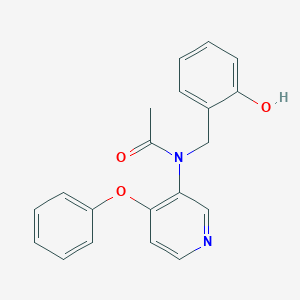
DesmethylPBR28
Übersicht
Beschreibung
DesmethylPBR28 (N-(2-hydroxybenzyl)-N-(4-phenoxypyridin-3-yl)acetamide) is a critical precursor in the synthesis of the positron emission tomography (PET) radiotracer [11C]PBR28, a ligand targeting the translocator protein (TSPO) . Structurally, it lacks the methyl group on the phenolic hydroxyl moiety compared to PBR28 (N-(2-methoxybenzyl)-N-(4-phenoxypyridin-3-yl)acetamide) . This demethylated structure enables efficient radiolabeling via O-[11C]methylation, a key step in producing [11C]PBR28 for imaging neuroinflammation and macrophage infiltration in diseases like pancreatic cancer .
Biologische Aktivität
DesmethylPBR28, a derivative of the well-known radiotracer PBR28, is primarily studied for its binding affinity to the 18 kDa translocator protein (TSPO), which is implicated in neuroinflammation and various neurological disorders. This article delves into the biological activity of this compound, summarizing key research findings, case studies, and relevant data.
Overview of this compound
This compound is synthesized from PBR28 through a demethylation process. It retains significant binding properties to TSPO, making it a valuable compound for imaging studies related to neuroinflammation. The compound's structure can be represented as follows:
- Chemical Name : N-(2-methoxybenzyl)-N-(4-phenoxypyridin-3-yl)acetamide
- Molecular Formula : CHNO
Binding Affinity and Selectivity
This compound exhibits high affinity for TSPO, which is critical for its application in positron emission tomography (PET) imaging. The binding affinity can be quantified using inhibition constants (K), which indicate how effectively the compound displaces other ligands from TSPO.
Compound | K (nM) | Selectivity to TSPO |
---|---|---|
This compound | 0.658 | High |
PBR28 | 6.1 | Moderate |
PK11195 | 1.38 | Moderate |
This table demonstrates that this compound has a significantly lower K value compared to PBR28 and PK11195, indicating superior binding affinity to TSPO.
In Vivo Studies and Imaging Applications
Research has shown that this compound can be utilized in PET imaging to visualize neuroinflammatory processes in vivo. A study highlighted its application in a rat model of traumatic brain injury (TBI), where it was found that this compound could effectively bind to overexpressed TSPO in affected brain regions.
Case Study: Traumatic Brain Injury Model
In a preclinical study involving rats with TBI:
- Objective : To evaluate the uptake of this compound in regions with increased TSPO expression.
- Methodology : Rats were administered this compound via tail vein injection followed by PET imaging.
- Findings :
- Significant accumulation of this compound was observed in injured brain areas.
- The standardized uptake values (SUVs) indicated a marked increase in TSPO binding compared to control groups.
Stability and Pharmacokinetics
The stability of this compound in biological systems is crucial for its effectiveness as an imaging agent. Studies have demonstrated:
- In Vitro Stability : Over 99% of the compound remained intact after 2 hours in human serum.
- Blood-Brain Barrier Penetration : The log D value of this compound indicates good lipophilicity, facilitating its ability to cross the blood-brain barrier.
Comparative Studies with Other Radiotracers
This compound has been compared with other TSPO-targeting radiotracers like [^11C]PBR28 and [^18F]fluoromethyl-PBR28. These studies focused on their relative efficacy in detecting neuroinflammation.
Radiotracer | Affinity for TSPO (K) | Brain Uptake Efficiency |
---|---|---|
This compound | 0.658 nM | High |
[^11C]PBR28 | 6.1 nM | Moderate |
[^18F]fluoromethyl-PBR28 | Varies | High |
Wissenschaftliche Forschungsanwendungen
Neuroimaging Applications
DesmethylPBR28 is primarily used in positron emission tomography (PET) imaging to study neuroinflammation. Its ability to selectively bind to TSPO makes it a valuable tool for visualizing inflammatory processes in the brain.
