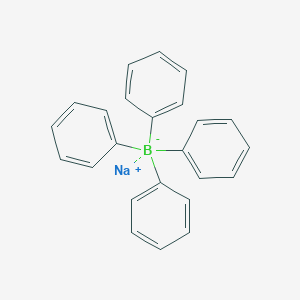
Sodium tetraphenylborate
Übersicht
Beschreibung
Sodium tetraphenylborate is an organic compound with the chemical formula NaB(C₆H₅)₄. It is a salt where the anion consists of four phenyl rings bonded to a boron atom. This white crystalline solid is used to prepare other tetraphenylborate salts, which are often highly soluble in organic solvents. This compound is widely used in inorganic and organometallic chemistry as a precipitating agent for potassium, ammonium, rubidium, and caesium ions, as well as some organic nitrogen compounds .
Vorbereitungsmethoden
Synthetic Routes and Reaction Conditions: Sodium tetraphenylborate is synthesized by the reaction between sodium tetrafluoroborate and phenylmagnesium bromide: [ \text{NaBF}_4 + 4 \text{PhMgBr} \rightarrow 2 \text{MgBr}_2 + 2 \text{MgF}_2 + \text{NaBPh}_4 ] where Ph represents the phenyl group. A related synthesis involves the use of phenylsodium in place of the Grignard reagent .
Industrial Production Methods: In industrial settings, this compound is produced by reacting sodium tetrafluoroborate with phenylmagnesium bromide under controlled conditions. The reaction is typically carried out in an organic solvent such as ether, and the product is purified by recrystallization from solvents like chloroform or acetone .
Analyse Chemischer Reaktionen
Preparation of N-Acylonium Salts
NaBPh₄ facilitates the synthesis of N-acylonium salts via reaction with tertiary amines and acid chlorides:
.
This method is effective for diverse substrates, including aliphatic and aromatic acid chlorides, and amines like triethylamine or pyridine derivatives .
Palladium-Catalyzed Cross-Coupling Reactions
NaBPh₄ acts as a phenyl donor in cross-coupling reactions with vinyl/aryl triflates under mild conditions:
Substrate Type | Product | Yield (%) | Conditions |
---|---|---|---|
Vinyl triflate | Arylalkene | 75–90 | Pd(PPh₃)₄, THF, 25°C |
Aryl triflate | Biaryl compound | 65–85 | Pd(OAc)₂, DMF, 60°C |
These reactions proceed via oxidative addition of the triflate to palladium, followed by transmetallation with BPh₄⁻ .
Catalytic Degradation Studies
Research on NaBPh₄ degradation under catalytic conditions revealed:
Catalyst System | Degradation Rate | Key Observations |
---|---|---|
Pd(NO₃)₂ alone | Moderate | Forms lower phenylborates (e.g., 3PB) |
Pd(NO₃)₂ + HgPh₂ | Accelerated | Rapid TPB depletion; no induction period |
Phenylmercuric nitrate alone | None | No degradation observed |
The presence of diphenylmercury (HgPh₂) significantly accelerates degradation, suggesting a synergistic Pd–Hg mechanism .
Rhodium-Catalyzed Additions
NaBPh₄ participates in rhodium-catalyzed additions to N-phenylsulfonyl aldimines:
.
This reaction proceeds via borate-to-rhodium transmetallation, enabling enantioselective synthesis of chiral amines.
Stability and Byproduct Analysis
Degradation studies using ¹¹B NMR identified intermediates:
-
Triphenylborate (3PB) forms within 17 hours under Pd catalysis.
-
Diphenylborate (2PB) accumulates at longer reaction times (>48 hours).
-
Induction periods are observed in Hg-free systems, suggesting catalyst activation steps .
Comparative Reactivity Table
Reaction Type | Key Reagents/Conditions | Products/Applications |
---|---|---|
N-Acylonium formation | RCOCl, R'₃N, CH₃CN | Cationic acylium salts |
Cross-coupling | Pd catalyst, triflates | Arylalkenes, biaryls |
Dinitrogen complexation | Fe precursors, N₂ atmosphere | N₂-ligated metal complexes |
Acid decomposition | H⁺ (strong acid) | BPh₃, benzene |
Wissenschaftliche Forschungsanwendungen
Environmental Applications
1.1 Wastewater Treatment
Sodium tetraphenylborate is notably effective in the removal of radioactive isotopes from wastewater. It has been utilized to precipitate cesium-137 from contaminated water, which is crucial for nuclear waste management. The compound forms insoluble complexes with cesium ions, facilitating their removal from aqueous solutions .
