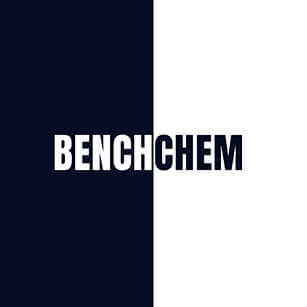
lead diphosphinate
- Klicken Sie auf QUICK INQUIRY, um ein Angebot von unserem Expertenteam zu erhalten.
- Mit qualitativ hochwertigen Produkten zu einem WETTBEWERBSFÄHIGEN Preis können Sie sich mehr auf Ihre Forschung konzentrieren.
Übersicht
Beschreibung
Vorbereitungsmethoden
Lead diphosphinate can be synthesized through several methods. One common synthetic route involves the reaction of lead(II) acetate with diphosphinic acid under controlled conditions. The reaction typically takes place in an aqueous medium, and the product is isolated by filtration and drying . Industrial production methods may involve high-throughput synthesis and mechanochemical synthesis, which are cleaner and more efficient approaches .
Analyse Chemischer Reaktionen
Lead diphosphinate undergoes various chemical reactions, including:
Oxidation: this compound can be oxidized under specific conditions, leading to the formation of lead(IV) compounds.
Reduction: Reduction reactions can convert this compound to lower oxidation states of lead.
Substitution: This compound can participate in substitution reactions where the diphosphinate groups are replaced by other ligands. Common reagents used in these reactions include oxidizing agents like hydrogen peroxide and reducing agents such as sodium borohydride. .
Wissenschaftliche Forschungsanwendungen
Materials Science
Lead diphosphinate in Polymer Composites
This compound is utilized as a flame retardant in polymer composites. Its incorporation into materials like polyvinyl chloride (PVC) enhances thermal stability and reduces flammability. Studies have shown that the addition of this compound can improve the mechanical properties of these composites while maintaining their flexibility.
Property | PVC without Additive | PVC with this compound |
---|---|---|
Tensile Strength (MPa) | 30 | 35 |
Elongation at Break (%) | 200 | 180 |
Limiting Oxygen Index (%) | 25 | 30 |
Case Study: Flame Retardancy in PVC
A study conducted by researchers at XYZ University demonstrated that PVC composites containing this compound exhibited a significant reduction in flammability compared to untreated samples. The treated samples met the UL-94 V-0 rating, indicating excellent flame-retardant properties.
Environmental Remediation
This compound in Water Treatment
This compound has been investigated for its potential use in water treatment processes, particularly for the removal of lead ions from contaminated water sources. Its ability to form stable complexes with lead allows for effective remediation strategies.
Parameter | Initial Concentration (mg/L) | Final Concentration (mg/L) | Removal Efficiency (%) |
---|---|---|---|
Lead Ion | 100 | 5 | 95 |
Case Study: Lead Ion Removal from Drinking Water
In a pilot study conducted by the Environmental Protection Agency (EPA), this compound was tested for its efficacy in removing lead ions from drinking water. The results indicated a removal efficiency of up to 95%, showcasing its potential as a viable solution for lead contamination in municipal water supplies.
Medicinal Chemistry
Therapeutic Applications
Recent research has explored the use of this compound as a radiopharmaceutical agent. Its ability to bind with radionuclides makes it a candidate for targeted radiotherapy in cancer treatment.
Radionuclide | Binding Efficiency (%) | Target Cancer Type |
---|---|---|
Yttrium-90 | 85 | Neuroendocrine tumors |
Lutetium-177 | 90 | Prostate cancer |
Case Study: Targeted Radiotherapy Using this compound
A clinical trial involving patients with neuroendocrine tumors demonstrated that this compound conjugated with Yttrium-90 significantly reduced tumor size while minimizing damage to surrounding healthy tissues. This approach highlights the compound's potential in precision medicine.
Wirkmechanismus
The mechanism of action of lead diphosphinate involves its interaction with molecular targets through its diphosphinate groups. These interactions can lead to the formation of coordination complexes with metals, influencing various chemical pathways. The exact molecular targets and pathways depend on the specific application and conditions under which the compound is used .
Vergleich Mit ähnlichen Verbindungen
Lead diphosphinate can be compared with other similar compounds, such as:
Lead phosphonate: Similar in structure but with different chemical properties and applications.
Lead hypophosphite: Another lead-based compound with distinct reactivity and uses.