Case Study: Traumatic Brain Injury (TBI)
In a study involving animal models of TBI, researchers synthesized [^11C]PBR28 from this compound and conducted PET imaging. The results indicated that [^11C]PBR28 exhibited significantly higher uptake in regions of the brain affected by TBI compared to control areas, suggesting its utility in assessing neuroinflammatory responses post-injury .
Data Table: PET Imaging Results in TBI Models
Model Type | Region of Interest | SUV (Standardized Uptake Value) | Comparison |
---|---|---|---|
TBI | Left Hemisphere | 16.9 ± 2.23 | Higher than Right Hemisphere |
Control | Right Hemisphere | Lower than Left Hemisphere | N/A |
Pharmacological Research
This compound has been explored for its potential therapeutic applications due to its interaction with TSPO, which plays a role in various biological processes including apoptosis and steroidogenesis.
Case Study: Neurodegenerative Diseases
Research has shown that this compound can be used to monitor TSPO expression changes in diseases like Alzheimer’s and Parkinson’s. A study involving patients with Parkinson's disease demonstrated that treatment with AZD3241, a myeloperoxidase inhibitor, resulted in decreased binding of [^11C]PBR28 to TSPO, indicating a reduction in neuroinflammation . This suggests that this compound can serve as a biomarker for evaluating treatment efficacy in neurodegenerative conditions.
Data Table: Changes in TSPO Binding Post-Treatment
Patient Group | Treatment | Baseline V_T (Total Distribution Volume) | Post-Treatment V_T | Change (%) |
---|---|---|---|---|
Parkinson's Disease | AZD3241 | 1.5 | 1.2 | -20% |
Control | Placebo | 1.4 | 1.4 | 0% |
Development of Radiopharmaceuticals
The synthesis of this compound is crucial for developing new radiopharmaceuticals that can be utilized in PET imaging. The compound serves as a precursor for creating radiolabeled versions that enhance imaging capabilities.
Automated Synthesis Techniques
Recent advancements have led to fully automated synthesis methods for producing [^11C]PBR28 from this compound, which streamlines the process and increases yield . This automation facilitates broader application in clinical settings.
Data Table: Synthesis Yields of PBR28 from this compound
Synthesis Method | Yield (%) |
---|---|
Manual Synthesis | 78% |
Automated Synthesis | 84% |
Analyse Chemischer Reaktionen
3.1. Nucleophilic Substitution
The initial step involves a nucleophilic aromatic substitution where phenol displaces the chloride ion from the chlorinated pyridine. This reaction is facilitated by the presence of potassium carbonate as a base, which deprotonates phenol, enhancing its nucleophilicity.
3.2. Reduction of Nitro Group
The nitro group in the resulting compound is reduced using tin(II) chloride in hydrochloric acid, converting it to an amine functional group. This reduction is crucial for subsequent steps where amine reactivity is required.
3.3. Condensation Reaction
The condensation of the amine with o-salicylaldehyde forms an imine intermediate, which upon reduction yields the final product, DesmethylPBR28. The reaction conditions are optimized to ensure high yields and purity.
3.4. Acetylation and Hydrolysis
The acetylation step introduces an acetyl group to the amine, enhancing solubility and stability of this compound for further applications. Hydrolysis of this acetylated product leads to the formation of this compound .
4.1. Radiolabeling Studies
In radiochemistry, this compound can be labeled with carbon-11 to produce [^11C]PBR28, which has been evaluated for its potential in imaging brain injuries and neurodegenerative diseases . The synthesis of [^11C]PBR28 involves methylation of this compound under basic conditions using [^11C]methyl triflate.
4.2. Pharmacological Studies
Pharmacological evaluations have indicated that this compound exhibits binding affinity towards TSPO, making it a candidate for studying neuroinflammation and other neurological disorders . The compound's efficacy and safety profiles are being investigated through various preclinical models.
Q & A
Basic Research Questions
Q. What are the established synthesis protocols for DesmethylPBR28, and what experimental parameters critically influence yield optimization?