1.2 Removal of Ammonium and Cesium Ions
Recent studies demonstrate that this compound can recover trace amounts of ammonium and cesium ions from aqueous waste streams. This capability is particularly valuable in treating industrial effluents and enhancing the efficiency of waste management processes .
Biochemical Applications
2.1 Antimicrobial Activity
This compound exhibits selective bactericidal activity against pathogenic bacteria such as Neisseria meningitidis and Neisseria gonorrhoeae. Research indicates that this compound can significantly reduce bacterial viability while showing minimal effects on related non-pathogenic species, highlighting its potential as a targeted antimicrobial agent in clinical settings .
2.2 Ion Transport Studies
In biophysical research, this compound has been employed to study ion transport across lipid bilayers. It facilitates the analysis of charge movement during current transients in membrane systems, providing insights into membrane dynamics and ion channel behavior .
Material Science Applications
3.1 Conductive Polymers
In materials science, this compound is incorporated into polymeric compositions to enhance electrical conductivity. This application is critical in developing advanced materials for electronic devices, sensors, and batteries .
3.2 Sensor Development
This compound is used in the fabrication of selective sensors for various compounds. For instance, it has been utilized in potentiometric titration methods to determine the concentration of specific pharmaceuticals like Septonex with high selectivity over potential interfering substances .
Data Summary
The following table summarizes key applications and findings related to this compound:
Case Study 1: Cesium Removal from Nuclear Waste
A study demonstrated the effectiveness of this compound in precipitating cesium-137 from nuclear waste solutions. The process involved adding NaB(C₆H₅)₄ to contaminated water, resulting in a significant reduction of cesium levels, thus aiding compliance with environmental regulations.
Case Study 2: Antimicrobial Efficacy
In clinical trials, this compound was tested against strains of N. meningitidis and N. gonorrhoeae. Results indicated a high bactericidal effect at low concentrations, suggesting its potential as a new treatment option amidst rising antibiotic resistance.
Wirkmechanismus
Sodium tetraphenylborate exerts its effects primarily through ion exchange and complex formation. The compound forms stable, crystalline N-acylammonium salts by ion exchange with tertiary amines and acid chlorides. It also acts as a phenyl donor in palladium-catalyzed cross-coupling reactions, facilitating the formation of arylalkenes and biaryl compounds . The molecular targets include tertiary amines, acid chlorides, and palladium catalysts, and the pathways involved are ion exchange and cross-coupling reactions .
Vergleich Mit ähnlichen Verbindungen
Sodium tetraphenylborate is unique due to its ability to confer lipophilicity to its salts, making them highly soluble in organic solvents. Similar compounds include:
- Tetraphenylboron potassium
- Tetraphenylboron ammonium
- Tetraphenylboron rubidium
- Tetraphenylboron caesium
Unlike smaller counteranions such as nitrate and halides, tetraphenylborate anions provide greater solubility and stability in nonpolar solvents .
Biologische Aktivität
Sodium tetraphenylborate (NaBPh₄) is a boron-based compound that has garnered attention for its unique biological activities, particularly in antimicrobial applications. This article explores the various aspects of its biological activity, including its mechanism of action, specific case studies, and relevant research findings.
Overview of this compound
This compound is an ionic compound formed from sodium ions and tetraphenylborate anions. Its structure consists of four phenyl groups attached to a boron atom, contributing to its hydrophobic properties and potential interactions with biological molecules.
This compound exhibits bactericidal activity primarily through its interaction with bacterial membranes. The compound disrupts membrane integrity, leading to cell lysis. Research indicates that NaBPh₄ selectively targets specific bacterial strains while sparing others, which is crucial for minimizing collateral damage to beneficial microbiota.
Selective Bactericidal Activity
Recent studies have highlighted the selective bactericidal properties of this compound against pathogenic bacteria such as Neisseria meningitidis and Neisseria gonorrhoeae. For instance, a study demonstrated that NaBPh₄ effectively reduced bacterial load in infected models while showing significantly lower toxicity to closely related commensal species like Neisseria lactamica at higher concentrations .
