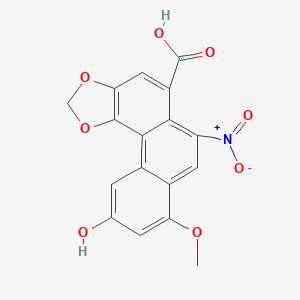
Aristolochiasäure d
Übersicht
Beschreibung
Aristolochic acid D is a member of the aristolochic acids family, which are naturally occurring compounds found in the Aristolochiaceae family of plants, including the genera Aristolochia and Asarum . These compounds are known for their potent nephrotoxic and carcinogenic properties . Aristolochic acid D, like its analogs, has been associated with severe health risks, including kidney damage and cancer .
Wissenschaftliche Forschungsanwendungen
Toxicology Studies
Aristolochic acid D has been extensively studied for its toxicological effects, particularly its role in aristolochic acid nephropathy (AAN). AAN is a condition resulting from the consumption of herbal remedies containing aristolochic acids, leading to kidney damage and increased cancer risk.
- Case Study : A study reported that patients exposed to AA-D through traditional herbal remedies developed significant renal impairment and urothelial carcinoma. Over 100 cases of AAN have been documented globally, highlighting the compound's nephrotoxic effects .
Carcinogenicity Research
AA-D is classified as a potent human carcinogen. It is associated with mutations that can lead to various cancers, particularly urothelial carcinoma of the upper urinary tract.
- Data Table: Carcinogenic Effects of Aristolochic Acid D
Antitumor Activity
Despite its toxic effects, there is ongoing research into the potential antitumor properties of aristolochic acids, including AA-D. Some studies suggest that structural modifications could enhance its therapeutic benefits while mitigating toxicity.
- Future Directions : Researchers are encouraged to explore the antitumor efficacy of AA-D and its derivatives, considering modifications that could reduce toxicity while maintaining effectiveness .
Analytical Chemistry
AA-D serves as a critical marker in analytical chemistry for identifying Aristolochiaceous plants due to its unique mutational signature. Techniques such as ultra-high-performance liquid chromatography coupled with high-resolution mass spectrometry (UHPLC-HR-MS) have been employed to detect AA-D in various samples.
- Data Table: Detection Methods for Aristolochic Acid D
Method | Sensitivity | Selectivity |
---|---|---|
UHPLC-HR-MS | High | High |
DNA Barcoding | Moderate | Low |
Wirkmechanismus
Target of Action
Aristolochic Acid D (AAD), like other aristolochic acids, primarily targets the kidney . Within the kidney, necrotic and apoptotic cell death occurs exclusively in the proximal tubule . This nephron segment is particularly prone to toxic injury due to its important role in urinary elimination of drugs and other xenobiotics .
Mode of Action
The carcinogenic effects of aristolochic acids are thought to be a result of mutation of the tumor suppressor gene TP53, which seems to be unique to aristolochic acid-associated carcinogenesis .
Biochemical Pathways
AAD activates NF-κB and STAT3 signaling pathways in hepatocytes, which may contribute to the inflammatory response and apoptosis . In liver sinusoidal endothelial cells (LSECs), AAD activates multiple oxidative stress and inflammatory associated signaling pathways and induces apoptosis . Importantly, AAD induces infiltration of cytotoxic T cells and activation of proinflammatory macrophage and neutrophil cells in the liver to produce inflammatory cytokines to aggravate inflammation .
Result of Action
AAD, acting as a carcinogen, causes otherwise rare urothelial carcinomas of the upper urinary tract, whereas its nephrotoxic effects in the kidney lead to irreversible chronic renal disease . In addition to being a urothelial carcinogen, AAD is a powerful and remarkably selective nephrotoxin .
Action Environment
Exposure to AAD is a significant risk factor for severe nephropathy and urologic, hepatobiliary, and potentially other cancers . Despite this, herbal medicinal products containing AAD continue to be manufactured and marketed worldwide with inadequate regulation, and possible environmental exposure routes receive little attention . As the trade of food and dietary supplements becomes increasingly globalized, further inaction on curtailing AAD exposure will have far-reaching negative effects on the disease trends of AAD-associated cancers .
