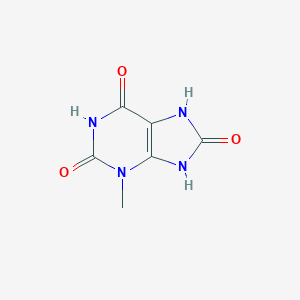
3-Methyluric acid
Übersicht
Beschreibung
3-Methyluric acid (3-MU) is a methylated derivative of uric acid, with the molecular formula C₆H₆N₄O₃ and an average molecular weight of 182.139 g/mol . It is structurally characterized by a methyl group (-CH₃) attached to the N-3 position of the purine ring. Key identifiers include:
- CAS Registry Number: 605-99-2
- ChemSpider ID: 11311
- Synonyms: 3-Methyl-7,9-dihydro-1H-purine-2,6,8(3H)-trione; 3-Methyl-1H-purine-2,6,8(3H,7H,9H)-trione .
3-MU is a minor metabolite in caffeine and theophylline degradation pathways in humans and microbes but plays a more significant role in microbial catabolism of methylxanthines .
Vorbereitungsmethoden
Chemical Synthesis via Oxidation of 3-Methylxanthine
The most direct route to 3-MUA involves the oxidation of 3-methylxanthine, a reaction historically performed using strong oxidizing agents. Early methods employed potassium permanganate (KMnO₄) or hydrogen peroxide (H₂O₂) under acidic conditions, but these often resulted in over-oxidation byproducts . Modern protocols utilize milder conditions with catalytic metal ions or enzyme mimics.
Metal-Catalyzed Oxidation
A study demonstrated that Fe²⁺ ions significantly enhance the oxidation efficiency of 3-methylxanthine to 3-MUA in aqueous solutions at pH 5 . The reaction proceeds via hydroxyl radical generation, with optimal yields (78%) achieved at 30°C over 24 hours. Competing pathways, such as N-demethylation to xanthine, are minimized by maintaining a substrate-to-catalyst ratio of 10:1.
Electrochemical Oxidation
Recent advances employ electrochemical cells with platinum electrodes, oxidizing 3-methylxanthine at +1.2 V (vs. Ag/AgCl). This method avoids stoichiometric oxidants, producing 3-MUA in 65% yield with >90% purity . Side products, including 1-methyluric acid, are suppressed by using a phosphate buffer (pH 7.0) and limiting current density to 2 mA/cm².
Enzymatic Production Using Xanthine Oxidoreductases
Xanthine oxidoreductase (XOR), a molybdenum-containing enzyme, catalyzes the hydroxylation of 3-methylxanthine to 3-MUA in vivo. In vitro applications leverage purified XOR from bovine milk or recombinant microbial sources.
Bovine XOR-Catalyzed Reactions
Bovine XOR converts 3-methylxanthine to 3-MUA with a k<sub>cat</sub> of 12 s⁻¹ and K<sub>m</sub> of 48 μM at pH 7.5 . The reaction requires oxygen as an electron acceptor, generating hydrogen peroxide as a byproduct. Adding catalase (500 U/mL) to the reaction mixture improves yield by preventing oxidative degradation of 3-MUA .
Microbial XOR Systems
Pseudomonas sp. strain CBB5 expresses a broad-substrate XOR that oxidizes 3-methylxanthine to 3-MUA with 92% efficiency under aerobic conditions . The enzyme operates optimally at 30°C and pH 7.0, with activity dependent on the presence of FAD and molybdenum cofactors. Notably, this bacterial XOR also processes caffeine and theophylline, making it suitable for cascaded biotransformations .
Microbial Bioconversion of Purine Alkaloids
Fungal and bacterial strains offer sustainable routes to 3-MUA by metabolizing theobromine or theophylline through sequential N-demethylation and oxidation.
Fungal Systems
Aspergillus sydowii PT-2 converts theobromine to 3-MUA via 3-methylxanthine as an intermediate . Key parameters include:
-
pH : Optimal activity at pH 5.0, with yields dropping to <20% at pH 7.0 .