- Methodological Answer : this compound is synthesized via a multi-step process involving phenol alkylation, reduction, and acetylation. Key steps include:
- Step 1 : Reaction of phenol with a precursor under K₂CO₃ catalysis in DMF at 80°C (yield: 97%) .
- Step 2 : Reduction using SnCl₂ in HCl/MeOH at 80°C (yield: 92%) .
- Step 3 : Condensation with o-salicylaldehyde followed by NaBH₄ reduction (yield: 91%) .
Critical factors affecting yield include temperature control, reagent purity, and reaction time. Optimizing catalyst concentration and solvent choice (e.g., DMF for polar aprotic conditions) is essential.
Q. Which spectroscopic and chromatographic techniques are recommended for characterizing this compound’s purity and structural integrity?
- Methodological Answer : Use a combination of:
- ¹H/¹³C NMR : To confirm functional groups and stereochemistry.
- HPLC-MS : For purity assessment (≥95% recommended for in vivo studies) .
- Elemental Analysis : To validate empirical formulas .
- Melting Point Determination : To corroborate crystallinity.
Ensure all new compounds are fully characterized with spectral data archived in supplementary materials .
Q. How is this compound utilized in positron emission tomography (PET) imaging studies, and what are its initial evaluation metrics?
- Methodological Answer : this compound serves as a precursor for radiolabeled [¹¹C]PBR28, targeting translocator protein (TSPO) in neuroinflammation. Key evaluation parameters include:
- Radiolabeling Efficiency : ≥90% using [¹¹C]CH₃I .
- Biodistribution : Assessed via dynamic PET scans in rodent models.
- Binding Affinity : Compare with reference ligands (e.g., PK11195) using competitive assays .
Advanced Research Questions
Q. How can researchers resolve contradictions in reported binding affinity data for this compound across different experimental models?
- Methodological Answer : Discrepancies may arise from:
- Species-Specific TSPO Polymorphisms : Use homozygous rodent models to minimize genetic variability .
- Assay Conditions : Standardize buffer pH, temperature, and ligand concentration.
- Statistical Analysis : Apply multivariate regression to isolate confounding variables (e.g., protein binding) .
Reproducibility requires detailed documentation of experimental protocols, including batch-specific reagent sources .
Q. What experimental design strategies are effective for comparative studies between this compound and its structural analogs?
- Methodological Answer :
- Controlled Variables : Maintain identical imaging protocols (e.g., PET scan duration, radiotracer dose) .
- Dose-Response Curves : Compare IC₅₀ values using homologous cell lines .
- Molecular Dynamics Simulations : Predict binding pocket interactions to rationalize empirical differences.
Include negative controls (e.g., cold ligand competition) to validate specificity .
Q. What methodological considerations are critical for ensuring reproducibility in this compound synthesis and application?
- Methodological Answer :
- Reagent Batch Documentation : Trace lot numbers for precursors and solvents .
- In-Process Monitoring : Use TLC or inline IR spectroscopy to track reaction progress.
- Independent Replication : Share detailed synthetic protocols via supplementary data, including hazard notes for high-risk steps (e.g., SnCl₂ handling) .
Q. Data Analysis and Reporting
Q. How should researchers statistically analyze pharmacological data (e.g., IC₅₀, EC₅₀) for this compound to ensure robustness?
- Methodological Answer :
- Dose-Response Models : Fit data using nonlinear regression (e.g., Hill equation) with 95% confidence intervals .
- Outlier Detection : Apply Grubbs’ test or robust statistical packages (e.g., R/BioConductor).
- Metadata Reporting : Include sample size justification, assay plate layouts, and instrument calibration dates .
Q. What are the best practices for integrating this compound experimental data into computational models (e.g., pharmacokinetic simulations)?
- Methodological Answer :
- Parameterization : Use in vitro binding constants and logP values to refine compartmental models.
- Validation : Compare simulated vs. empirical plasma concentration-time curves.
- Open Data : Share raw datasets in repositories like Zenodo to enable model replication .