Biochemische Analyse
Biochemical Properties
Aristolochic Acid D, like other AAs, interacts with various enzymes, proteins, and other biomolecules. It is metabolized by oxidation and reduction pathways, or phase I metabolism .
Cellular Effects
Aristolochic Acid D has been shown to have significant effects on various types of cells and cellular processes. In hepatocytes, it has been found to activate NF-κB and STAT3 signaling pathways, which may contribute to the inflammatory response and apoptosis . It also induces infiltration of cytotoxic T cells and activation of proinflammatory macrophage and neutrophil cells in the liver to produce inflammatory cytokines to aggravate inflammation .
Molecular Mechanism
At the molecular level, Aristolochic Acid D exerts its effects through binding interactions with biomolecules, enzyme inhibition or activation, and changes in gene expression. It is enzymatically reduced to reactive intermediates that generate DNA adducts . A mutational signature predominantly characterized by A→T transversions has been detected in AA-induced UTUC tumor tissues .
Temporal Effects in Laboratory Settings
The effects of Aristolochic Acid D change over time in laboratory settings. While specific information on Aristolochic Acid D’s stability, degradation, and long-term effects on cellular function is limited, it is known that the progressive lesions and mutational events initiated by AAs are irreversible .
Metabolic Pathways
Aristolochic Acid D is involved in various metabolic pathways. It is metabolized by oxidation and reduction pathways, or phase I metabolism
Transport and Distribution
It is known that AAs are metabolized in the liver and then transported via circulation to exert their effects .
Vorbereitungsmethoden
Synthetic Routes and Reaction Conditions: The synthesis of aristolochic acid D involves several steps, starting from the basic phenanthrene structure. The process typically includes nitration, reduction, and cyclization reactions. Specific conditions such as temperature, solvents, and catalysts are crucial for the successful synthesis of this compound .
Industrial Production Methods: Industrial production of aristolochic acid D is not common due to its toxic nature. extraction from natural sources, such as Aristolochia plants, is a method used in research settings. Techniques like pressurized liquid extraction (PLE) and supercritical fluid extraction (SFE) are employed to isolate aristolochic acids from plant materials .
Analyse Chemischer Reaktionen
Arten von Reaktionen: Aristolochiasäure D unterliegt verschiedenen chemischen Reaktionen, darunter:
Oxidation: Diese Reaktion kann zur Bildung von Chinonen und anderen oxidierten Derivaten führen.
Reduktion: Reduktionsreaktionen können Nitrogruppen in Aminogruppen umwandeln.
Substitution: Elektrophile aromatische Substitutionsreaktionen können verschiedene Substituenten am Phenanthrenring einführen.
Häufige Reagenzien und Bedingungen:
Oxidation: Reagenzien wie Kaliumpermanganat (KMnO4) oder Chromtrioxid (CrO3) werden häufig verwendet.
Reduktion: Katalytische Hydrierung oder Metallhydride wie Lithiumaluminiumhydrid (LiAlH4) werden eingesetzt.
Substitution: Halogenierungsmittel und Friedel-Crafts-Katalysatoren werden für Substitutionsreaktionen verwendet.
Hauptprodukte: Die Hauptprodukte, die aus diesen Reaktionen gebildet werden, umfassen verschiedene substituierte Phenanthrenderivate und reduzierte Formen von this compound .
Vergleich Mit ähnlichen Verbindungen
Aristolochic Acid I (AA-I): The most abundant and well-studied member of the aristolochic acids family.
Aristolochic Acid II (AA-II): Another major compound with similar toxicological properties.
Aristolactams: Structurally related compounds with similar biological activities
Uniqueness: Aristolochic acid D is unique due to its specific substitution pattern on the phenanthrene ring, which influences its reactivity and biological effects. Compared to AA-I and AA-II, aristolochic acid D has distinct chemical properties that make it a valuable compound for studying the structure-activity relationships within the aristolochic acids family .
Biologische Aktivität
Aristolochic acid D (AA-D) is a member of the aristolochic acid family, which is known for its significant biological activities, particularly its nephrotoxic and carcinogenic properties. This article explores the biological activity of AA-D, focusing on its mechanisms of action, effects on various biological processes, and relevant case studies.
