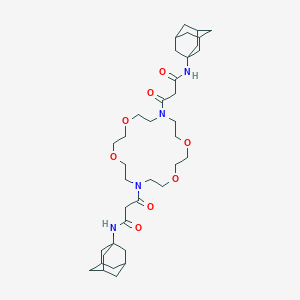
Magnesium ionophore VII
Übersicht
Beschreibung
Vorbereitungsmethoden
Synthetic Routes and Reaction Conditions: The synthesis of magnesium ionophore VII involves the reaction of diaza-18-crown-6 with adamantyl isocyanate under controlled conditions. The reaction typically occurs in an organic solvent such as dichloromethane, with the temperature maintained at room temperature to ensure optimal yield and purity .
Industrial Production Methods: Industrial production of this compound follows a similar synthetic route but on a larger scale. The process involves the use of high-purity reagents and solvents, with stringent control over reaction conditions to ensure consistency and quality. The final product is purified using techniques such as recrystallization or chromatography to achieve the desired purity levels .
Analyse Chemischer Reaktionen
Types of Reactions: Magnesium ionophore VII primarily undergoes complexation reactions with magnesium ions. It does not typically participate in oxidation, reduction, or substitution reactions due to its stable structure .
Common Reagents and Conditions: The primary reagent used with this compound is magnesium chloride, which provides the magnesium ions for complexation. The reaction conditions are typically mild, with the process occurring at room temperature in an aqueous or organic solvent .
Major Products: The major product of the reaction between this compound and magnesium chloride is a stable magnesium-ionophore complex. This complex is highly selective for magnesium ions, making it useful for various analytical and research applications .
Wissenschaftliche Forschungsanwendungen
Magnesium ionophore VII has a wide range of applications in scientific research:
Wirkmechanismus
Magnesium ionophore VII functions by forming a complex with magnesium ions, effectively transporting them across lipid membranes. The compound’s structure includes a lipophilic cavity that binds magnesium ions, facilitating their movement through the hydrophobic environment of the membrane . This process is essential for maintaining ion balance within cells and is critical for various biochemical and physiological functions .
Vergleich Mit ähnlichen Verbindungen
Magnesium ionophore VII is unique due to its high selectivity and affinity for magnesium ions. Similar compounds include:
Magnesium ionophore I: Another synthetic ionophore with a different structure but similar function.
Magnesium ionophore III: Known for its effectiveness in binding magnesium ions, though with slightly different selectivity properties.
Calcium ionophore II: While primarily selective for calcium ions, it can also interact with magnesium ions under certain conditions.
This compound stands out due to its superior selectivity and stability, making it a preferred choice for applications requiring precise magnesium ion detection and transport .
Eigenschaften
IUPAC Name |
N-(1-adamantyl)-3-[16-[3-(1-adamantylamino)-3-oxopropanoyl]-1,4,10,13-tetraoxa-7,16-diazacyclooctadec-7-yl]-3-oxopropanamide | |
---|---|---|
Source | PubChem | |
URL | https://pubchem.ncbi.nlm.nih.gov | |
Description | Data deposited in or computed by PubChem | |
InChI |
InChI=1S/C38H60N4O8/c43-33(39-37-21-27-13-28(22-37)15-29(14-27)23-37)19-35(45)41-1-5-47-9-10-49-7-3-42(4-8-50-12-11-48-6-2-41)36(46)20-34(44)40-38-24-30-16-31(25-38)18-32(17-30)26-38/h27-32H,1-26H2,(H,39,43)(H,40,44) | |
Source | PubChem | |
URL | https://pubchem.ncbi.nlm.nih.gov | |
Description | Data deposited in or computed by PubChem | |
InChI Key |
