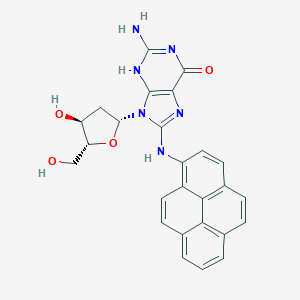
N-(Deoxyguanosin-8-yl)-1-aminopyrene
Übersicht
Beschreibung
N-(Deoxyguanosin-8-yl)-1-aminopyrene: is a DNA adduct formed when the carcinogenic compound 1-aminopyrene reacts with the DNA base guanine. This compound is significant in the study of mutagenesis and carcinogenesis because it represents a type of DNA damage that can lead to mutations if not properly repaired.
Vorbereitungsmethoden
Synthetic Routes and Reaction Conditions: The synthesis of N-(Deoxyguanosin-8-yl)-1-aminopyrene typically involves the reaction of 1-aminopyrene with deoxyguanosine. This reaction can be facilitated by using an activated form of 1-aminopyrene, such as N-acetoxy-1-aminopyrene, which reacts with the guanine base in DNA to form the adduct .
Industrial Production Methods: Industrial production methods for this compound are not well-documented, as it is primarily synthesized for research purposes rather than commercial production. The synthesis is usually carried out in specialized laboratories equipped to handle carcinogenic substances.
Analyse Chemischer Reaktionen
Types of Reactions: N-(Deoxyguanosin-8-yl)-1-aminopyrene can undergo various chemical reactions, including:
Oxidation: This reaction can lead to the formation of oxidized derivatives of the compound.
Reduction: Reduction reactions can modify the aminopyrene moiety.
Substitution: Substitution reactions can occur at the amino group or other reactive sites on the molecule.
Common Reagents and Conditions:
Oxidation: Common oxidizing agents include hydrogen peroxide and potassium permanganate.
Reduction: Reducing agents such as sodium borohydride or lithium aluminum hydride can be used.
Substitution: Conditions for substitution reactions vary depending on the desired product but often involve nucleophilic or electrophilic reagents.
Major Products: The major products formed from these reactions depend on the specific conditions and reagents used. For example, oxidation can lead to the formation of hydroxylated derivatives, while reduction can yield deaminated products.
Wissenschaftliche Forschungsanwendungen
N-(Deoxyguanosin-8-yl)-1-aminopyrene is used extensively in scientific research, particularly in the fields of:
Chemistry: Studying the chemical properties and reactivity of DNA adducts.
Biology: Investigating the biological effects of DNA damage and the mechanisms of DNA repair.
Medicine: Understanding the role of DNA adducts in carcinogenesis and developing potential therapeutic interventions.
Industry: Assessing the genotoxicity of environmental pollutants and industrial chemicals.
Wirkmechanismus
The mechanism by which N-(Deoxyguanosin-8-yl)-1-aminopyrene exerts its effects involves the formation of a covalent bond between the aminopyrene moiety and the guanine base in DNA. This adduct can cause distortions in the DNA helix, leading to errors during DNA replication and transcription. The primary molecular targets are the DNA repair enzymes that recognize and remove these adducts to prevent mutations .
Vergleich Mit ähnlichen Verbindungen
N-(Deoxyguanosin-8-yl)-2-aminofluorene: Another DNA adduct formed by the reaction of 2-aminofluorene with deoxyguanosine.
N-(Deoxyguanosin-8-yl)-2-acetylaminofluorene: Formed by the reaction of 2-acetylaminofluorene with deoxyguanosine.
Uniqueness: N-(Deoxyguanosin-8-yl)-1-aminopyrene is unique due to its specific structure and the particular carcinogenic properties of 1-aminopyrene. This compound is especially relevant in studies of environmental carcinogens, as 1-aminopyrene is a common pollutant found in diesel exhaust and other sources of combustion .