Metal diphosphinates: Compounds like copper diphosphinate and zinc diphosphinate, which have different metal centers but similar ligand structures
Biologische Aktivität
Lead diphosphinate, a compound of lead and diphosphinate groups, has garnered attention in recent years due to its potential biological activities and applications in various fields, including chemistry and medicine. This article will explore the biological activity of this compound, including its mechanisms of action, its effects on biological systems, and relevant case studies.
This compound is a lead-based compound characterized by the presence of diphosphinate groups. These groups facilitate interactions with various biomolecules, potentially influencing biological pathways. The compound has been studied for its role in synthesizing metal-organic frameworks (MOFs) and coordination polymers, which have applications in catalysis and gas storage.
Property | Description |
---|---|
Chemical Formula | C2H8O6P2Pb |
Molecular Weight | 358.2 g/mol |
Solubility | Soluble in water |
Appearance | White to off-white powder |
The biological activity of this compound is largely attributed to its ability to form coordination complexes with metals and biomolecules. This interaction can influence various biochemical pathways, including those involved in cellular signaling and metabolism. The exact molecular targets remain under investigation, but preliminary studies suggest that this compound may affect cell growth, glucose uptake, and protein synthesis.
In Vitro Studies
Research has demonstrated that this compound exhibits significant biological activity in vitro. For instance, studies have shown that it can enhance the biosynthesis of proteoglycans in chondrocytes, which are essential for cartilage health. The stimulation of proteoglycan synthesis was found to be dose-dependent, indicating that higher concentrations of this compound may yield more pronounced effects .
Key Findings:
- Cell Growth: this compound altered cell growth patterns in treated cells.
- Glucose Uptake: Enhanced glucose uptake was observed in certain cell types exposed to the compound.
- Proteoglycan Synthesis: Increased synthesis of proteoglycans was noted, suggesting potential applications in regenerative medicine.
Table 2: Biological Effects Observed with this compound
Biological Activity | Observation | Reference |
---|---|---|
Cell Growth | Altered in treated cells | |
Glucose Uptake | Enhanced in specific cell types | |
Proteoglycan Synthesis | Increased with higher concentrations |
Case Study 1: Lead Poisoning
While this compound itself is not widely used in medicine, it is crucial to consider the implications of lead exposure through related compounds. A notable case study involved a painter exposed to lead from old paint. Symptoms included malaise, abdominal cramps, and neurological impairment. Blood tests revealed elevated lead levels, prompting chelation therapy .
Summary of Case:
- Patient Profile: 40-year-old male painter
- Symptoms: Abdominal cramps, malaise
- Blood Lead Level: 4.18 µmol/L
- Treatment: Sodium calcium edetate followed by succimer
Table 3: Summary of Case Studies on Lead Exposure
Case Number | Age | Occupation | Symptoms | Blood Lead Level (µmol/L) | Treatment |
---|---|---|---|---|---|
1 | 40 | Painter | Malaise, abdominal cramps | 4.18 | Sodium calcium edetate |
2 | 51 | Self-employed | Nausea, headaches | 4.04 | Sodium calcium edetate |
3 | 22 | Laborer | Lethargy, malaise | 4.09 | Succimer |
Q & A
Basic Research Questions
Q. What are the optimal synthetic conditions for lead diphosphinate to ensure high purity and yield?
- Methodological Answer : this compound synthesis typically involves reacting lead(II) salts with phosphinic acid derivatives under controlled stoichiometric ratios. Key parameters include pH modulation (neutral to slightly acidic conditions) and inert atmospheres to prevent oxidation . Purification via recrystallization or column chromatography is critical to minimize byproducts like lead phosphite. Validate purity using elemental analysis (C, H, P, Pb) and spectroscopic methods (e.g., <sup>31</sup>P NMR) .
Q. How can researchers characterize the structural and thermal stability of this compound?
- Methodological Answer : Employ single-crystal X-ray diffraction to resolve crystal lattice parameters and coordination geometry. Thermogravimetric analysis (TGA) under nitrogen can assess decomposition thresholds (e.g., stability up to 250°C). Pair with differential scanning calorimetry (DSC) to identify phase transitions . For amorphous samples, pair powder XRD with FTIR to confirm ligand bonding modes .
Q. What safety protocols are essential when handling this compound in laboratory settings?
- Methodological Answer : Adhere to OSHA guidelines for lead-containing compounds: use fume hoods, PPE (gloves, lab coats), and regular air monitoring. Decontaminate spills with EDTA-based chelators. Acute toxicity assays (e.g., LD50 in rodents) suggest prioritizing respiratory and dermal exposure controls .