Chemical Structure and Properties
Aristolochic acids are characterized by a common structural motif that includes a bicyclic system with a nitrogen atom. AA-D, specifically, has been identified as having distinct biological activities compared to its analogs such as AA-I and AA-II. The structure-activity relationship indicates that variations in functional groups can significantly influence the toxicity and biological effects of these compounds.
Nephrotoxicity
AA-D has been implicated in causing aristolochic acid nephropathy (AAN), a condition characterized by acute and chronic kidney injury. Studies have shown that AA-D induces apoptosis in renal cells by disrupting mitochondrial function and metabolic processes. Specifically, it binds covalently to key proteins involved in cellular respiration and metabolism, leading to impaired mitochondrial function and energy production .
Table 1: Key Mechanisms of AA-D Induced Nephrotoxicity
Carcinogenicity
AA-D is recognized as a potent human carcinogen. It forms DNA adducts that lead to mutations, particularly A:T to T:A transversions, which are prevalent in various cancers associated with aristolochic acid exposure . The formation of these adducts has been linked to urothelial carcinoma and hepatocellular carcinoma.
Table 2: Carcinogenic Effects of AA-D
Case Studies
Numerous case studies have documented the effects of AA-D exposure:
- Chronic Aristolochic Acid Nephropathy (AAN) : Over 90% of patients exposed to aristolochic acids experienced chronic kidney disease with a rapid decline in renal function. Many patients had long histories of exposure through traditional herbal remedies containing AA-D .
- Urothelial Cancer Incidence : In Taiwan, a significant correlation was established between AA exposure and the incidence of upper urinary tract urothelial carcinoma, highlighting the compound's carcinogenic potential .
Research Findings
Recent studies have utilized advanced techniques such as chemical proteomics and metabolomics to elucidate the biological activities of AA-D. These studies have identified several key metabolic enzymes affected by AA-D, including isocitrate dehydrogenase (IDH2) and lactate dehydrogenase (LDH), which are crucial for energy metabolism in cells .
Metabolic Pathways Affected by AA-D
Eigenschaften
IUPAC Name |
10-hydroxy-8-methoxy-6-nitronaphtho[2,1-g][1,3]benzodioxole-5-carboxylic acid | |
---|---|---|
Source | PubChem | |
URL | https://pubchem.ncbi.nlm.nih.gov | |
Description | Data deposited in or computed by PubChem | |
InChI |
InChI=1S/C17H11NO8/c1-24-12-3-7(19)2-9-8(12)4-11(18(22)23)14-10(17(20)21)5-13-16(15(9)14)26-6-25-13/h2-5,19H,6H2,1H3,(H,20,21) | |
Source | PubChem | |
URL | https://pubchem.ncbi.nlm.nih.gov | |
Description | Data deposited in or computed by PubChem | |
InChI Key |
PADIFGYTAXNCRK-UHFFFAOYSA-N | |
Source | PubChem | |
URL | https://pubchem.ncbi.nlm.nih.gov | |
Description | Data deposited in or computed by PubChem | |
Canonical SMILES |
COC1=CC(=CC2=C3C(=C(C=C12)[N+](=O)[O-])C(=CC4=C3OCO4)C(=O)O)O | |
Source | PubChem | |
URL | https://pubchem.ncbi.nlm.nih.gov | |
Description | Data deposited in or computed by PubChem | |
Molecular Formula |
C17H11NO8 | |
Source | PubChem | |
URL | https://pubchem.ncbi.nlm.nih.gov | |
Description | Data deposited in or computed by PubChem | |
DSSTOX Substance ID |
DTXSID40169766 | |
Record name | Aristolochic acid-D | |
Source | EPA DSSTox | |
URL | https://comptox.epa.gov/dashboard/DTXSID40169766 | |
Description | DSSTox provides a high quality public chemistry resource for supporting improved predictive toxicology. | |
Molecular Weight |
357.3 g/mol | |
Source | PubChem | |
URL | https://pubchem.ncbi.nlm.nih.gov | |
Description | Data deposited in or computed by PubChem | |
CAS No. |
17413-38-6 | |
Record name | Aristolochic acid-D | |
Source | ChemIDplus | |
URL | https://pubchem.ncbi.nlm.nih.gov/substance/?source=chemidplus&sourceid=0017413386 | |
Description | ChemIDplus is a free, web search system that provides access to the structure and nomenclature authority files used for the identification of chemical substances cited in National Library of Medicine (NLM) databases, including the TOXNET system. | |
Record name | Aristolochic acid-D | |
Source | EPA DSSTox | |
URL | https://comptox.epa.gov/dashboard/DTXSID40169766 | |
Description | DSSTox provides a high quality public chemistry resource for supporting improved predictive toxicology. | |
Retrosynthesis Analysis
AI-Powered Synthesis Planning: Our tool employs the Template_relevance Pistachio, Template_relevance Bkms_metabolic, Template_relevance Pistachio_ringbreaker, Template_relevance Reaxys, Template_relevance Reaxys_biocatalysis model, leveraging a vast database of chemical reactions to predict feasible synthetic routes.
