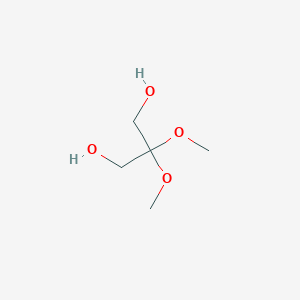
2,2-Dimethoxypropane-1,3-diol
Übersicht
Beschreibung
“2,2-Dimethoxypropane-1,3-diol” is an organic compound with the molecular formula C5H12O4 . It has a molecular weight of 136.15 g/mol . The compound is also known by several synonyms, including “2,2-dimethoxypropane-1,3-diol”, “153214-82-5”, “2,2-dimethoxy-propane-1,3-diol”, “MFCD09878500”, and "2,2-dimethoxy-1,3-propanediol" .
Synthesis Analysis
The synthesis of “2,2-Dimethoxypropane-1,3-diol” involves the use of various reagents such as propan-2-one, 2,2-dimethoxypropane, and 2-methoxypropene . These reagents are commonly used for the preparation of isopropylidene ketals . The reaction scope, selectivity, and catalyst loading are critical issues that have been thoroughly optimized .
Molecular Structure Analysis
The molecular structure of “2,2-Dimethoxypropane-1,3-diol” is represented by the InChI code InChI=1S/C5H12O4/c1-8-5 (3-6,4-7)9-2/h6-7H,3-4H2,1-2H3
. The compound has a topological polar surface area of 58.9 Ų and a complexity of 63.4 . It has a total of 9 heavy atoms .
Chemical Reactions Analysis
“2,2-Dimethoxypropane-1,3-diol” is known to undergo various chemical reactions. For instance, it reacts with water to produce methanol and acetone . This reaction has been employed in a method for the quantification of water in natural products by gas-liquid chromatography .
Physical And Chemical Properties Analysis
“2,2-Dimethoxypropane-1,3-diol” has a molecular weight of 136.15 g/mol . It has a hydrogen bond donor count of 2 and a hydrogen bond acceptor count of 4 . The compound has a rotatable bond count of 4 . Its exact mass and monoisotopic mass are both 136.07355886 g/mol .
Wissenschaftliche Forschungsanwendungen
Synthesis of Aliphatic Polycarbonate
The compound is used in the synthesis of aliphatic polycarbonate based on dihydroxyacetone . The six-membered cyclic carbonate monomer, 2,2-dimethoxy-1,3-propanediol carbonate, is prepared by a two-step reaction including protection and ring-closing, starting from dihydroxyacetone .
Ring-Opening Polymerization
The ring-opening polymerization of 2,2-dimethoxy-1,3-propanediol carbonate is carried out in bulk at 110–140°C initiated by stannous octanoate to give polycarbonate . The effects of different reaction conditions including different catalyst, reaction temperature, molar ratio of monomer to initiator and polymerization time on the polymerization were investigated .
Hydrolytic Degradation
The protecting ketal group is partly removed by hydrolysis using 50% trifluroacetic acid as a catalyst to give a functional polycarbonate containing 70% ketone carbonyl group, which improved the hydrophilicity of initial polycarbonate .
Water Scavenger
2,2-Dimethoxypropane-1,3-diol is used as a water scavenger in water-sensitive reactions . Upon acid-catalyzed reaction, it reacts quantitatively with water to form acetone and methanol .
Quantification of Water
This property can be used to accurately determine the amount of water in a sample, alternatively to the Karl Fischer method .
Preparation of Acetonides
2,2-Dimethoxypropane-1,3-diol is specifically used to prepare acetonides .
Protection of Carbonyl Groups
It is used for protection of carbonyl groups as 4,4-dimethyl-1,3-dioxanes, often preferred to the more usual 1,3-dioxolanes due to easier separation and greater stability .
Dehydration of Biological Samples
Acidified 2,2-Dimethoxypropane-1,3-diol has been employed for the dehydration of biological samples .