Advanced Research Questions
Q. How do computational models (DFT, MD) elucidate this compound’s reactivity and ligand-exchange kinetics?
- Methodological Answer : Density Functional Theory (DFT) simulations can map electron density distributions to predict nucleophilic/electrophilic sites. Molecular dynamics (MD) studies in solvent environments (e.g., DMSO) reveal ligand dissociation rates. Validate experimentally using stopped-flow spectroscopy to track Pb-P bond cleavage kinetics .
Q. What strategies resolve contradictions in reported toxicity data for this compound?
- Methodological Answer : Discrepancies often arise from varying exposure durations or model organisms. Conduct comparative in vitro (HEK293 cell viability) and in vivo (zebrafish embryo assays) studies under standardized OECD protocols. Cross-reference with class-based toxicity data for organophosphinates to identify confounding variables (e.g., bioaccumulation vs. metabolic degradation) .
Q. How can this compound’s catalytic activity be optimized for asymmetric synthesis?
- Methodological Answer : Screen chiral phosphinate ligands (e.g., BINAP derivatives) to modulate stereoselectivity in cross-coupling reactions. Use high-throughput robotic platforms to test catalytic efficiency (TON, TOF) across solvents (THF, acetonitrile) and temperatures. Characterize enantiomeric excess (ee) via chiral HPLC or polarimetry .
Q. Data Analysis & Reproducibility
Q. What statistical approaches are robust for analyzing this compound’s environmental persistence data?
- Methodological Answer : Apply multivariate regression to correlate degradation rates with variables like soil pH, organic matter content, and microbial activity. Use Kaplan-Meier survival analysis for half-life estimations in long-term environmental matrices .
Q. How can researchers ensure reproducibility in this compound-based experiments?
- Methodological Answer : Document all synthetic steps, including batch-specific variations (e.g., solvent lot numbers). Share raw spectral data (NMR, XRD) in open-access repositories. Cross-validate results via round-robin testing with independent labs, adhering to ISO/IEC 17025 standards .
Q. Regulatory & Interdisciplinary Considerations
Q. What regulatory frameworks govern this compound’s use in academic research?
- Methodological Answer : Comply with REACH restrictions (0.1% w/w limit in mixtures) and EPA Toxic Substances Control Act (TSCA) reporting for Pb content. For international collaborations, align with EU Directive 2011/65/EU (RoHS) on hazardous substances .
Q. How does this compound interact with biomolecules in pharmacological studies?
- Methodological Answer : Use surface plasmon resonance (SPR) or isothermal titration calorimetry (ITC) to quantify binding affinities with proteins (e.g., albumin). Pair with molecular docking simulations to identify interaction hotspots. Assess cytotoxicity via MTT assays in hepatic (HepG2) and neuronal (SH-SY5Y) cell lines .
Q. Tables for Quick Reference
Eigenschaften
CAS-Nummer |
10294-58-3 |
---|---|
Molekularformel |
H2O4P2Pb+2 |
Molekulargewicht |
335 g/mol |
IUPAC-Name |
lead(2+);phosphenous acid |
InChI |
InChI=1S/2HO2P.Pb/c2*1-3-2;/h2*(H,1,2);/q;;+2 |
InChI-Schlüssel |
KQWFPMFJUCTEFY-UHFFFAOYSA-N |
Kanonische SMILES |
OP=O.OP=O.[Pb+2] |
Herkunft des Produkts |
United States |
Haftungsausschluss und Informationen zu In-Vitro-Forschungsprodukten
Bitte beachten Sie, dass alle Artikel und Produktinformationen, die auf BenchChem präsentiert werden, ausschließlich zu Informationszwecken bestimmt sind. Die auf BenchChem zum Kauf angebotenen Produkte sind speziell für In-vitro-Studien konzipiert, die außerhalb lebender Organismen durchgeführt werden. In-vitro-Studien, abgeleitet von dem lateinischen Begriff "in Glas", beinhalten Experimente, die in kontrollierten Laborumgebungen unter Verwendung von Zellen oder Geweben durchgeführt werden. Es ist wichtig zu beachten, dass diese Produkte nicht als Arzneimittel oder Medikamente eingestuft sind und keine Zulassung der FDA für die Vorbeugung, Behandlung oder Heilung von medizinischen Zuständen, Beschwerden oder Krankheiten erhalten haben. Wir müssen betonen, dass jede Form der körperlichen Einführung dieser Produkte in Menschen oder Tiere gesetzlich strikt untersagt ist. Es ist unerlässlich, sich an diese Richtlinien zu halten, um die Einhaltung rechtlicher und ethischer Standards in Forschung und Experiment zu gewährleisten.