Eigenschaften
IUPAC Name |
2-amino-9-[(2R,4S,5R)-4-hydroxy-5-(hydroxymethyl)oxolan-2-yl]-8-(pyren-1-ylamino)-1H-purin-6-one | |
---|---|---|
Source | PubChem | |
URL | https://pubchem.ncbi.nlm.nih.gov | |
Description | Data deposited in or computed by PubChem | |
InChI |
InChI=1S/C26H22N6O4/c27-25-30-23-22(24(35)31-25)29-26(32(23)19-10-17(34)18(11-33)36-19)28-16-9-7-14-5-4-12-2-1-3-13-6-8-15(16)21(14)20(12)13/h1-9,17-19,33-34H,10-11H2,(H,28,29)(H3,27,30,31,35)/t17-,18+,19+/m0/s1 | |
Source | PubChem | |
URL | https://pubchem.ncbi.nlm.nih.gov | |
Description | Data deposited in or computed by PubChem | |
InChI Key |
QBMJHUSBJZIUSS-IPMKNSEASA-N | |
Source | PubChem | |
URL | https://pubchem.ncbi.nlm.nih.gov | |
Description | Data deposited in or computed by PubChem | |
Canonical SMILES |
C1C(C(OC1N2C3=C(C(=O)NC(=N3)N)N=C2NC4=C5C=CC6=CC=CC7=C6C5=C(C=C7)C=C4)CO)O | |
Source | PubChem | |
URL | https://pubchem.ncbi.nlm.nih.gov | |
Description | Data deposited in or computed by PubChem | |
Isomeric SMILES |
C1[C@@H]([C@H](O[C@H]1N2C3=C(C(=O)NC(=N3)N)N=C2NC4=C5C=CC6=CC=CC7=C6C5=C(C=C7)C=C4)CO)O | |
Source | PubChem | |
URL | https://pubchem.ncbi.nlm.nih.gov | |
Description | Data deposited in or computed by PubChem | |
Molecular Formula |
C26H22N6O4 | |
Source | PubChem | |
URL | https://pubchem.ncbi.nlm.nih.gov | |
Description | Data deposited in or computed by PubChem | |
DSSTOX Substance ID |
DTXSID201006568 | |
Record name | 9-(2-Deoxypentofuranosyl)-2-imino-8-[(pyren-1-yl)imino]-3,7,8,9-tetrahydro-2H-purin-6-ol | |
Source | EPA DSSTox | |
URL | https://comptox.epa.gov/dashboard/DTXSID201006568 | |
Description | DSSTox provides a high quality public chemistry resource for supporting improved predictive toxicology. | |
Molecular Weight |
482.5 g/mol | |
Source | PubChem | |
URL | https://pubchem.ncbi.nlm.nih.gov | |
Description | Data deposited in or computed by PubChem | |
CAS No. |
85989-43-1 | |
Record name | N-(Deoxyguanosin-8-yl)-1-aminopyrene | |
Source | ChemIDplus | |
URL | https://pubchem.ncbi.nlm.nih.gov/substance/?source=chemidplus&sourceid=0085989431 | |
Description | ChemIDplus is a free, web search system that provides access to the structure and nomenclature authority files used for the identification of chemical substances cited in National Library of Medicine (NLM) databases, including the TOXNET system. | |
Record name | 9-(2-Deoxypentofuranosyl)-2-imino-8-[(pyren-1-yl)imino]-3,7,8,9-tetrahydro-2H-purin-6-ol | |
Source | EPA DSSTox | |
URL | https://comptox.epa.gov/dashboard/DTXSID201006568 | |
Description | DSSTox provides a high quality public chemistry resource for supporting improved predictive toxicology. | |
Retrosynthesis Analysis
AI-Powered Synthesis Planning: Our tool employs the Template_relevance Pistachio, Template_relevance Bkms_metabolic, Template_relevance Pistachio_ringbreaker, Template_relevance Reaxys, Template_relevance Reaxys_biocatalysis model, leveraging a vast database of chemical reactions to predict feasible synthetic routes.
One-Step Synthesis Focus: Specifically designed for one-step synthesis, it provides concise and direct routes for your target compounds, streamlining the synthesis process.
Accurate Predictions: Utilizing the extensive PISTACHIO, BKMS_METABOLIC, PISTACHIO_RINGBREAKER, REAXYS, REAXYS_BIOCATALYSIS database, our tool offers high-accuracy predictions, reflecting the latest in chemical research and data.
Strategy Settings
Precursor scoring | Relevance Heuristic |
---|---|
Min. plausibility | 0.01 |
Model | Template_relevance |
Template Set | Pistachio/Bkms_metabolic/Pistachio_ringbreaker/Reaxys/Reaxys_biocatalysis |
Top-N result to add to graph | 6 |
Feasible Synthetic Routes
A: N-(Deoxyguanosin-8-yl)-1-aminopyrene (dG(AP)), primarily interacts with DNA by forming a covalent bond at the C8 position of guanine. This adduct formation significantly disrupts the DNA structure, leading to various downstream effects:
- Replication blockage: DNA polymerases often stall at or near the dG(AP) adduct, hindering DNA replication. This blockage can trigger cellular responses such as DNA repair or apoptosis.