Wirkmechanismus
Target of Action
The primary target of 2,2-Dimethoxypropane-1,3-diol is water in water-sensitive reactions . It acts as a water scavenger, reacting with water to form acetone and methanol .
Mode of Action
2,2-Dimethoxypropane-1,3-diol interacts with its target (water) through an acid-catalyzed reaction . This reaction is quantitative, meaning it proceeds until one of the reactants (in this case, water or 2,2-Dimethoxypropane-1,3-diol) is completely consumed .
Biochemical Pathways
The primary biochemical pathway affected by 2,2-Dimethoxypropane-1,3-diol is the formation of acetone and methanol from water . This reaction can be used to accurately determine the amount of water in a sample, providing an alternative to the Karl Fischer method . Additionally, 2,2-Dimethoxypropane-1,3-diol is used to prepare acetonides .
Result of Action
The result of the action of 2,2-Dimethoxypropane-1,3-diol is the formation of acetone and methanol . This reaction is used in various applications, including the preparation of acetonides and the dehydration of animal tissue in histology .
Action Environment
The action of 2,2-Dimethoxypropane-1,3-diol is influenced by the presence of water, as it is used as a water scavenger in water-sensitive reactions . Therefore, the compound’s action, efficacy, and stability are likely to be influenced by environmental factors that affect the availability of water.
Safety and Hazards
Eigenschaften
IUPAC Name |
2,2-dimethoxypropane-1,3-diol | |
---|---|---|
Source | PubChem | |
URL | https://pubchem.ncbi.nlm.nih.gov | |
Description | Data deposited in or computed by PubChem | |
InChI |
InChI=1S/C5H12O4/c1-8-5(3-6,4-7)9-2/h6-7H,3-4H2,1-2H3 | |
Source | PubChem | |
URL | https://pubchem.ncbi.nlm.nih.gov | |
Description | Data deposited in or computed by PubChem | |
InChI Key |
HYRZFKCRTXTLLZ-UHFFFAOYSA-N | |
Source | PubChem | |
URL | https://pubchem.ncbi.nlm.nih.gov | |
Description | Data deposited in or computed by PubChem | |
Canonical SMILES |
COC(CO)(CO)OC | |
Source | PubChem | |
URL | https://pubchem.ncbi.nlm.nih.gov | |
Description | Data deposited in or computed by PubChem | |
Molecular Formula |
C5H12O4 | |
Source | PubChem | |
URL | https://pubchem.ncbi.nlm.nih.gov | |
Description | Data deposited in or computed by PubChem | |
DSSTOX Substance ID |
DTXSID50456058 | |
Record name | 2,2-dimethoxypropane-1,3-diol | |
Source | EPA DSSTox | |
URL | https://comptox.epa.gov/dashboard/DTXSID50456058 | |
Description | DSSTox provides a high quality public chemistry resource for supporting improved predictive toxicology. | |
Molecular Weight |
136.15 g/mol | |
Source | PubChem | |
URL | https://pubchem.ncbi.nlm.nih.gov | |
Description | Data deposited in or computed by PubChem | |
Product Name |
2,2-Dimethoxypropane-1,3-diol | |
CAS RN |
153214-82-5 | |
Record name | 2,2-dimethoxypropane-1,3-diol | |
Source | EPA DSSTox | |
URL | https://comptox.epa.gov/dashboard/DTXSID50456058 | |
Description | DSSTox provides a high quality public chemistry resource for supporting improved predictive toxicology. | |
Synthesis routes and methods
Procedure details
Retrosynthesis Analysis
AI-Powered Synthesis Planning: Our tool employs the Template_relevance Pistachio, Template_relevance Bkms_metabolic, Template_relevance Pistachio_ringbreaker, Template_relevance Reaxys, Template_relevance Reaxys_biocatalysis model, leveraging a vast database of chemical reactions to predict feasible synthetic routes.
One-Step Synthesis Focus: Specifically designed for one-step synthesis, it provides concise and direct routes for your target compounds, streamlining the synthesis process.
