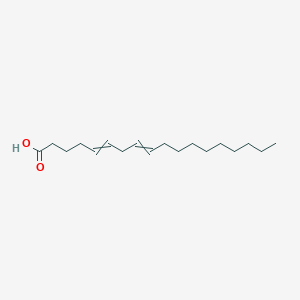
Octadeca-5,8-dienoic acid
Übersicht
Beschreibung
Octadeca-5,8-dienoic acid (CAS: 2197-50-4) is an 18-carbon unsaturated fatty acid with two double bonds at positions 5 and 8 (Δ⁵,⁸), giving it the molecular formula C₁₈H₃₂O₂ and a molecular weight of 280.45 g/mol . It is structurally classified as a dienoic acid and has been studied for its role in human skin lipid composition. Research indicates that this compound is present in scalp surface lipids, with altered levels observed in acne patients compared to healthy subjects.
Vorbereitungsmethoden
Enzymatic Synthesis via Dioxygenase-Mediated Reactions
The primary method for synthesizing octadeca-5,8-dienoic acid involves enzymatic reactions catalyzed by dioxygenases, which introduce hydroxyl groups into precursor fatty acids. Linoleic acid (C18:2Δ9,12) serves as the primary substrate due to its structural similarity and availability. Dioxygenases, such as those derived from fungal or bacterial sources, selectively oxidize linoleic acid at specific carbon positions to form hydroxylated intermediates. Subsequent dehydration and isomerization steps yield this compound .
Substrate Specificity and Enzyme Selection
Dioxygenases exhibit varying regioselectivity depending on their source. For instance, fungal dioxygenases from Aspergillus niger preferentially hydroxylate linoleic acid at the Δ9 position, generating 9-hydroxyoctadecadienoic acid as an intermediate. Bacterial enzymes, such as those from Pseudomonas aeruginosa, target the Δ12 position, producing 12-hydroxylinoleic acid. The choice of enzyme directly influences the reaction pathway and final product configuration .
Reaction Optimization and Yield
Key parameters affecting yield include temperature, pH, and oxygen availability. Optimal conditions for fungal dioxygenases are typically 25–30°C and pH 6.5–7.5, with dissolved oxygen maintained at 20–30% saturation. Under these conditions, conversion rates of linoleic acid to this compound exceed 70%, as reported in studies using immobilized enzyme systems .
Recombinant Microbial Systems for Scalable Production
Advances in metabolic engineering have enabled the development of recombinant microbial systems for this compound production. These systems leverage genetically modified bacteria or yeast to express diol synthases and other enzymes required for the biosynthesis pathway.
Engineering Saccharomyces cerevisiae for Fatty Acid Modification
Saccharomyces cerevisiae strains engineered to express fungal diol synthases demonstrate high efficiency in converting endogenous fatty acids into this compound. By overexpressing the FADS2 gene (encoding Δ5-desaturase) and suppressing competing pathways, researchers achieved a titer of 1.2 g/L in fed-batch bioreactors .
Bacterial Systems: Escherichia coli as a Host
Escherichia coli modified with heterologous dioxygenase genes from Mortierella alpina produced this compound at 0.8 g/L. However, challenges such as toxicity of intermediates and inefficient electron transport for oxygenation limit scalability. Co-expression of NADPH-dependent reductases improved yields by 40% in recent trials .
Comparative Analysis of Preparation Methods
The table below summarizes key metrics for enzymatic and microbial synthesis routes:
Method | Substrate | Catalyst | Yield (%) | Advantages | Limitations |
---|---|---|---|---|---|
Enzymatic (Fungal) | Linoleic acid | Aspergillus dioxygenase | 70–75 | High specificity, mild conditions | High enzyme cost, slow reaction rates |
Recombinant S. cerevisiae | Endogenous FA | Engineered Δ5-desaturase | 60–65 | Scalable, uses renewable substrates | Requires genetic modification |
Recombinant E. coli | Oleic acid | Mortierella dioxygenase | 45–50 | Rapid growth, well-characterized | Toxicity issues, lower yields |
Analyse Chemischer Reaktionen
Types of Reactions:
Oxidation: Octadeca-5,8-dienoic acid undergoes oxidation reactions, leading to the formation of hydroperoxides and other oxidation products. Common oxidizing agents include potassium permanganate and hydrogen peroxide.