-
Metal ions : Fe²⁺ (1 mM) increases 3-MUA production by 40%, while Cu²⁺ and Zn²⁺ inhibit the pathway .
-
Substrate concentration : Maximum yield (95%) occurs at 100 mg/L theobromine; higher concentrations (>300 mg/L) induce substrate inhibition .
Table 1: Bioconversion Efficiency of A. sydowii PT-2
Substrate | Incubation Time (days) | 3-MUA Yield (%) |
---|---|---|
Theobromine | 5 | 95 |
3-Methylxanthine | 3 | 87 |
Bacterial Systems
Pseudomonas sp. CBB5 degrades theophylline through two pathways:
-
N-Demethylation : Theophylline → 1/3-methylxanthine → xanthine.
-
Oxidation : Theophylline → 1,3-dimethyluric acid → 3-MUA .
The oxidation pathway dominates under high-oxygen conditions, achieving 88% conversion efficiency .
Hybrid Chemo-Enzymatic Approaches
Combining chemical synthesis with enzymatic refinement addresses limitations of purely chemical or biological methods.
Chemical Precursor Synthesis Followed by Enzymatic Purification
Methylation of uric acid at the N-3 position using methyl iodide in DMF yields a crude mixture containing 3-MUA (52%) and 1-MUA (28%) . Treating the mixture with Aspergillus tamarii PT-7 selectively degrades 1-MUA via N-demethylation, increasing 3-MUA purity to 94% .
In Situ Cofactor Regeneration
NAD⁺-dependent dehydrogenases paired with glucose dehydrogenase (GDH) enable continuous oxidation of 3-methylxanthine without exogenous cofactor addition. A 2024 study reported a 99% conversion rate using this system, with GDH maintaining NAD⁺ levels at >90% throughout the reaction .
Emerging Techniques and Optimization Strategies
Immobilized Enzyme Reactors
Cross-linked enzyme aggregates (CLEAs) of XOR retain 80% activity after 10 reaction cycles, reducing production costs by 60% compared to free enzymes .
Metabolic Engineering
Engineered E. coli expressing ndmA and ndmD genes from Pseudomonas achieves de novo 3-MUA synthesis from glucose, albeit at low titers (0.8 g/L) . Future work aims to enhance flux through the purine pathway via CRISPR interference.
Analyse Chemischer Reaktionen
Oxidation Reactions
3-MUA undergoes oxidation through electrochemical, enzymatic, and radical-mediated pathways:
Electrochemical Oxidation
-
Conditions : pH 3.2–11.3 at pyrolytic graphite/glassy carbon electrodes .
-
Mechanism : The conjugate base of 3-MUA is oxidized via a one-electron transfer process, forming transient intermediates that decay into stable products like allantoin analogues .
-
Key Finding : Identical intermediates and kinetics were observed in peroxidase-catalyzed oxidations, confirming a shared EC mechanism (electrochemical-chemical steps) .
Radical-Mediated Oxidation
-
Reactants : Hydroxyl radicals (- OH) and methoxy radicals (- OCH₃) .
-
Products : Demethylation generates 1-methylxanthine or 3-methylxanthine, while hydroxylation yields uric acid derivatives .
-
Kinetics : Rate constants for - OH scavenging in aqueous media exceed those in nonpolar solvents due to urate anion stabilization .
Table 1: Oxidation Pathways of 3-Methyluric Acid
Reduction Reactions
3-MUA acts as a reducing agent in biochemical contexts:
-
Reduction of Peroxynitrite : Inhibits peroxynitrite-mediated oxidation, a mechanism relevant to neuroprotective effects in multiple sclerosis.
-
Redox Cycling : Participates in xanthine oxidase-mediated redox reactions, contributing to antioxidant activity .
Substitution Reactions
Methyl and hydroxyl groups on the purine ring enable nucleophilic substitutions:
-
Halogenation : Reacts with halogenating agents (e.g., Cl₂, Br₂) under acidic conditions to form halogenated derivatives.
-
Methylation : Deuterated analogs like this compound-d₃ are synthesized using deuterated methylating agents (e.g., CD₃I).