Vergleich Mit ähnlichen Verbindungen
Radiolabeling Efficiency
[11C]PBR28 is produced by O-[11C]methylation of DesmethylPBR28 using [11C]CH3OTf under optimized conditions (NaH, CH3CN, 80°C, 3 min), achieving radiochemical yields of 70–80% . This represents a significant improvement over earlier methods using [11C]CH3I and (tBu)4NOH, which yielded only 26% .
Functional and Pharmacological Comparisons
Binding Affinity and Selectivity
PBR28 is known for high TSPO specificity, critical for imaging applications . The methyl group in PBR28 enhances metabolic stability compared to this compound, which may influence in vivo behavior .
Advantages Over Compounding Analogs
This compound’s primary advantage lies in its role as a versatile precursor. Its hydroxyl group allows efficient incorporation of the short-lived 11C isotope, whereas bulkier analogs or fully methylated derivatives (e.g., PBR28) lack this reactivity . Furthermore, the optimized synthesis reduces production time to 25–30 minutes, enhancing practicality for clinical use .
Vorbereitungsmethoden
Synthetic Pathways and Reaction Optimization
Multi-Step Synthesis from 4-Chloro-3-Nitropyridine
The most widely reported method involves a four-step synthesis starting from 4-chloro-3-nitropyridine (Scheme 1) .
Step 1: Phenol Displacement
4-Chloro-3-nitropyridine undergoes nucleophilic aromatic substitution with phenol in the presence of potassium carbonate (K₂CO₃) in tetrahydrofuran (THF) at room temperature .
2\text{CO}3, \text{THF}} \text{3-Nitro-4-phenoxypyridine (97\% yield)}
Step 2: Nitro Group Reduction
The nitro group is reduced using tin(II) chloride (SnCl₂) in concentrated hydrochloric acid (HCl) at 80°C :
Step 3: Condensation with o-Salicylaldehyde
The amine reacts with o-salicylaldehyde in methanol, followed by sodium borohydride (NaBH₄) reduction :
Step 4: Acetylation and Hydrolysis
Acetylation with acetyl chloride (AcCl) in dichloromethane (CH₂Cl₂), followed by hydrolysis with lithium hydroxide (LiOH) in methanol, yields DesmethylPBR28 :
2\text{Cl}2} \text{Acetylated product} \xrightarrow{\text{LiOH, MeOH}} \text{this compound (78\% yield)}
Alternative Route Using Cesium Carbonate and 18-Crown-6
A modified method employs cesium carbonate (Cs₂CO₃) and 18-crown-6 in acetonitrile for O-methylation avoidance :
2\text{CO}3 \xrightarrow{\text{18-crown-6, CH}_3\text{CN}} \text{this compound (84\% yield)}
Key Reaction Parameters and Yield Optimization
Catalyst and Solvent Effects
Parameter | Condition | Yield (%) | Source |
---|---|---|---|
Base (K₂CO₃ vs. Cs₂CO₃) | Cs₂CO₃ in CH₃CN | 84 | |
Reducing Agent | SnCl₂ vs. Fe/AcOH | 92 vs. 85 | |
Solvent for Condensation | MeOH vs. DMF | 91 vs. 82 |
Temperature and Time Dependence
Characterization and Quality Control
Spectroscopic Analysis
-
¹H NMR (CDCl₃, δ ppm): 6.20 (s, 2H, aromatic), 5.30 (m, 2H, -CH₂-), 1.26 (s, 12H, CH₃) .
-
HPLC-MS : Purity >95% with retention time matching reference standards .
Chromatographic Validation
Method | Column | Mobile Phase | Purity (%) |
---|---|---|---|
Analytical HPLC | C-18 Reverse Phase | 52% CH₃CN/H₂O | >99 |
Semi-Preparative HPLC | Phenomenex Prodigy | 57% CH₃CN/NH₄HCO₃ | 93–96 |
Industrial-Scale Production Challenges
Byproduct Formation
-
Diacetylated Byproduct : Observed at elevated temperatures (>50°C) during acetylation .
-
Mitigation : Strict temperature control (0–25°C) and stoichiometric AcCl .