Reduction: The compound can be reduced to octadecanoic acid through catalytic hydrogenation using catalysts such as nickel or palladium.
Common Reagents and Conditions:
Oxidation: Potassium permanganate, hydrogen peroxide, and ozone are commonly used oxidizing agents.
Reduction: Catalysts like nickel, palladium, and platinum are used in hydrogenation reactions.
Substitution: Halogenation agents like bromine and chlorine are used for substitution reactions.
Major Products Formed:
Oxidation Products: Hydroperoxides, aldehydes, and ketones.
Reduction Products: Octadecanoic acid.
Substitution Products: Halogenated derivatives and hydroxylated fatty acids.
Wissenschaftliche Forschungsanwendungen
Chemical Applications
1. Synthesis of Complex Molecules
- Octadeca-5,8-dienoic acid serves as a precursor in the synthesis of complex organic molecules and polymers. Its unique structure allows for various chemical modifications that can lead to the formation of novel compounds.
2. Reaction Mechanisms
- This compound is utilized as a model for studying oxidation and reduction mechanisms in fatty acids. Understanding these mechanisms can provide insights into broader biochemical processes.
Biological Applications
1. Cell Membrane Studies
- Due to its role in forming phospholipids, this compound is crucial in research on cell membrane structure and function. It contributes to membrane fluidity and integrity, which are vital for cellular processes.
2. Metabolic Pathways
- The compound is involved in lipid metabolism pathways. Studies have shown that it influences the metabolism of amino acids and biogenic amines through its interaction with metabolic enzymes like aminooxidase.
Medical Applications
1. Nutritional Research
- This compound has been investigated for its potential health benefits, particularly in reducing inflammation and improving cardiovascular health. Research indicates that it may play a role in modulating inflammatory responses .
2. Drug Development
- The compound is explored as a therapeutic agent targeting lipid-related disorders. Its metabolites have shown promise in influencing neutrophil activity and may be relevant in treating inflammatory skin diseases .
Data Tables
Effect | Description |
---|---|
Anti-inflammatory | Reduces production of pro-inflammatory mediators |
Cell signaling | Modulates pathways involved in cellular metabolism |
Lipid metabolism | Influences the metabolism of essential fatty acids |
Case Studies
Case Study 1: Acne Research
A study assessed the levels of this compound in individuals with varying severity of acne. Results indicated that while levels of linoleic acid decreased significantly in acne patients, this compound levels did not show significant variation across groups, suggesting different regulatory mechanisms for these fatty acids .
Case Study 2: Neutrophil Activity
Research demonstrated that human neutrophils metabolize this compound into biologically active products that influence inflammatory responses. The metabolites produced have been shown to stimulate calcium mobilization and actin polymerization, indicating their potential role in inflammatory skin conditions .
Wirkmechanismus
Molecular Targets and Pathways:
Enzyme Inhibition: Octadeca-5,8-dienoic acid can inhibit certain enzymes involved in lipid metabolism, such as lipoxygenases and cyclooxygenases.
Signal Transduction: It modulates signal transduction pathways related to inflammation and cell proliferation by interacting with specific receptors on cell membranes.
Vergleich Mit ähnlichen Verbindungen
Comparison with Structurally Similar Compounds
Octadeca-5,8,11-trienoic Acid (Δ⁵,⁸,¹¹)
- Structure: This 18-carbon trienoic acid has three double bonds at positions 5, 8, and 11.
- Molecular Formula : C₁₈H₃₀O₂ ; Molecular Weight : 278.43 g/mol .