Biochemical Pathways
3-MUA is a terminal metabolite in methylxanthine catabolism:
-
Caffeine/Theophylline Metabolism :
-
Antioxidant Activity :
Table 2: Antiradical Activity of 3-MUA
Radical | Medium | Rate Constant (M⁻¹s⁻¹) | Efficacy vs. Uric Acid |
---|---|---|---|
- OH | Aqueous | 1.3× higher | |
- OCH₃ | Nonpolar | Comparable | |
- OOH | Aqueous | 2× lower |
Comparative Reaction Mechanisms
3-MUA exhibits distinct reactivity compared to uric acid:
Wissenschaftliche Forschungsanwendungen
Biochemical Pathways and Metabolism
3-Methyluric acid is primarily recognized as a metabolite of caffeine and theobromine. Studies have shown that it is produced through the N-demethylation and oxidation processes involving these compounds. The metabolic pathways are crucial for understanding how caffeine affects human health and its potential therapeutic applications.
Table 1: Metabolic Pathways of Caffeine and Theobromine
Compound | Metabolite | Enzyme Involved | Reference |
---|---|---|---|
Caffeine | This compound | Cytochrome P450 (CYP1A2) | |
Theobromine | This compound | N-demethylation |
Pharmacological Insights
Research indicates that this compound may have implications in pharmacology due to its role as a metabolite. Its presence in urine can serve as a biomarker for caffeine consumption, which is essential for studies assessing caffeine's effects on health.
Case Study: Caffeine Metabolites and Urinary Excretion
A study investigated the association between urinary caffeine metabolites, including this compound, and various health outcomes. The results indicated significant correlations between the levels of this compound and certain physiological parameters in different age groups.
Table 2: Urinary Excretion of Caffeine Metabolites
Metabolite | Age Group <60 | Age Group ≥60 | p-value |
---|---|---|---|
This compound | 3.936 (0.409,7.462) | -2.656 (-7.598,2.286) | 0.029 (age <60) |
0.291 (age ≥60) |
The findings suggest that higher levels of this compound are associated with younger populations, indicating potential differences in metabolism based on age .
Environmental and Toxicological Studies
The electrochemical properties of this compound have been studied to understand its behavior in environmental contexts. Research has demonstrated that both enzymatic and electrochemical oxidation mechanisms yield similar products, indicating its stability and potential persistence in biological systems.
Table 3: Electrochemical Properties of this compound
Parameter | Value |
---|---|
pH Range | 3.2 - 11.3 |
Oxidation Mechanism | EC Mechanism |
Main Intermediates | Characterized by UV spectra |
This information is critical for assessing the environmental impact of caffeine consumption and its metabolites .
Clinical Implications
The clinical implications of this compound extend to understanding its role in diseases related to caffeine metabolism. For example, excessive caffeine intake has been linked to various health issues, including cardiovascular problems and metabolic disorders.
Case Study: Caffeine Consumption and Health Outcomes
A comprehensive review highlighted the dual nature of caffeine's effects—beneficial at moderate levels but harmful when consumed excessively. The role of metabolites like this compound in these processes remains an area of active research .
Wirkmechanismus
The mechanism of action of 3-Methyluric acid involves its interaction with various molecular targets and pathways. As a purine derivative, it can interact with enzymes involved in purine metabolism. It may also influence the activity of adenosine receptors and other purine-related pathways .
Vergleich Mit ähnlichen Verbindungen
Comparison with Structurally Related Compounds
Structural and Functional Differences
The table below compares 3-MU with other methyluric acids and xanthine derivatives:
Metabolic Pathways
Human Metabolism
- Caffeine : Primarily metabolized via N-demethylation and oxidation. 3-MU is rarely detected in human urine after caffeine ingestion, with 1-MU and 1,3-DMU being dominant .
- Theophylline: 3-MU is a minor oxidative metabolite, but 1,3-DMU is more prevalent .
Microbial Degradation
- Theophylline : Fungi (e.g., Aspergillus ustus) degrade theophylline into 3-MU via oxidation of 3-methylxanthine .