IPVOJCDBVJUPRP-UHFFFAOYSA-N | |
Source | PubChem | |
URL | https://pubchem.ncbi.nlm.nih.gov | |
Description | Data deposited in or computed by PubChem | |
Canonical SMILES |
C1COCCOCCN(CCOCCOCCN1C(=O)CC(=O)NC23CC4CC(C2)CC(C4)C3)C(=O)CC(=O)NC56CC7CC(C5)CC(C7)C6 | |
Source | PubChem | |
URL | https://pubchem.ncbi.nlm.nih.gov | |
Description | Data deposited in or computed by PubChem | |
Molecular Formula |
C38H60N4O8 | |
Source | PubChem | |
URL | https://pubchem.ncbi.nlm.nih.gov | |
Description | Data deposited in or computed by PubChem | |
DSSTOX Substance ID |
DTXSID10583334 | |
Record name | 3,3'-(1,4,10,13-Tetraoxa-7,16-diazacyclooctadecane-7,16-diyl)bis[3-oxo-N-(tricyclo[3.3.1.1~3,7~]decan-1-yl)propanamide] | |
Source | EPA DSSTox | |
URL | https://comptox.epa.gov/dashboard/DTXSID10583334 | |
Description | DSSTox provides a high quality public chemistry resource for supporting improved predictive toxicology. | |
Molecular Weight |
700.9 g/mol | |
Source | PubChem | |
URL | https://pubchem.ncbi.nlm.nih.gov | |
Description | Data deposited in or computed by PubChem | |
CAS No. |
156210-12-7 | |
Record name | 3,3'-(1,4,10,13-Tetraoxa-7,16-diazacyclooctadecane-7,16-diyl)bis[3-oxo-N-(tricyclo[3.3.1.1~3,7~]decan-1-yl)propanamide] | |
Source | EPA DSSTox | |
URL | https://comptox.epa.gov/dashboard/DTXSID10583334 | |
Description | DSSTox provides a high quality public chemistry resource for supporting improved predictive toxicology. | |
Retrosynthesis Analysis
AI-Powered Synthesis Planning: Our tool employs the Template_relevance Pistachio, Template_relevance Bkms_metabolic, Template_relevance Pistachio_ringbreaker, Template_relevance Reaxys, Template_relevance Reaxys_biocatalysis model, leveraging a vast database of chemical reactions to predict feasible synthetic routes.
One-Step Synthesis Focus: Specifically designed for one-step synthesis, it provides concise and direct routes for your target compounds, streamlining the synthesis process.
Accurate Predictions: Utilizing the extensive PISTACHIO, BKMS_METABOLIC, PISTACHIO_RINGBREAKER, REAXYS, REAXYS_BIOCATALYSIS database, our tool offers high-accuracy predictions, reflecting the latest in chemical research and data.
Strategy Settings
Precursor scoring | Relevance Heuristic |
---|---|
Min. plausibility | 0.01 |
Model | Template_relevance |
Template Set | Pistachio/Bkms_metabolic/Pistachio_ringbreaker/Reaxys/Reaxys_biocatalysis |
Top-N result to add to graph | 6 |
Feasible Synthetic Routes
Haftungsausschluss und Informationen zu In-Vitro-Forschungsprodukten
Bitte beachten Sie, dass alle Artikel und Produktinformationen, die auf BenchChem präsentiert werden, ausschließlich zu Informationszwecken bestimmt sind. Die auf BenchChem zum Kauf angebotenen Produkte sind speziell für In-vitro-Studien konzipiert, die außerhalb lebender Organismen durchgeführt werden. In-vitro-Studien, abgeleitet von dem lateinischen Begriff "in Glas", beinhalten Experimente, die in kontrollierten Laborumgebungen unter Verwendung von Zellen oder Geweben durchgeführt werden. Es ist wichtig zu beachten, dass diese Produkte nicht als Arzneimittel oder Medikamente eingestuft sind und keine Zulassung der FDA für die Vorbeugung, Behandlung oder Heilung von medizinischen Zuständen, Beschwerden oder Krankheiten erhalten haben. Wir müssen betonen, dass jede Form der körperlichen Einführung dieser Produkte in Menschen oder Tiere gesetzlich strikt untersagt ist. Es ist unerlässlich, sich an diese Richtlinien zu halten, um die Einhaltung rechtlicher und ethischer Standards in Forschung und Experiment zu gewährleisten.