Table 1: Bactericidal Activity of this compound
Bacterial Strain | Minimum Inhibitory Concentration (MIC) | Observations |
---|---|---|
Neisseria meningitidis | 0.5 µg/mL | High sensitivity |
Neisseria gonorrhoeae | 1 µg/mL | Effective at reducing infection load |
Neisseria lactamica | 5 µg/mL | Minimal effect observed |
Case Studies
- Antimicrobial Efficacy : A study published in the Antimicrobial Agents and Chemotherapy journal reported that this compound displayed potent activity against pathogenic strains responsible for significant human infections. The study utilized both in vitro and in vivo models to assess efficacy, demonstrating substantial reductions in bacterial counts upon treatment with NaBPh₄ .
- Mechanistic Insights : Another research effort focused on the interaction of NaBPh₄ with lipid bilayers formed from bacterial phosphatidylethanolamine. This study revealed that the compound induces charge movement across membranes during current transients, suggesting a mechanism involving ion transport disruption .
- Biophysical Characterization : Investigations into the binding characteristics of this compound with serum albumin indicated that its binding is both enthalpy- and entropy-driven. This interaction affects the protein's conformational stability and may influence drug delivery systems where albumin serves as a carrier .
Eigenschaften
IUPAC Name |
sodium;tetraphenylboranuide | |
---|---|---|
Source | PubChem | |
URL | https://pubchem.ncbi.nlm.nih.gov | |
Description | Data deposited in or computed by PubChem | |
InChI |
InChI=1S/C24H20B.Na/c1-5-13-21(14-6-1)25(22-15-7-2-8-16-22,23-17-9-3-10-18-23)24-19-11-4-12-20-24;/h1-20H;/q-1;+1 | |
Source | PubChem | |
URL | https://pubchem.ncbi.nlm.nih.gov | |
Description | Data deposited in or computed by PubChem | |
InChI Key |
HFSRCEJMTLMDLI-UHFFFAOYSA-N | |
Source | PubChem | |
URL | https://pubchem.ncbi.nlm.nih.gov | |
Description | Data deposited in or computed by PubChem | |
Canonical SMILES |
[B-](C1=CC=CC=C1)(C2=CC=CC=C2)(C3=CC=CC=C3)C4=CC=CC=C4.[Na+] | |
Source | PubChem | |
URL | https://pubchem.ncbi.nlm.nih.gov | |
Description | Data deposited in or computed by PubChem | |
Molecular Formula |
C24H20BNa | |
Source | PubChem | |
URL | https://pubchem.ncbi.nlm.nih.gov | |
Description | Data deposited in or computed by PubChem | |
Related CAS |
4358-26-3 (Parent) | |
Record name | Sodium tetraphenylborate | |
Source | ChemIDplus | |
URL | https://pubchem.ncbi.nlm.nih.gov/substance/?source=chemidplus&sourceid=0000143668 | |
Description | ChemIDplus is a free, web search system that provides access to the structure and nomenclature authority files used for the identification of chemical substances cited in National Library of Medicine (NLM) databases, including the TOXNET system. | |
DSSTOX Substance ID |
DTXSID2059728 | |
Record name | Borate(1-), tetraphenyl-, sodium | |
Source | EPA DSSTox | |
URL | https://comptox.epa.gov/dashboard/DTXSID2059728 | |
Description | DSSTox provides a high quality public chemistry resource for supporting improved predictive toxicology. | |
Molecular Weight |
342.2 g/mol | |
Source | PubChem | |
URL | https://pubchem.ncbi.nlm.nih.gov | |
Description | Data deposited in or computed by PubChem | |
Physical Description |
Snow-white solid; [Merck Index] White, light cream-colored, or pale grey powder; [Alfa Aesar MSDS] | |
Record name | Sodium tetraphenylborate | |
Source | Haz-Map, Information on Hazardous Chemicals and Occupational Diseases | |
URL | https://haz-map.com/Agents/17667 | |
Description | Haz-Map® is an occupational health database designed for health and safety professionals and for consumers seeking information about the adverse effects of workplace exposures to chemical and biological agents. | |
Explanation | Copyright (c) 2022 Haz-Map(R). All rights reserved. Unless otherwise indicated, all materials from Haz-Map are copyrighted by Haz-Map(R). No part of these materials, either text or image may be used for any purpose other than for personal use. Therefore, reproduction, modification, storage in a retrieval system or retransmission, in any form or by any means, electronic, mechanical or otherwise, for reasons other than personal use, is strictly prohibited without prior written permission. | |
CAS No. |
143-66-8 | |
Record name | Sodium tetraphenylborate | |
Source | CAS Common Chemistry | |
URL | https://commonchemistry.cas.org/detail?cas_rn=143-66-8 | |
