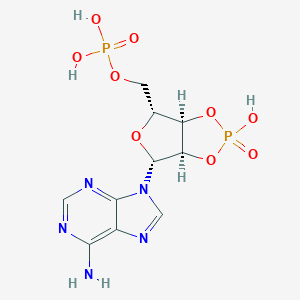
Adenosine-5'-phosphate-2',3'-cyclic phosphate
Übersicht
Beschreibung
Adenosine-5’-phosphate-2’,3’-cyclic phosphate is a form of an adenosine nucleotide with two phosphate groups attached to different carbons in the ribose ring . It has a role as an Escherichia coli metabolite .
Synthesis Analysis
Adenosine-3’,5’-bisphosphate is produced as a product of sulfotransferase enzymes from the donation of a sulfate group from the coenzyme 3’-phosphoadenosine-5’-phosphosulfate . There are also other synthesis methods such as the treatment of 2-aza-1, N6 -etheno- c -AMP with NBS or Br 2 under mild conditions .Molecular Structure Analysis
The molecular formula of Adenosine-5’-phosphate-2’,3’-cyclic phosphate is C10H13N5O9P2. It has an average mass of 409.186 Da and a monoisotopic mass of 409.018860 Da .Chemical Reactions Analysis
Adenosine Cyclic 2’‘,3’‘-Phosphate 5’‘-Phosphate can be used in biological study for 2’‘:3’'-cyclic PAP, sulfuryl group acceptor in a reaction catalyzed by phenol sulfotransferase . It can also be used in biological study for the effect of adenine nucleotides on myo-inositol-1,4,5-trisphosphate-induced calcium release .Physical And Chemical Properties Analysis
Adenosine-5’-phosphate-2’,3’-cyclic phosphate has a density of 2.6±0.1 g/cm3, a boiling point of 806.4±75.0 °C at 760 mmHg, and a vapor pressure of 0.0±3.0 mmHg at 25°C . It also has an enthalpy of vaporization of 122.9±3.0 kJ/mol and a flash point of 441.5±37.1 °C .Wissenschaftliche Forschungsanwendungen
Enzymatic Activity and Biological Roles
Adenosine-5'-phosphate-2',3'-cyclic phosphate plays a crucial role in biological systems. One of its primary functions is identified through the enzymatic activity of cyclic nucleotide phosphodiesterase. This enzyme is responsible for hydrolyzing adenosine 3',5'-phosphate, yielding adenosine 5'-phosphate, a process essential for controlling adenosine levels in biological systems (Butcher & Sutherland, 1962). Furthermore, the unique structural properties of adenosine 3',5'-cyclic phosphate have been elucidated through x-ray diffraction studies, providing insights into its role at the molecular level (Watenpaugh et al., 1968).
Role in Steroidogenesis and Enzyme Activation
Significantly, adenosine 3',5'-cyclic phosphate stimulates steroidogenesis in adrenal mitochondria, indicating its role in hormone production and regulation (Roberts et al., 1967). The compound also shows specificity in activating phosphorylase b kinase, an enzyme crucial in various metabolic pathways (Drummond & Powell, 1970).
Synthesis and Antitumor Activity
The synthesis and antitumor activity of adenosine 5'-phosphates and cyclic 3',5'-phosphates derived from biologically active nucleosides have been a focus of research, highlighting the potential therapeutic applications of these compounds (Hong et al., 1975).
Metabolic and Hormonal Effects
Adenosine 3',5'-cyclic phosphate is implicated in several metabolic processes, such as the inactivation of liver glycogen synthetase and mapping of binding sites on protein kinases, which are central to cellular regulation and signaling (Glinsmann & Hern, 1969); (Yagura & Miller, 1981).
Biochemical Analysis and Assay Development
Advancements in biochemical analysis include the development of a radioimmunoassay for adenosine 3',5'-cyclic phosphate, offering a sensitive method for measuring this nucleotide in tissue extracts (Steiner et al., 1969).
Wirkmechanismus
A 2’,3’-cyclic phosphate (cP) is one of the 3’-terminal forms of RNAs mainly generated from RNA cleavage by ribonucleases . Despite cP-RNAs’ invisibility in RNA-seq data, increasing evidence indicates that they are not accumulated simply as non-functional degradation products; rather, they have physiological roles in various biological processes .