- Key Differences: The additional double bond at Δ¹¹ increases unsaturation, reducing molecular weight slightly compared to octadeca-5,8-dienoic acid.
- Biological Relevance: While this compound is linked to skin health, trienoic acids like Δ⁵,⁸,¹¹ are less studied in mammals but may act as precursors for signaling molecules in plants or microbes .
(9Z,12Z)-Octadeca-9,12-dienoic Acid (Linoleic Acid, Δ⁹,¹²)
- Structure: A positional isomer of this compound, with double bonds at Δ⁹ and Δ¹².
- Molecular Formula : C₁₈H₃₂O₂ ; Molecular Weight : 280.45 g/mol .
- Key Differences: Double bond positions influence physical properties; linoleic acid (Δ⁹,¹²) is liquid at room temperature, whereas Δ⁵,⁸ isomers may exhibit different melting points. Biological Role: Linoleic acid is an essential omega-6 fatty acid critical for maintaining skin barrier function and serving as a precursor to pro-inflammatory eicosanoids. In contrast, this compound’s role is less defined but may modulate sebum composition .
Oleic Acid (Octadeca-9-enoic Acid, Δ⁹)
- Structure: An 18-carbon monoenoic acid with one double bond at Δ⁹.
- Molecular Formula : C₁₈H₃₄O₂ ; Molecular Weight : 282.47 g/mol .
- Key Differences: Lower unsaturation (one double bond) increases molecular weight compared to dienoic acids. Functional Role: Oleic acid is a major component of human sebum and dietary fats.
Tetradeca-5,8-dienoic Acid (Δ⁵,⁸)
- Structure: A 14-carbon dienoic acid with double bonds at Δ⁵ and Δ⁸.
- Molecular Formula : C₁₄H₂₄O₂ ; Molecular Weight : 224.34 g/mol .
- Key Differences: Shorter carbon chain reduces hydrophobicity and alters metabolic pathways. Applications: Found in plant lipids and microbial systems, this compound is less relevant to human physiology compared to this compound but serves as a model for studying unsaturated fatty acid biosynthesis .
Comparative Data Table
Research Findings and Implications
- Skin Health: this compound’s reduced levels in severe acne patients suggest it may inhibit pro-inflammatory pathways or regulate sebum viscosity, unlike linoleic acid, which is directly involved in barrier repair .
- Structural Reactivity: The position and number of double bonds influence enzymatic conversion. For example, linoleic acid (Δ⁹,¹²) is a substrate for lipoxygenases, while this compound’s Δ⁵,⁸ configuration may favor alternative oxidation pathways .
- Industrial Applications: Oleic acid’s stability and moisturizing properties make it preferable in cosmetics, whereas this compound’s niche role limits its commercial use .
Biologische Aktivität
Overview
Octadeca-5,8-dienoic acid, also known as (10E,12Z)-octadecadienoic acid, is a polyunsaturated fatty acid characterized by its molecular formula . It features two double bonds located at the 5th and 8th carbon positions. This compound is derived from linoleic acid and is found in various plant oils, including grape seed oil and sunflower oil. Its unique structure contributes to distinct biological activities and metabolic pathways compared to other fatty acids.
Target Enzymes and Pathways
This compound interacts with specific enzymes, notably the putative aminooxidase in Propionibacterium acnes. This interaction suggests that it may influence metabolic processes related to amino acids and biogenic amines, potentially affecting cellular metabolism and signaling pathways.
As a fatty acid, this compound can be converted into acyl phosphate, a precursor to acyl-CoA. This conversion is essential for various lipid metabolic pathways and cellular functions. The compound can undergo various chemical reactions, including oxidation and reduction, which can lead to the formation of hydroperoxides and other oxidation products.
Anti-inflammatory Effects
Research indicates that this compound possesses anti-inflammatory properties. It has been studied for its potential role in reducing inflammation, particularly in skin-related conditions such as acne. Its interaction with Propionibacterium acnes may modulate inflammatory responses in the skin.