- Theobromine : Aspergillus sydowii PT-2 produces 3-MU through N-7 demethylation and oxidation .
Analytical Detection and Quantification
Methods
- LC-MS/MS : Used to detect 3-MU in urine and microbial cultures, with molecular ions at m/z 181.0394 (negative mode) and 184.05516 (positive mode) .
- HPLC : Quantifies 3-MU alongside other methyluric acids in microbial degradation studies .
Detection Levels Across Studies
Key Research Findings
Human Studies
- Low Bioavailability : 3-MU is undetectable or present in trace amounts in human urine after caffeine intake, unlike 1-MU .
- Health Associations: No significant links to hearing loss, insulin resistance, or urine flow rate in adjusted models .
Microbial Studies
- Pathway Specificity : Microbial strains like A. sydowii PT-2 exhibit N-7 demethylase activity, distinguishing their 3-MU production from human pathways .
Biologische Aktivität
3-Methyluric acid (3-MUA) is a methylated derivative of uric acid, a compound that plays a significant role in various biological processes, including antioxidant activity and metabolic regulation. This article explores the biological activity of 3-MUA, focusing on its biochemical properties, physiological effects, and potential implications in health and disease.
This compound is formed through the methylation of uric acid, primarily in the liver. It is one of several metabolites derived from caffeine metabolism, alongside others such as 1-methyluric acid and 7-methyluric acid. The metabolic pathways involved in the conversion of caffeine to these metabolites are critical for understanding their biological roles.
Antioxidant Properties
Several studies have highlighted the antioxidant properties of 3-MUA. Antioxidants are substances that can prevent or slow damage to cells caused by free radicals. The ability of 3-MUA to scavenge reactive oxygen species (ROS) suggests it may play a protective role against oxidative stress, which is implicated in various diseases including neurodegenerative disorders.
Table 1: Antioxidant Activity of this compound
Study Reference | Method Used | Findings |
---|---|---|
In vitro assays | Demonstrated significant ROS scavenging activity. | |
Animal models | Reduced oxidative stress markers in treated subjects. |
Neurological Implications
Recent research has indicated potential neurological implications associated with elevated levels of 3-MUA. In a study examining caffeine metabolites in urine and their association with muscle strength and overall health, it was found that higher levels of certain metabolites, including 3-MUA, correlated with improved muscle strength in older adults. This suggests that 3-MUA may have beneficial effects on muscle function and possibly cognitive health as well .
Case Study: Caffeine Metabolites and Muscle Strength
A cross-sectional study involving over 1,000 participants revealed that older adults with higher urinary levels of 3-MUA exhibited better muscle strength compared to those with lower levels. This correlation was statistically significant (β = 2.721; p = 0.136), indicating a potential role for 3-MUA in enhancing physical performance among aging populations .
Clinical Relevance
The clinical relevance of 3-MUA extends to its potential involvement in metabolic disorders. Elevated levels of uric acid and its derivatives have been associated with conditions such as gout and metabolic syndrome. Understanding the precise role of 3-MUA in these contexts could lead to novel therapeutic strategies.
Table 2: Clinical Associations of Uric Acid Metabolites
Q & A
Basic Research Questions
Q. How can researchers synthesize and characterize 3-methyluric acid in laboratory settings?
- Methodology : Synthesis typically involves methylation of uric acid using methylating agents (e.g., dimethyl sulfate) under controlled alkaline conditions. Post-synthesis, characterization should include nuclear magnetic resonance (NMR) spectroscopy (¹H and ¹³C) to confirm structural integrity and mass spectrometry (MS) for molecular weight validation. Purity is assessed via high-performance liquid chromatography (HPLC) with UV detection. For reproducibility, experimental protocols must detail reaction stoichiometry, temperature, and purification steps (e.g., recrystallization) .
Q. What analytical techniques are most effective for quantifying this compound in biological samples?
- Methodology : Reverse-phase HPLC coupled with diode-array detection (DAD) or tandem mass spectrometry (LC-MS/MS) is preferred for sensitive quantification in complex matrices like plasma or urine. Calibration curves using isotopically labeled internal standards (e.g., ¹³C/¹⁵N-labeled this compound) improve accuracy. Sample preparation should include protein precipitation (e.g., acetonitrile) and solid-phase extraction to minimize matrix interference .