Accurate Predictions: Utilizing the extensive PISTACHIO, BKMS_METABOLIC, PISTACHIO_RINGBREAKER, REAXYS, REAXYS_BIOCATALYSIS database, our tool offers high-accuracy predictions, reflecting the latest in chemical research and data.
Strategy Settings
Precursor scoring | Relevance Heuristic |
---|---|
Min. plausibility | 0.01 |
Model | Template_relevance |
Template Set | Pistachio/Bkms_metabolic/Pistachio_ringbreaker/Reaxys/Reaxys_biocatalysis |
Top-N result to add to graph | 6 |
Feasible Synthetic Routes
Q & A
Q1: What is the significance of 2,2-Dimethoxypropane-1,3-diol in the synthesis of Dihydroxyacetone phosphate (DHAP)?
A1: 2,2-Dimethoxypropane-1,3-diol serves as a key intermediate in a novel synthesis pathway for Dihydroxyacetone phosphate (DHAP) []. This pathway starts with the conversion of dihydroxyacetone dimer (DHA) into 2,2-Dimethoxypropane-1,3-diol. This compound then undergoes enzymatic desymmetrization by acetylation using lipase AK. This step is crucial for introducing chirality into the molecule, a necessary feature for DHAP. Subsequent phosphorylation and deprotection steps ultimately yield DHAP in high purity and yield [].
Q2: How is 2,2-Dimethoxypropane-1,3-diol used in macromolecular engineering?
A2: 2,2-Dimethoxypropane-1,3-diol serves as a precursor to its corresponding cyclic carbonate monomer, 2,2-dimethoxypropane-1,3-diol carbonate (TMC(OMe)2) []. This monomer is valuable in ring-opening polymerization (ROP) reactions, particularly for creating block copolymers with tunable properties []. Researchers have successfully incorporated TMC(OMe)2 into copolymers alongside other monomers like trimethylene carbonate (TMC) []. This strategy allows for the fine-tuning of the thermal and mechanical characteristics of the resulting polymers, offering a platform for developing materials with tailored properties [].
Q3: Can you elaborate on the “immortal” ring-opening polymerization technique mentioned in relation to 2,2-Dimethoxypropane-1,3-diol?
A3: "Immortal" ring-opening polymerization (iROP) is characterized by the use of specific catalytic systems that enable precise control over the polymerization process []. In the context of 2,2-Dimethoxypropane-1,3-diol-derived monomers like TMC(OMe)2, iROP, facilitated by catalysts such as [(BDIiPr)Zn(N(SiMe3)2)] or BEMP, allows for the creation of well-defined block copolymers []. This controlled polymerization is vital for tailoring the monomer sequence and ultimately dictating the final properties of the resulting copolymer materials [].
Haftungsausschluss und Informationen zu In-Vitro-Forschungsprodukten
Bitte beachten Sie, dass alle Artikel und Produktinformationen, die auf BenchChem präsentiert werden, ausschließlich zu Informationszwecken bestimmt sind. Die auf BenchChem zum Kauf angebotenen Produkte sind speziell für In-vitro-Studien konzipiert, die außerhalb lebender Organismen durchgeführt werden. In-vitro-Studien, abgeleitet von dem lateinischen Begriff "in Glas", beinhalten Experimente, die in kontrollierten Laborumgebungen unter Verwendung von Zellen oder Geweben durchgeführt werden. Es ist wichtig zu beachten, dass diese Produkte nicht als Arzneimittel oder Medikamente eingestuft sind und keine Zulassung der FDA für die Vorbeugung, Behandlung oder Heilung von medizinischen Zuständen, Beschwerden oder Krankheiten erhalten haben. Wir müssen betonen, dass jede Form der körperlichen Einführung dieser Produkte in Menschen oder Tiere gesetzlich strikt untersagt ist. Es ist unerlässlich, sich an diese Richtlinien zu halten, um die Einhaltung rechtlicher und ethischer Standards in Forschung und Experiment zu gewährleisten.