Cardiovascular Health
This compound may contribute to cardiovascular health through its role in lipid metabolism. Fatty acids are known to influence cholesterol levels and overall heart health. Preliminary studies suggest that this compound could have beneficial effects on lipid profiles .
Case Study: Acne Treatment
A study investigated the effects of this compound on Propionibacterium acnes in vitro. The results showed that the compound could inhibit the growth of this bacterium while modulating inflammatory markers associated with acne lesions. This suggests a potential therapeutic application in acne treatment.
Comparative Analysis with Similar Compounds
To better understand the unique properties of this compound, it is useful to compare it with similar fatty acids:
Compound Name | Molecular Formula | Unique Features |
---|---|---|
Linoleic Acid | C18H32O2 | Double bonds at positions 9 and 12 |
Alpha-Linolenic Acid | C18H30O2 | Contains three double bonds at positions 9, 12, 15 |
Oleic Acid | C18H34O2 | Single double bond at position 9 |
Arachidonic Acid | C20H32O2 | Four double bonds at various positions |
The unique positioning of double bonds in this compound distinguishes it from other fatty acids and may lead to different biological activities and applications.
Q & A
Basic Research Questions
Q. How is Octadeca-5,8-dienoic acid structurally characterized, and what analytical techniques are critical for its identification?
- Methodological Answer : Structural elucidation requires nuclear magnetic resonance (NMR) spectroscopy (¹H and ¹³C) to confirm double-bond positions at C5 and C8, supported by gas chromatography-mass spectrometry (GC-MS) for purity assessment. Infrared (IR) spectroscopy can validate carboxylate functional groups. Reference standards with confirmed CAS 2197-50-4 are essential for comparative analysis .
Q. What synthetic routes are used to produce this compound in laboratory settings?
- Methodological Answer : Biosynthetic pathways involve elongation and desaturation of shorter-chain precursors (e.g., deuterium-labeled intermediates) using enzyme systems like fatty acid desaturases. Chemical synthesis may employ Wittig reactions or catalytic hydrogenation to introduce double bonds stereospecifically. Purity is monitored via thin-layer chromatography (TLC) and high-performance liquid chromatography (HPLC) .
Q. Which experimental models are suitable for studying the biological activity of this compound?
- Methodological Answer : In vitro assays using human cell lines (e.g., HepG2 for lipid metabolism) or microbial systems (e.g., Streptomyces spp. for biosynthetic studies) are common. Dose-response curves and kinetic studies should control for solvent interference (e.g., DMSO cytotoxicity). Data normalization to internal standards (e.g., stearic acid) ensures reproducibility .
Q. How can researchers address inconsistencies in reported melting points or solubility data for this compound?
- Methodological Answer : Discrepancies often arise from polymorphic forms or impurities. Recrystallization in polar solvents (e.g., ethanol/water mixtures) followed by differential scanning calorimetry (DSC) can resolve polymorph issues. Solubility studies must standardize temperature, pH, and solvent systems, referencing IUPAC guidelines .
Q. What strategies ensure accurate quantification of this compound in complex biological matrices?
- Methodological Answer : Liquid-liquid extraction (LLE) with chloroform/methanol mixtures isolates lipids, followed by derivatization (e.g., methyl ester formation) for GC-MS analysis. Internal standards (e.g., heptadecanoic acid) correct for recovery losses. Matrix-matched calibration curves mitigate ion suppression effects .
Advanced Research Questions
Q. How do double-bond configurations (cis/trans) at C5 and C8 influence the compound’s metabolic stability in vivo?
- Methodological Answer : Isotopic labeling (e.g., ¹³C at C5/C8) paired with tracer studies in animal models tracks β-oxidation rates. Comparative NMR analysis of cis vs. trans isomers identifies steric hindrance effects on enzyme binding. Data should be contextualized with molecular docking simulations of acyl-CoA dehydrogenases .