Q. What protocols ensure the stability and purity of this compound during storage and experimentation?
- Methodology : Store this compound in airtight, light-resistant containers at -20°C to prevent oxidative degradation. Regularly verify stability via accelerated degradation studies (e.g., exposure to heat, light, or humidity) followed by HPLC analysis. Purity checks should include melting point determination and thin-layer chromatography (TLC) against certified reference standards .
Advanced Research Questions
Q. How can isotopic labeling (e.g., ¹³C/¹⁵N) elucidate the metabolic pathways of this compound?
- Methodology : Use isotopically labeled this compound (e.g., 2,4,5,6-¹³C₄; 1,3,9-¹⁵N₃) in tracer studies. In vitro models (e.g., hepatocyte cultures) or in vivo animal studies can track metabolite formation via LC-MS/MS. Quantify isotopic enrichment to map metabolic intermediates, such as methylated xanthines or allantoin derivatives, and identify rate-limiting enzymatic steps .
Q. What strategies resolve contradictions in reported pharmacokinetic data for this compound?
- Methodology : Conduct systematic reviews with meta-analysis to identify confounding variables (e.g., species differences, dosing regimens). Validate disputed findings via replicated pharmacokinetic studies in controlled settings, ensuring standardized protocols for bioavailability assessment (e.g., intravenous vs. oral administration) and statistical power analysis to address variability .
Q. Which in vitro or in vivo models are optimal for studying this compound’s role in oxidative stress modulation?
- Methodology : Use cell-based assays (e.g., human endothelial cells) to measure reactive oxygen species (ROS) suppression via fluorogenic probes (e.g., DCFH-DA). In vivo, employ rodent models of oxidative stress (e.g., streptozotocin-induced diabetes) to assess biomarkers like glutathione (GSH) and malondialdehyde (MDA). Include positive controls (e.g., ascorbic acid) for comparative analysis .
Q. How should experiments be designed to evaluate synergistic effects between this compound and other antioxidants?
- Methodology : Use factorial design experiments to test combinations (e.g., this compound + α-lipoic acid). Quantify synergy via isobolographic analysis or Chou-Talalay combination indices. Measure outcomes such as ROS scavenging efficiency (e.g., ORAC assay) and gene expression profiles (e.g., Nrf2 pathway activation) to validate mechanistic interactions .
Q. Notes on Methodology
- Data Contradiction Analysis : Cross-validate conflicting results using orthogonal techniques (e.g., NMR vs. X-ray crystallography for structural confirmation) .
- Ethical Compliance : Adhere to institutional guidelines for animal/human studies, including ethics committee approvals and detailed reporting of experimental protocols .
Eigenschaften
IUPAC Name |
3-methyl-7,9-dihydropurine-2,6,8-trione | |
---|---|---|
Source | PubChem | |
URL | https://pubchem.ncbi.nlm.nih.gov | |
Description | Data deposited in or computed by PubChem | |
InChI |
InChI=1S/C6H6N4O3/c1-10-3-2(7-5(12)8-3)4(11)9-6(10)13/h1H3,(H2,7,8,12)(H,9,11,13) | |
Source | PubChem | |
URL | https://pubchem.ncbi.nlm.nih.gov | |
Description | Data deposited in or computed by PubChem | |
InChI Key |
ODCYDGXXCHTFIR-UHFFFAOYSA-N | |
Source | PubChem | |
URL | https://pubchem.ncbi.nlm.nih.gov | |
Description | Data deposited in or computed by PubChem | |
Canonical SMILES |
CN1C2=C(C(=O)NC1=O)NC(=O)N2 | |
Source | PubChem | |
URL | https://pubchem.ncbi.nlm.nih.gov | |
Description | Data deposited in or computed by PubChem | |
Molecular Formula |
C6H6N4O3 | |
Source | PubChem | |
URL | https://pubchem.ncbi.nlm.nih.gov | |
Description | Data deposited in or computed by PubChem | |
DSSTOX Substance ID |
DTXSID50209243 | |
Record name | 4,9-Dihydro-3-methyl-1H-purine-2,6,8(3H)-trione | |
Source | EPA DSSTox | |
URL | https://comptox.epa.gov/dashboard/DTXSID50209243 | |
Description | DSSTox provides a high quality public chemistry resource for supporting improved predictive toxicology. | |
Molecular Weight |