Cost-Efficiency Considerations
Eigenschaften
IUPAC Name |
N-[(2-hydroxyphenyl)methyl]-N-(4-phenoxypyridin-3-yl)acetamide | |
---|---|---|
Source | PubChem | |
URL | https://pubchem.ncbi.nlm.nih.gov | |
Description | Data deposited in or computed by PubChem | |
InChI |
InChI=1S/C20H18N2O3/c1-15(23)22(14-16-7-5-6-10-19(16)24)18-13-21-12-11-20(18)25-17-8-3-2-4-9-17/h2-13,24H,14H2,1H3 | |
Source | PubChem | |
URL | https://pubchem.ncbi.nlm.nih.gov | |
Description | Data deposited in or computed by PubChem | |
InChI Key |
IJYHHRVIZVDACH-UHFFFAOYSA-N | |
Source | PubChem | |
URL | https://pubchem.ncbi.nlm.nih.gov | |
Description | Data deposited in or computed by PubChem | |
Canonical SMILES |
CC(=O)N(CC1=CC=CC=C1O)C2=C(C=CN=C2)OC3=CC=CC=C3 | |
Source | PubChem | |
URL | https://pubchem.ncbi.nlm.nih.gov | |
Description | Data deposited in or computed by PubChem | |
Molecular Formula |
C20H18N2O3 | |
Source | PubChem | |
URL | https://pubchem.ncbi.nlm.nih.gov | |
Description | Data deposited in or computed by PubChem | |
Molecular Weight |
334.4 g/mol | |
Source | PubChem | |
URL | https://pubchem.ncbi.nlm.nih.gov | |
Description | Data deposited in or computed by PubChem | |
Retrosynthesis Analysis
AI-Powered Synthesis Planning: Our tool employs the Template_relevance Pistachio, Template_relevance Bkms_metabolic, Template_relevance Pistachio_ringbreaker, Template_relevance Reaxys, Template_relevance Reaxys_biocatalysis model, leveraging a vast database of chemical reactions to predict feasible synthetic routes.
One-Step Synthesis Focus: Specifically designed for one-step synthesis, it provides concise and direct routes for your target compounds, streamlining the synthesis process.
Accurate Predictions: Utilizing the extensive PISTACHIO, BKMS_METABOLIC, PISTACHIO_RINGBREAKER, REAXYS, REAXYS_BIOCATALYSIS database, our tool offers high-accuracy predictions, reflecting the latest in chemical research and data.
Strategy Settings
Precursor scoring | Relevance Heuristic |
---|---|
Min. plausibility | 0.01 |
Model | Template_relevance |
Template Set | Pistachio/Bkms_metabolic/Pistachio_ringbreaker/Reaxys/Reaxys_biocatalysis |
Top-N result to add to graph | 6 |
Feasible Synthetic Routes
Haftungsausschluss und Informationen zu In-Vitro-Forschungsprodukten
Bitte beachten Sie, dass alle Artikel und Produktinformationen, die auf BenchChem präsentiert werden, ausschließlich zu Informationszwecken bestimmt sind. Die auf BenchChem zum Kauf angebotenen Produkte sind speziell für In-vitro-Studien konzipiert, die außerhalb lebender Organismen durchgeführt werden. In-vitro-Studien, abgeleitet von dem lateinischen Begriff "in Glas", beinhalten Experimente, die in kontrollierten Laborumgebungen unter Verwendung von Zellen oder Geweben durchgeführt werden. Es ist wichtig zu beachten, dass diese Produkte nicht als Arzneimittel oder Medikamente eingestuft sind und keine Zulassung der FDA für die Vorbeugung, Behandlung oder Heilung von medizinischen Zuständen, Beschwerden oder Krankheiten erhalten haben. Wir müssen betonen, dass jede Form der körperlichen Einführung dieser Produkte in Menschen oder Tiere gesetzlich strikt untersagt ist. Es ist unerlässlich, sich an diese Richtlinien zu halten, um die Einhaltung rechtlicher und ethischer Standards in Forschung und Experiment zu gewährleisten.