- Mutations: The presence of dG(AP) can cause misincorporation of nucleotides during DNA replication, leading to mutations. The type and frequency of mutations depend on various factors including the sequence context surrounding the adduct, the specific DNA polymerase involved, and the cellular repair machinery. Frameshift mutations, particularly -1 frameshifts involving the deletion of a neighboring cytosine, are frequently observed.
- Genomic instability: The accumulation of unrepaired dG(AP) adducts can contribute to genomic instability, potentially leading to cell transformation and cancer.
ANone:
- HPLC: High-Performance Liquid Chromatography, utilized for separation and identification of dG(AP) from other DNA adducts. Retention times and characteristic elution profiles are crucial for identification.
- NMR: Nuclear Magnetic Resonance, used to elucidate the structure and conformation of dG(AP) within a DNA duplex. Chemical shifts, coupling constants, and NOE (Nuclear Overhauser Effect) patterns provide valuable structural insights.
- UV-Vis Spectroscopy: Used to study the interaction of the aminopyrene moiety with DNA bases. Changes in absorbance and shifts in absorption maxima provide information about adduct formation and potential conformational changes.
- Temperature: Increased temperatures can lead to destabilization of the DNA duplex containing dG(AP), as evidenced by thermal melting studies. The extent of destabilization depends on factors like the base opposite the adduct and the surrounding sequence.
- Enzymes: The dG(AP) adduct can be removed from DNA by specific DNA repair enzymes. Additionally, enzymes like nuclease P1, which cleaves single-stranded DNA, can be used to differentiate dG(AP) from other adducts based on their susceptibility to enzymatic digestion.
ANone: dG(AP) is generated through a series of metabolic reactions involving the environmental contaminant 1-nitropyrene (1-NP):
- Metabolic Activation: 1-NP itself is not directly reactive with DNA. It requires metabolic activation, primarily through nitroreduction.
- Nitroreduction: Enzymes like bacterial nitroreductases or mammalian enzymes such as xanthine oxidase catalyze the reduction of the nitro group on 1-NP. This process generates the reactive intermediate, N-hydroxy-1-aminopyrene.
- Adduct Formation: N-hydroxy-1-aminopyrene, being electrophilic, can readily react with the electron-rich C8 position of guanine in DNA to form the stable dG(AP) adduct.
ANone: Computational chemistry techniques are instrumental in providing a deeper understanding of dG(AP) behavior at the molecular level:
- Molecular Dynamics (MD) simulations: MD simulations help visualize and analyze the structural and dynamic consequences of dG(AP) incorporation into DNA. These simulations provide insights into how the adduct alters DNA conformation, flexibility, and interactions with other molecules, including DNA polymerases.
- Energy Minimizations: These calculations are used to identify the most stable conformations of dG(AP) within a DNA duplex. Understanding the preferred adduct conformation is crucial for predicting its impact on DNA replication and repair processes.
- Docking studies: Computational docking methods can be used to model the interaction of dG(AP)-containing DNA with DNA polymerases or repair enzymes. This can help explain the observed differences in bypass efficiency and fidelity among different polymerases.
- QSAR (Quantitative Structure-Activity Relationship) models: QSAR approaches correlate the structural features of dG(AP) and related compounds with their observed biological activities, such as mutagenicity or cytotoxicity. These models can be helpful in predicting the potential toxicity of other aromatic amines.
ANone: The structure of dG(AP) plays a critical role in its biological activity. The bulky, aromatic 1-aminopyrene moiety attached to the guanine base is a key determinant of its effects:
- Bulky Adduct: The size and rigidity of the 1-aminopyrene moiety distort the normal helical structure of DNA. This distortion interferes with DNA replication by hindering the progression of DNA polymerases. The extent of distortion and replication blockage varies depending on the adduct's conformation, which can be influenced by the surrounding DNA sequence and the base opposite the adduct.
- Intercalation: The planar, aromatic nature of the 1-aminopyrene moiety allows it to intercalate between DNA base pairs, further stabilizing the adduct within the DNA helix. This intercalation can enhance the mutagenic potential of dG(AP) as it can promote misincorporation of bases during DNA replication.