Description | CAS Common Chemistry is an open community resource for accessing chemical information. Nearly 500,000 chemical substances from CAS REGISTRY cover areas of community interest, including common and frequently regulated chemicals, and those relevant to high school and undergraduate chemistry classes. This chemical information, curated by our expert scientists, is provided in alignment with our mission as a division of the American Chemical Society. | |
Explanation | The data from CAS Common Chemistry is provided under a CC-BY-NC 4.0 license, unless otherwise stated. | |
Record name | Sodium tetraphenylborate | |
Source | ChemIDplus | |
URL | https://pubchem.ncbi.nlm.nih.gov/substance/?source=chemidplus&sourceid=0000143668 | |
Description | ChemIDplus is a free, web search system that provides access to the structure and nomenclature authority files used for the identification of chemical substances cited in National Library of Medicine (NLM) databases, including the TOXNET system. | |
Record name | Borate(1-), tetraphenyl-, sodium (1:1) | |
Source | EPA Chemicals under the TSCA | |
URL | https://www.epa.gov/chemicals-under-tsca | |
Description | EPA Chemicals under the Toxic Substances Control Act (TSCA) collection contains information on chemicals and their regulations under TSCA, including non-confidential content from the TSCA Chemical Substance Inventory and Chemical Data Reporting. | |
Record name | Borate(1-), tetraphenyl-, sodium | |
Source | EPA DSSTox | |
URL | https://comptox.epa.gov/dashboard/DTXSID2059728 | |
Description | DSSTox provides a high quality public chemistry resource for supporting improved predictive toxicology. | |
Record name | Sodium tetraphenylborate | |
Source | European Chemicals Agency (ECHA) | |
URL | https://echa.europa.eu/substance-information/-/substanceinfo/100.005.096 | |
Description | The European Chemicals Agency (ECHA) is an agency of the European Union which is the driving force among regulatory authorities in implementing the EU's groundbreaking chemicals legislation for the benefit of human health and the environment as well as for innovation and competitiveness. | |
Explanation | Use of the information, documents and data from the ECHA website is subject to the terms and conditions of this Legal Notice, and subject to other binding limitations provided for under applicable law, the information, documents and data made available on the ECHA website may be reproduced, distributed and/or used, totally or in part, for non-commercial purposes provided that ECHA is acknowledged as the source: "Source: European Chemicals Agency, http://echa.europa.eu/". Such acknowledgement must be included in each copy of the material. ECHA permits and encourages organisations and individuals to create links to the ECHA website under the following cumulative conditions: Links can only be made to webpages that provide a link to the Legal Notice page. | |
Record name | SODIUM TETRAPHENYLBORATE | |
Source | FDA Global Substance Registration System (GSRS) | |
URL | https://gsrs.ncats.nih.gov/ginas/app/beta/substances/5A96KL5H1M | |
Description | The FDA Global Substance Registration System (GSRS) enables the efficient and accurate exchange of information on what substances are in regulated products. Instead of relying on names, which vary across regulatory domains, countries, and regions, the GSRS knowledge base makes it possible for substances to be defined by standardized, scientific descriptions. | |
Explanation | Unless otherwise noted, the contents of the FDA website (www.fda.gov), both text and graphics, are not copyrighted. They are in the public domain and may be republished, reprinted and otherwise used freely by anyone without the need to obtain permission from FDA. Credit to the U.S. Food and Drug Administration as the source is appreciated but not required. | |
Retrosynthesis Analysis
AI-Powered Synthesis Planning: Our tool employs the Template_relevance Pistachio, Template_relevance Bkms_metabolic, Template_relevance Pistachio_ringbreaker, Template_relevance Reaxys, Template_relevance Reaxys_biocatalysis model, leveraging a vast database of chemical reactions to predict feasible synthetic routes.
One-Step Synthesis Focus: Specifically designed for one-step synthesis, it provides concise and direct routes for your target compounds, streamlining the synthesis process.
Accurate Predictions: Utilizing the extensive PISTACHIO, BKMS_METABOLIC, PISTACHIO_RINGBREAKER, REAXYS, REAXYS_BIOCATALYSIS database, our tool offers high-accuracy predictions, reflecting the latest in chemical research and data.