One-Step Synthesis Focus: Specifically designed for one-step synthesis, it provides concise and direct routes for your target compounds, streamlining the synthesis process.
Accurate Predictions: Utilizing the extensive PISTACHIO, BKMS_METABOLIC, PISTACHIO_RINGBREAKER, REAXYS, REAXYS_BIOCATALYSIS database, our tool offers high-accuracy predictions, reflecting the latest in chemical research and data.
Strategy Settings
Precursor scoring | Relevance Heuristic |
---|---|
Min. plausibility | 0.01 |
Model | Template_relevance |
Template Set | Pistachio/Bkms_metabolic/Pistachio_ringbreaker/Reaxys/Reaxys_biocatalysis |
Top-N result to add to graph | 6 |
Feasible Synthetic Routes
Q1: What makes Aristolochic Acid D cytotoxic, particularly towards plant tumor cells? [, ]
A1: While the exact mechanism of Aristolochic Acid D's cytotoxicity isn't fully detailed in the provided research, one study demonstrated its negative impact on both animal and plant cells. Interestingly, this study found that plant tumor cells (specifically Crown-gall) were more sensitive to Aristolochic Acid D than animal tumor cells. [] This suggests a potential interaction with cellular pathways specific to plant cells or those upregulated in tumorigenesis. Further research is needed to elucidate the precise mechanisms of action responsible for its cytotoxic effects.
Q3: What is the significance of Aristolochic Acid D being a substrate for human UDP-glucuronosyltransferase 1As? []
A3: The research indicates that human UDP-glucuronosyltransferase 1As enzymes can catalyze the O-glucuronidation of Aristolochic Acid D. This process results in the formation of an Aristolochic Acid D glucuronide. [] Glucuronidation is a common metabolic process in the body, often leading to increased water solubility and facilitating the excretion of compounds. While the specific glucuronide formed from Aristolochic Acid D requires further study, this finding suggests a potential detoxification pathway for this compound in humans.
Haftungsausschluss und Informationen zu In-Vitro-Forschungsprodukten
Bitte beachten Sie, dass alle Artikel und Produktinformationen, die auf BenchChem präsentiert werden, ausschließlich zu Informationszwecken bestimmt sind. Die auf BenchChem zum Kauf angebotenen Produkte sind speziell für In-vitro-Studien konzipiert, die außerhalb lebender Organismen durchgeführt werden. In-vitro-Studien, abgeleitet von dem lateinischen Begriff "in Glas", beinhalten Experimente, die in kontrollierten Laborumgebungen unter Verwendung von Zellen oder Geweben durchgeführt werden. Es ist wichtig zu beachten, dass diese Produkte nicht als Arzneimittel oder Medikamente eingestuft sind und keine Zulassung der FDA für die Vorbeugung, Behandlung oder Heilung von medizinischen Zuständen, Beschwerden oder Krankheiten erhalten haben. Wir müssen betonen, dass jede Form der körperlichen Einführung dieser Produkte in Menschen oder Tiere gesetzlich strikt untersagt ist. Es ist unerlässlich, sich an diese Richtlinien zu halten, um die Einhaltung rechtlicher und ethischer Standards in Forschung und Experiment zu gewährleisten.