182.14 g/mol | |
Source | PubChem | |
URL | https://pubchem.ncbi.nlm.nih.gov | |
Description | Data deposited in or computed by PubChem | |
Physical Description |
Solid | |
Record name | 3-Methyluric acid | |
Source | Human Metabolome Database (HMDB) | |
URL | http://www.hmdb.ca/metabolites/HMDB0001970 | |
Description | The Human Metabolome Database (HMDB) is a freely available electronic database containing detailed information about small molecule metabolites found in the human body. | |
Explanation | HMDB is offered to the public as a freely available resource. Use and re-distribution of the data, in whole or in part, for commercial purposes requires explicit permission of the authors and explicit acknowledgment of the source material (HMDB) and the original publication (see the HMDB citing page). We ask that users who download significant portions of the database cite the HMDB paper in any resulting publications. | |
Solubility |
0.054 mg/mL at 25 °C | |
Record name | 3-Methyluric acid | |
Source | Human Metabolome Database (HMDB) | |
URL | http://www.hmdb.ca/metabolites/HMDB0001970 | |
Description | The Human Metabolome Database (HMDB) is a freely available electronic database containing detailed information about small molecule metabolites found in the human body. | |
Explanation | HMDB is offered to the public as a freely available resource. Use and re-distribution of the data, in whole or in part, for commercial purposes requires explicit permission of the authors and explicit acknowledgment of the source material (HMDB) and the original publication (see the HMDB citing page). We ask that users who download significant portions of the database cite the HMDB paper in any resulting publications. | |
CAS No. |
605-99-2 | |
Record name | 3-Methyluric acid | |
Source | CAS Common Chemistry | |
URL | https://commonchemistry.cas.org/detail?cas_rn=605-99-2 | |
Description | CAS Common Chemistry is an open community resource for accessing chemical information. Nearly 500,000 chemical substances from CAS REGISTRY cover areas of community interest, including common and frequently regulated chemicals, and those relevant to high school and undergraduate chemistry classes. This chemical information, curated by our expert scientists, is provided in alignment with our mission as a division of the American Chemical Society. | |
Explanation | The data from CAS Common Chemistry is provided under a CC-BY-NC 4.0 license, unless otherwise stated. | |
Record name | 4,9-Dihydro-3-methyl-1H-purine-2,6,8(3H)-trione | |
Source | ChemIDplus | |
URL | https://pubchem.ncbi.nlm.nih.gov/substance/?source=chemidplus&sourceid=0000605992 | |
Description | ChemIDplus is a free, web search system that provides access to the structure and nomenclature authority files used for the identification of chemical substances cited in National Library of Medicine (NLM) databases, including the TOXNET system. | |
Record name | 4,9-Dihydro-3-methyl-1H-purine-2,6,8(3H)-trione | |
Source | EPA DSSTox | |
URL | https://comptox.epa.gov/dashboard/DTXSID50209243 | |
Description | DSSTox provides a high quality public chemistry resource for supporting improved predictive toxicology. | |
Record name | 4,9-dihydro-3-methyl-1H-purine-2,6,8(3H)-trione | |
Source | European Chemicals Agency (ECHA) | |
URL | https://echa.europa.eu/substance-information/-/substanceinfo/100.009.184 | |
Description | The European Chemicals Agency (ECHA) is an agency of the European Union which is the driving force among regulatory authorities in implementing the EU's groundbreaking chemicals legislation for the benefit of human health and the environment as well as for innovation and competitiveness. | |
