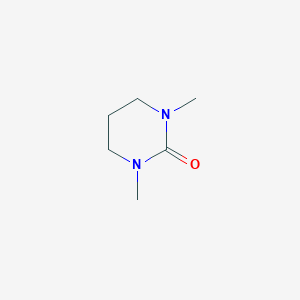
1,3-Dimethyl-3,4,5,6-tetrahydro-2(1H)-pyrimidinone
Übersicht
Beschreibung
1,3-Dimethyl-3,4,5,6-tetrahydro-2(1H)-pyrimidinone, also known as DMPU, is a compound with the empirical formula C6H12N2O . It is often used as a versatile solvent in various applications such as the N-alkylation of amines, the O-alkylation of aldoses, and the synthesis of poly(arylethers) .
Molecular Structure Analysis
The molecular structure of 1,3-Dimethyl-3,4,5,6-tetrahydro-2(1H)-pyrimidinone involves a pyrimidinone ring with two methyl groups attached to the nitrogen atoms . The SMILES string representation of the molecule is CN1CCCN©C1=O .Chemical Reactions Analysis
1,3-Dimethyl-3,4,5,6-tetrahydro-2(1H)-pyrimidinone (DMPU) is used as a solvent in various chemical reactions. For instance, it is used in the N-alkylation of amines, the O-alkylation of aldoses, and the synthesis of poly(arylethers) . It is also used as a urea solvent in the synthesis of 1-Aryl-3,4,5-substituted pyrazoles through the cyclocondensation of arylhydrazin hydrochlorides with 1,3-diketones .Physical And Chemical Properties Analysis
1,3-Dimethyl-3,4,5,6-tetrahydro-2(1H)-pyrimidinone is a liquid at room temperature with a density of 1.06 g/mL at 25 °C . It has a boiling point of 146 °C at 44 mmHg .Wissenschaftliche Forschungsanwendungen
Peptide Synthesis
DMPU is utilized in peptide synthesis due to its ability to dissolve both organic and inorganic compounds. It acts as a solvent that can facilitate the coupling of amino acids to form peptides .
N-Alkylation Reactions
In the field of organic synthesis, DMPU serves as a solvent for N-alkylation reactions. This process is crucial for modifying the structure of amines, which are fundamental building blocks in the synthesis of various pharmaceuticals .
O-Alkylation of Carbohydrates
DMPU is involved in the O-alkylation of carbohydrates. This reaction is important for the modification of sugars, which can lead to the development of new therapeutic agents .
Synthesis of Poly(Aryl Ether)
The compound is used as a solvent in the synthesis of poly(aryl ether), a type of polymer known for its thermal stability and mechanical strength, making it suitable for high-performance applications .
Synthesis of 1-Aryl-3,4,5-Substituted Pyrazoles
DMPU acts as a urea solvent in the synthesis of 1-aryl-3,4,5-substituted pyrazoles through the reaction of hydrazine hydrochloride salts with 1,3-diketones. Pyrazoles have significant pharmacological properties .
Additive in Asymmetric Cyanohydrin Synthesis
It is used as an additive in the synthesis of asymmetric cyanohydrins, which are valuable intermediates in the production of α-hydroxy acids, amino acids, and their derivatives .
Solvent for Prins Fluorination
DMPU, in combination with HF, serves as a nucleophilic fluorinating agent in Prins fluorination reactions to synthesize substituted 4-fluorotetrahydropyrans and 4-fluoropiperidines .
Green Chemistry Applications
DMPU is recognized for its role in green chemistry, where it is used as a solvent in solvent-free conditions at room temperature via grinding. This method is environmentally friendly and efficient for synthesizing dihydropyrimidin-2(1H)-ones .
Wirkmechanismus
Target of Action
1,3-Dimethyl-3,4,5,6-tetrahydro-2(1H)-pyrimidinone, also known as 1,3-dimethyltetrahydropyrimidin-2(1H)-one, is a versatile solvent used in various chemical reactions . The primary targets of this compound are the reactants in the chemical reactions where it is used as a solvent.
Mode of Action
This compound interacts with its targets by facilitating chemical reactions. It is used as a solvent in the N-alkylation of amines, O-alkylation of aldoses, and synthesis of poly(arylethers) . It also acts as a urea solvent in the synthesis of 1-aryl-3,4,5-substituted pyrazoles through the cyclocondensation of arylhydrazine hydrochlorides with 1,3-diketones .