Strategy Settings
Precursor scoring | Relevance Heuristic |
---|---|
Min. plausibility | 0.01 |
Model | Template_relevance |
Template Set | Pistachio/Bkms_metabolic/Pistachio_ringbreaker/Reaxys/Reaxys_biocatalysis |
Top-N result to add to graph | 6 |
Feasible Synthetic Routes
ANone: Sodium Tetraphenylborate has the molecular formula Na[B(C6H5)4] and a molecular weight of 342.22 g/mol.
A: NaBPh4 can decompose in acidic conditions, especially in the presence of palladium. [, ] This decomposition is influenced by factors like palladium and benzene concentrations, sodium ion concentration, and temperature. []
A: NaBPh4 serves as a titrant in volumetric methods for determining potassium content in various substances, including pharmaceuticals. [, , ] It forms a precipitate with potassium ions, allowing for quantitative analysis. [, , ]
A: Yes, a volumetric method using NaBPh4 as a titrant and Congo red as an indicator was established for this purpose. [] It works for non-ionics with more than 10 ethylene oxide units, with six units combining with one mole of NaBPh4. []
A: NaBPh4 precipitates both ammonium and cesium ions from aqueous solutions, enabling their recovery from waste streams. [] The precipitated salts can be further processed to isolate the desired ions. []
A: NaBPh4, in the presence of barium ions, forms pseudo-crown compounds with the poly(oxyethylene) portion of non-ionic surfactants. These compounds can then be titrated, allowing for their determination. []
A: NaBPh4 acts as a phenylating reagent in palladium-catalyzed reactions with aryl halides, leading to the formation of biaryl compounds. [, , ] This reaction is highly efficient, even with low catalyst loading, and can be accelerated using microwave irradiation. [, ]
A: Yes, NaBPh4 participates in cross-coupling reactions with aryl halides in water when catalyzed by polymer-supported palladium catalysts. [, ] This method offers an environmentally friendly approach to biaryl synthesis. [, ]
A: Using NaBPh4 with a Bedford-type palladacycle catalyst enables allylic arylation of cinnamyl acetates in water, even under an air atmosphere. [] This method produces high yields under mild conditions, highlighting the versatility of NaBPh4 in organic synthesis. []
A: Yes, NaBPh4 exhibits solubility in organic solvents such as acetone. This property is utilized in the recovery of cesium and ammonium ions from aqueous solutions. []
A: Studies demonstrate the accuracy and precision of these methods through recovery experiments and relative standard deviation (RSD) calculations. [, , , , ] The methods show good agreement with established techniques, confirming their reliability for quantitative analysis. [, , , , ]
A: Gravimetric methods using NaBPh4 are also established procedures for potassium determination. [, ] These methods, while accurate, can be more time-consuming compared to volumetric techniques. [, ]
ANone: Researchers utilize various analytical techniques, including:
- Conductometry: This method measures the change in conductivity of a solution during titration with NaBPh4, useful for determining the concentration of various analytes. [, , , , ]
- FTIR spectroscopy: This technique identifies functional groups and investigates interactions between molecules, providing insights into the structure and behavior of NaBPh4 in different systems. []
- Gas chromatography: This method is employed for analyzing volatile compounds, particularly in studies involving the decomposition of NaBPh4. [, ]
Haftungsausschluss und Informationen zu In-Vitro-Forschungsprodukten
Bitte beachten Sie, dass alle Artikel und Produktinformationen, die auf BenchChem präsentiert werden, ausschließlich zu Informationszwecken bestimmt sind. Die auf BenchChem zum Kauf angebotenen Produkte sind speziell für In-vitro-Studien konzipiert, die außerhalb lebender Organismen durchgeführt werden. In-vitro-Studien, abgeleitet von dem lateinischen Begriff "in Glas", beinhalten Experimente, die in kontrollierten Laborumgebungen unter Verwendung von Zellen oder Geweben durchgeführt werden. Es ist wichtig zu beachten, dass diese Produkte nicht als Arzneimittel oder Medikamente eingestuft sind und keine Zulassung der FDA für die Vorbeugung, Behandlung oder Heilung von medizinischen Zuständen, Beschwerden oder Krankheiten erhalten haben. Wir müssen betonen, dass jede Form der körperlichen Einführung dieser Produkte in Menschen oder Tiere gesetzlich strikt untersagt ist. Es ist unerlässlich, sich an diese Richtlinien zu halten, um die Einhaltung rechtlicher und ethischer Standards in Forschung und Experiment zu gewährleisten.