- Size and Shape: Smaller aromatic amines, like 2-aminofluorene, form less bulky adducts that are less disruptive to DNA structure and hence, less mutagenic. Conversely, larger or more rigid aromatic systems might lead to greater distortion and enhanced mutagenicity.
ANone: The study of dG(AP) is crucial in various research areas, including:
- Environmental Toxicology: Researchers investigate the presence and levels of dG(AP) in biological samples as a biomarker of exposure to 1-NP, a common environmental pollutant found in diesel exhaust and other combustion products.
- Chemical Carcinogenesis: Scientists study the formation, repair, and mutagenic potential of dG(AP) to understand the mechanisms by which 1-NP and related compounds contribute to cancer development.
- DNA Replication and Repair: dG(AP) serves as a valuable tool to investigate the mechanisms of DNA replication fidelity and the cellular responses to bulky DNA lesions. Researchers study how different DNA polymerases and repair enzymes process dG(AP) to gain insights into these fundamental cellular processes.
- 32P-Postlabeling: This highly sensitive method is widely used to detect and quantify dG(AP) and other DNA adducts in various biological samples. It involves enzymatic digestion of DNA, followed by labeling of the adducted nucleotides with radioactive phosphorus-32. The labeled adducts are then separated and visualized using thin-layer chromatography.
- HPLC with various detection methods: HPLC, often coupled with UV, fluorescence, or mass spectrometric detection, is used to separate and identify dG(AP) from other DNA adducts and normal nucleotides. Characteristic retention times and spectral properties help confirm its identity and quantify its levels.
- Mass spectrometry (MS): MS techniques can provide definitive identification of dG(AP) based on its mass-to-charge ratio. This method can be particularly useful for characterizing the adduct in complex biological matrices.
ANone: Yes, although dG(AP) is a major and well-studied DNA adduct of 1-NP, alternative approaches exist to assess 1-NP exposure and its biological effects:
- Hemoglobin Adducts: Stable adducts formed between 1-NP metabolites and hemoglobin can serve as dosimeters for 1-NP exposure. These adducts are relatively long-lived compared to DNA adducts and can reflect cumulative exposure over a longer period.
ANone: Not necessarily. While dG(AP) is a potent blocking lesion for DNA polymerases and has a strong potential to induce mutations, several factors influence whether its formation will ultimately result in a mutation:
- DNA Repair: Cells possess sophisticated DNA repair pathways, such as Nucleotide Excision Repair (NER), that can recognize and remove dG(AP) from DNA. If repair occurs efficiently before DNA replication, the adduct will not lead to a mutation.
- Translesion Synthesis: When dG(AP) persists during DNA replication, specific DNA polymerases, often belonging to the Y-family, can carry out translesion synthesis (TLS) past the lesion. While TLS allows for complete replication, these polymerases often have lower fidelity compared to replicative polymerases, increasing the chance of misincorporation. The specific polymerase involved influences the types and frequencies of mutations.
- Sequence Context: The DNA sequence surrounding the dG(AP) adduct influences its conformation, the efficiency of repair processes, and the fidelity of TLS. Certain sequences may favor error-free bypass, while others are more prone to specific types of mutations.
Haftungsausschluss und Informationen zu In-Vitro-Forschungsprodukten
Bitte beachten Sie, dass alle Artikel und Produktinformationen, die auf BenchChem präsentiert werden, ausschließlich zu Informationszwecken bestimmt sind. Die auf BenchChem zum Kauf angebotenen Produkte sind speziell für In-vitro-Studien konzipiert, die außerhalb lebender Organismen durchgeführt werden. In-vitro-Studien, abgeleitet von dem lateinischen Begriff "in Glas", beinhalten Experimente, die in kontrollierten Laborumgebungen unter Verwendung von Zellen oder Geweben durchgeführt werden. Es ist wichtig zu beachten, dass diese Produkte nicht als Arzneimittel oder Medikamente eingestuft sind und keine Zulassung der FDA für die Vorbeugung, Behandlung oder Heilung von medizinischen Zuständen, Beschwerden oder Krankheiten erhalten haben. Wir müssen betonen, dass jede Form der körperlichen Einführung dieser Produkte in Menschen oder Tiere gesetzlich strikt untersagt ist. Es ist unerlässlich, sich an diese Richtlinien zu halten, um die Einhaltung rechtlicher und ethischer Standards in Forschung und Experiment zu gewährleisten.