Pharmacokinetics
Its properties such as boiling point (146 °c/44 mmhg) and density (1.06 g/mL at 25 °C) can affect its behavior and efficacy as a solvent.
Action Environment
Environmental factors such as temperature and pressure can influence the action, efficacy, and stability of 1,3-Dimethyl-3,4,5,6-tetrahydro-2(1H)-pyrimidinone as a solvent. For example, its boiling point indicates that it can be used in reactions requiring elevated temperatures .
Eigenschaften
IUPAC Name |
1,3-dimethyl-1,3-diazinan-2-one | |
---|---|---|
Source | PubChem | |
URL | https://pubchem.ncbi.nlm.nih.gov | |
Description | Data deposited in or computed by PubChem | |
InChI |
InChI=1S/C6H12N2O/c1-7-4-3-5-8(2)6(7)9/h3-5H2,1-2H3 | |
Source | PubChem | |
URL | https://pubchem.ncbi.nlm.nih.gov | |
Description | Data deposited in or computed by PubChem | |
InChI Key |
GUVUOGQBMYCBQP-UHFFFAOYSA-N | |
Source | PubChem | |
URL | https://pubchem.ncbi.nlm.nih.gov | |
Description | Data deposited in or computed by PubChem | |
Canonical SMILES |
CN1CCCN(C1=O)C | |
Source | PubChem | |
URL | https://pubchem.ncbi.nlm.nih.gov | |
Description | Data deposited in or computed by PubChem | |
Molecular Formula |
C6H12N2O | |
Source | PubChem | |
URL | https://pubchem.ncbi.nlm.nih.gov | |
Description | Data deposited in or computed by PubChem | |
DSSTOX Substance ID |
DTXSID3074575 | |
Record name | 2(1H)-Pyrimidinone, tetrahydro-1,3-dimethyl- | |
Source | EPA DSSTox | |
URL | https://comptox.epa.gov/dashboard/DTXSID3074575 | |
Description | DSSTox provides a high quality public chemistry resource for supporting improved predictive toxicology. | |
Molecular Weight |
128.17 g/mol | |
Source | PubChem | |
URL | https://pubchem.ncbi.nlm.nih.gov | |
Description | Data deposited in or computed by PubChem | |
Physical Description |
Colorless odorless liquid; Hygroscopic; [Alfa Aesar MSDS] | |
Record name | Tetrahydro-1,3-dimethyl-1H-pyrimidin-2-one | |
Source | Haz-Map, Information on Hazardous Chemicals and Occupational Diseases | |
URL | https://haz-map.com/Agents/19256 | |
Description | Haz-Map® is an occupational health database designed for health and safety professionals and for consumers seeking information about the adverse effects of workplace exposures to chemical and biological agents. | |
Explanation | Copyright (c) 2022 Haz-Map(R). All rights reserved. Unless otherwise indicated, all materials from Haz-Map are copyrighted by Haz-Map(R). No part of these materials, either text or image may be used for any purpose other than for personal use. Therefore, reproduction, modification, storage in a retrieval system or retransmission, in any form or by any means, electronic, mechanical or otherwise, for reasons other than personal use, is strictly prohibited without prior written permission. | |
Product Name |
1,3-Dimethyl-3,4,5,6-tetrahydro-2(1H)-pyrimidinone | |
CAS RN |
7226-23-5 | |
Record name | 1,3-Dimethyl-3,4,5,6-tetrahydro-2(1H)-pyrimidinone | |
Source | CAS Common Chemistry | |
URL | https://commonchemistry.cas.org/detail?cas_rn=7226-23-5 | |
Description | CAS Common Chemistry is an open community resource for accessing chemical information. Nearly 500,000 chemical substances from CAS REGISTRY cover areas of community interest, including common and frequently regulated chemicals, and those relevant to high school and undergraduate chemistry classes. This chemical information, curated by our expert scientists, is provided in alignment with our mission as a division of the American Chemical Society. | |
Explanation | The data from CAS Common Chemistry is provided under a CC-BY-NC 4.0 license, unless otherwise stated. | |
Record name | 2(1H)-Pyrimidinone, tetrahydro-1,3-dimethyl- | |
Source | ChemIDplus | |
URL | https://pubchem.ncbi.nlm.nih.gov/substance/?source=chemidplus&sourceid=0007226235 | |
Description | ChemIDplus is a free, web search system that provides access to the structure and nomenclature authority files used for the identification of chemical substances cited in National Library of Medicine (NLM) databases, including the TOXNET system. | |
