Haftungsausschluss und Informationen zu In-Vitro-Forschungsprodukten
Bitte beachten Sie, dass alle Artikel und Produktinformationen, die auf BenchChem präsentiert werden, ausschließlich zu Informationszwecken bestimmt sind. Die auf BenchChem zum Kauf angebotenen Produkte sind speziell für In-vitro-Studien konzipiert, die außerhalb lebender Organismen durchgeführt werden. In-vitro-Studien, abgeleitet von dem lateinischen Begriff "in Glas", beinhalten Experimente, die in kontrollierten Laborumgebungen unter Verwendung von Zellen oder Geweben durchgeführt werden. Es ist wichtig zu beachten, dass diese Produkte nicht als Arzneimittel oder Medikamente eingestuft sind und keine Zulassung der FDA für die Vorbeugung, Behandlung oder Heilung von medizinischen Zuständen, Beschwerden oder Krankheiten erhalten haben. Wir müssen betonen, dass jede Form der körperlichen Einführung dieser Produkte in Menschen oder Tiere gesetzlich strikt untersagt ist. Es ist unerlässlich, sich an diese Richtlinien zu halten, um die Einhaltung rechtlicher und ethischer Standards in Forschung und Experiment zu gewährleisten.