Zukünftige Richtungen
Eigenschaften
IUPAC Name |
[(3aR,4R,6R,6aR)-4-(6-aminopurin-9-yl)-2-hydroxy-2-oxo-3a,4,6,6a-tetrahydrofuro[3,4-d][1,3,2]dioxaphosphol-6-yl]methyl dihydrogen phosphate | |
---|---|---|
Source | PubChem | |
URL | https://pubchem.ncbi.nlm.nih.gov | |
Description | Data deposited in or computed by PubChem | |
InChI |
InChI=1S/C10H13N5O9P2/c11-8-5-9(13-2-12-8)15(3-14-5)10-7-6(23-26(19,20)24-7)4(22-10)1-21-25(16,17)18/h2-4,6-7,10H,1H2,(H,19,20)(H2,11,12,13)(H2,16,17,18)/t4-,6-,7-,10-/m1/s1 | |
Source | PubChem | |
URL | https://pubchem.ncbi.nlm.nih.gov | |
Description | Data deposited in or computed by PubChem | |
InChI Key |
HJMBCNJTGVMDOA-KQYNXXCUSA-N | |
Source | PubChem | |
URL | https://pubchem.ncbi.nlm.nih.gov | |
Description | Data deposited in or computed by PubChem | |
Canonical SMILES |
C1=NC(=C2C(=N1)N(C=N2)C3C4C(C(O3)COP(=O)(O)O)OP(=O)(O4)O)N | |
Source | PubChem | |
URL | https://pubchem.ncbi.nlm.nih.gov | |
Description | Data deposited in or computed by PubChem | |
Isomeric SMILES |
C1=NC(=C2C(=N1)N(C=N2)[C@H]3[C@H]4[C@@H]([C@H](O3)COP(=O)(O)O)OP(=O)(O4)O)N | |
Source | PubChem | |
URL | https://pubchem.ncbi.nlm.nih.gov | |
Description | Data deposited in or computed by PubChem | |
Molecular Formula |
C10H13N5O9P2 | |
Source | PubChem | |
URL | https://pubchem.ncbi.nlm.nih.gov | |
Description | Data deposited in or computed by PubChem | |
Molecular Weight |
409.19 g/mol | |
Source | PubChem | |
URL | https://pubchem.ncbi.nlm.nih.gov | |
Description | Data deposited in or computed by PubChem | |
Product Name |
Adenosine-5'-phosphate-2',3'-cyclic phosphate |
Retrosynthesis Analysis
AI-Powered Synthesis Planning: Our tool employs the Template_relevance Pistachio, Template_relevance Bkms_metabolic, Template_relevance Pistachio_ringbreaker, Template_relevance Reaxys, Template_relevance Reaxys_biocatalysis model, leveraging a vast database of chemical reactions to predict feasible synthetic routes.
One-Step Synthesis Focus: Specifically designed for one-step synthesis, it provides concise and direct routes for your target compounds, streamlining the synthesis process.
Accurate Predictions: Utilizing the extensive PISTACHIO, BKMS_METABOLIC, PISTACHIO_RINGBREAKER, REAXYS, REAXYS_BIOCATALYSIS database, our tool offers high-accuracy predictions, reflecting the latest in chemical research and data.
Strategy Settings
Precursor scoring | Relevance Heuristic |
---|---|
Min. plausibility | 0.01 |
Model | Template_relevance |
Template Set | Pistachio/Bkms_metabolic/Pistachio_ringbreaker/Reaxys/Reaxys_biocatalysis |
Top-N result to add to graph | 6 |
Feasible Synthetic Routes
Haftungsausschluss und Informationen zu In-Vitro-Forschungsprodukten
Bitte beachten Sie, dass alle Artikel und Produktinformationen, die auf BenchChem präsentiert werden, ausschließlich zu Informationszwecken bestimmt sind. Die auf BenchChem zum Kauf angebotenen Produkte sind speziell für In-vitro-Studien konzipiert, die außerhalb lebender Organismen durchgeführt werden. In-vitro-Studien, abgeleitet von dem lateinischen Begriff "in Glas", beinhalten Experimente, die in kontrollierten Laborumgebungen unter Verwendung von Zellen oder Geweben durchgeführt werden. Es ist wichtig zu beachten, dass diese Produkte nicht als Arzneimittel oder Medikamente eingestuft sind und keine Zulassung der FDA für die Vorbeugung, Behandlung oder Heilung von medizinischen Zuständen, Beschwerden oder Krankheiten erhalten haben. Wir müssen betonen, dass jede Form der körperlichen Einführung dieser Produkte in Menschen oder Tiere gesetzlich strikt untersagt ist. Es ist unerlässlich, sich an diese Richtlinien zu halten, um die Einhaltung rechtlicher und ethischer Standards in Forschung und Experiment zu gewährleisten.