Record name | 2(1H)-Pyrimidinone, tetrahydro-1,3-dimethyl- | |
Source | EPA Chemicals under the TSCA | |
URL | https://www.epa.gov/chemicals-under-tsca | |
Description | EPA Chemicals under the Toxic Substances Control Act (TSCA) collection contains information on chemicals and their regulations under TSCA, including non-confidential content from the TSCA Chemical Substance Inventory and Chemical Data Reporting. | |
Record name | 2(1H)-Pyrimidinone, tetrahydro-1,3-dimethyl- | |
Source | EPA DSSTox | |
URL | https://comptox.epa.gov/dashboard/DTXSID3074575 | |
Description | DSSTox provides a high quality public chemistry resource for supporting improved predictive toxicology. | |
Record name | Tetrahydro-1,3-dimethyl-1H-pyrimidin-2-one | |
Source | European Chemicals Agency (ECHA) | |
URL | https://echa.europa.eu/substance-information/-/substanceinfo/100.027.841 | |
Description | The European Chemicals Agency (ECHA) is an agency of the European Union which is the driving force among regulatory authorities in implementing the EU's groundbreaking chemicals legislation for the benefit of human health and the environment as well as for innovation and competitiveness. | |
Explanation | Use of the information, documents and data from the ECHA website is subject to the terms and conditions of this Legal Notice, and subject to other binding limitations provided for under applicable law, the information, documents and data made available on the ECHA website may be reproduced, distributed and/or used, totally or in part, for non-commercial purposes provided that ECHA is acknowledged as the source: "Source: European Chemicals Agency, http://echa.europa.eu/". Such acknowledgement must be included in each copy of the material. ECHA permits and encourages organisations and individuals to create links to the ECHA website under the following cumulative conditions: Links can only be made to webpages that provide a link to the Legal Notice page. | |
Retrosynthesis Analysis
AI-Powered Synthesis Planning: Our tool employs the Template_relevance Pistachio, Template_relevance Bkms_metabolic, Template_relevance Pistachio_ringbreaker, Template_relevance Reaxys, Template_relevance Reaxys_biocatalysis model, leveraging a vast database of chemical reactions to predict feasible synthetic routes.
One-Step Synthesis Focus: Specifically designed for one-step synthesis, it provides concise and direct routes for your target compounds, streamlining the synthesis process.
Accurate Predictions: Utilizing the extensive PISTACHIO, BKMS_METABOLIC, PISTACHIO_RINGBREAKER, REAXYS, REAXYS_BIOCATALYSIS database, our tool offers high-accuracy predictions, reflecting the latest in chemical research and data.
Strategy Settings
Precursor scoring | Relevance Heuristic |
---|---|
Min. plausibility | 0.01 |
Model | Template_relevance |
Template Set | Pistachio/Bkms_metabolic/Pistachio_ringbreaker/Reaxys/Reaxys_biocatalysis |
Top-N result to add to graph | 6 |
Feasible Synthetic Routes
Haftungsausschluss und Informationen zu In-Vitro-Forschungsprodukten
Bitte beachten Sie, dass alle Artikel und Produktinformationen, die auf BenchChem präsentiert werden, ausschließlich zu Informationszwecken bestimmt sind. Die auf BenchChem zum Kauf angebotenen Produkte sind speziell für In-vitro-Studien konzipiert, die außerhalb lebender Organismen durchgeführt werden. In-vitro-Studien, abgeleitet von dem lateinischen Begriff "in Glas", beinhalten Experimente, die in kontrollierten Laborumgebungen unter Verwendung von Zellen oder Geweben durchgeführt werden. Es ist wichtig zu beachten, dass diese Produkte nicht als Arzneimittel oder Medikamente eingestuft sind und keine Zulassung der FDA für die Vorbeugung, Behandlung oder Heilung von medizinischen Zuständen, Beschwerden oder Krankheiten erhalten haben. Wir müssen betonen, dass jede Form der körperlichen Einführung dieser Produkte in Menschen oder Tiere gesetzlich strikt untersagt ist. Es ist unerlässlich, sich an diese Richtlinien zu halten, um die Einhaltung rechtlicher und ethischer Standards in Forschung und Experiment zu gewährleisten.