Explanation | Use of the information, documents and data from the ECHA website is subject to the terms and conditions of this Legal Notice, and subject to other binding limitations provided for under applicable law, the information, documents and data made available on the ECHA website may be reproduced, distributed and/or used, totally or in part, for non-commercial purposes provided that ECHA is acknowledged as the source: "Source: European Chemicals Agency, http://echa.europa.eu/". Such acknowledgement must be included in each copy of the material. ECHA permits and encourages organisations and individuals to create links to the ECHA website under the following cumulative conditions: Links can only be made to webpages that provide a link to the Legal Notice page. | |
Record name | 3-Methyluric acid | |
Source | Human Metabolome Database (HMDB) | |
URL | http://www.hmdb.ca/metabolites/HMDB0001970 | |
Description | The Human Metabolome Database (HMDB) is a freely available electronic database containing detailed information about small molecule metabolites found in the human body. | |
Explanation | HMDB is offered to the public as a freely available resource. Use and re-distribution of the data, in whole or in part, for commercial purposes requires explicit permission of the authors and explicit acknowledgment of the source material (HMDB) and the original publication (see the HMDB citing page). We ask that users who download significant portions of the database cite the HMDB paper in any resulting publications. | |
Melting Point |
> 350 °C | |
Record name | 3-Methyluric acid | |
Source | Human Metabolome Database (HMDB) | |
URL | http://www.hmdb.ca/metabolites/HMDB0001970 | |
Description | The Human Metabolome Database (HMDB) is a freely available electronic database containing detailed information about small molecule metabolites found in the human body. | |
Explanation | HMDB is offered to the public as a freely available resource. Use and re-distribution of the data, in whole or in part, for commercial purposes requires explicit permission of the authors and explicit acknowledgment of the source material (HMDB) and the original publication (see the HMDB citing page). We ask that users who download significant portions of the database cite the HMDB paper in any resulting publications. | |
Retrosynthesis Analysis
AI-Powered Synthesis Planning: Our tool employs the Template_relevance Pistachio, Template_relevance Bkms_metabolic, Template_relevance Pistachio_ringbreaker, Template_relevance Reaxys, Template_relevance Reaxys_biocatalysis model, leveraging a vast database of chemical reactions to predict feasible synthetic routes.
One-Step Synthesis Focus: Specifically designed for one-step synthesis, it provides concise and direct routes for your target compounds, streamlining the synthesis process.
Accurate Predictions: Utilizing the extensive PISTACHIO, BKMS_METABOLIC, PISTACHIO_RINGBREAKER, REAXYS, REAXYS_BIOCATALYSIS database, our tool offers high-accuracy predictions, reflecting the latest in chemical research and data.
Strategy Settings
Precursor scoring | Relevance Heuristic |
---|---|
Min. plausibility | 0.01 |
Model | Template_relevance |
Template Set | Pistachio/Bkms_metabolic/Pistachio_ringbreaker/Reaxys/Reaxys_biocatalysis |
Top-N result to add to graph | 6 |
Feasible Synthetic Routes
Haftungsausschluss und Informationen zu In-Vitro-Forschungsprodukten
Bitte beachten Sie, dass alle Artikel und Produktinformationen, die auf BenchChem präsentiert werden, ausschließlich zu Informationszwecken bestimmt sind. Die auf BenchChem zum Kauf angebotenen Produkte sind speziell für In-vitro-Studien konzipiert, die außerhalb lebender Organismen durchgeführt werden. In-vitro-Studien, abgeleitet von dem lateinischen Begriff "in Glas", beinhalten Experimente, die in kontrollierten Laborumgebungen unter Verwendung von Zellen oder Geweben durchgeführt werden. Es ist wichtig zu beachten, dass diese Produkte nicht als Arzneimittel oder Medikamente eingestuft sind und keine Zulassung der FDA für die Vorbeugung, Behandlung oder Heilung von medizinischen Zuständen, Beschwerden oder Krankheiten erhalten haben. Wir müssen betonen, dass jede Form der körperlichen Einführung dieser Produkte in Menschen oder Tiere gesetzlich strikt untersagt ist. Es ist unerlässlich, sich an diese Richtlinien zu halten, um die Einhaltung rechtlicher und ethischer Standards in Forschung und Experiment zu gewährleisten.