Q. What molecular mechanisms underlie the compound’s reported inhibition of lipase activity?
- Methodological Answer : Kinetic assays (e.g., Michaelis-Menten plots) using purified pancreatic lipase quantify competitive vs. non-competitive inhibition. X-ray crystallography of enzyme-inhibitor complexes reveals binding motifs. Mutagenesis studies (e.g., Ser152Ala substitutions) validate catalytic site interactions .
Q. How can researchers resolve contradictions in its purported anti-inflammatory vs. pro-inflammatory effects across studies?
- Methodological Answer : Systematic review of cell-specific responses (e.g., macrophage vs. endothelial cells) using cytokine profiling (ELISA/MSD multiplex assays). Dose- and time-dependent experiments clarify biphasic effects. Meta-analyses should account for interspecies variability (e.g., murine vs. human TNF-α signaling) .
Q. What advanced spectral techniques differentiate this compound from its Δ6,9 or Δ4,7 isomers?
- Methodological Answer : Two-dimensional NMR (COSY, HSQC) maps proton-proton coupling networks, while ozone-induced dissociation mass spectrometry (OzID-MS) cleaves double bonds for positional confirmation. Comparative retention indices on reverse-phase HPLC with C30 columns enhance separation .
Q. How do environmental factors (e.g., pH, temperature) affect its oxidative degradation in storage?
- Methodological Answer : Accelerated stability studies under ICH guidelines (40°C/75% RH) monitor peroxide formation via iodometric titration. Antioxidants (e.g., BHT) are tested for efficacy using Arrhenius modeling. Degradation products are characterized by LC-QTOF-MS and compared to stress-test databases .
Q. Data Presentation and Analysis
Eigenschaften
IUPAC Name |
octadeca-5,8-dienoic acid | |
---|---|---|
Source | PubChem | |
URL | https://pubchem.ncbi.nlm.nih.gov | |
Description | Data deposited in or computed by PubChem | |
InChI |
InChI=1S/C18H32O2/c1-2-3-4-5-6-7-8-9-10-11-12-13-14-15-16-17-18(19)20/h10-11,13-14H,2-9,12,15-17H2,1H3,(H,19,20) | |
Source | PubChem | |
URL | https://pubchem.ncbi.nlm.nih.gov | |
Description | Data deposited in or computed by PubChem | |
InChI Key |
UQQPKQIHIFHHKO-UHFFFAOYSA-N | |
Source | PubChem | |
URL | https://pubchem.ncbi.nlm.nih.gov | |
Description | Data deposited in or computed by PubChem | |
Canonical SMILES |
CCCCCCCCCC=CCC=CCCCC(=O)O | |
Source | PubChem | |
URL | https://pubchem.ncbi.nlm.nih.gov | |
Description | Data deposited in or computed by PubChem | |
Molecular Formula |
C18H32O2 | |
Source | PubChem | |
URL | https://pubchem.ncbi.nlm.nih.gov | |
Description | Data deposited in or computed by PubChem | |
Molecular Weight |
280.4 g/mol | |
Source | PubChem | |
URL | https://pubchem.ncbi.nlm.nih.gov | |
Description | Data deposited in or computed by PubChem | |
Retrosynthesis Analysis
AI-Powered Synthesis Planning: Our tool employs the Template_relevance Pistachio, Template_relevance Bkms_metabolic, Template_relevance Pistachio_ringbreaker, Template_relevance Reaxys, Template_relevance Reaxys_biocatalysis model, leveraging a vast database of chemical reactions to predict feasible synthetic routes.
One-Step Synthesis Focus: Specifically designed for one-step synthesis, it provides concise and direct routes for your target compounds, streamlining the synthesis process.
Accurate Predictions: Utilizing the extensive PISTACHIO, BKMS_METABOLIC, PISTACHIO_RINGBREAKER, REAXYS, REAXYS_BIOCATALYSIS database, our tool offers high-accuracy predictions, reflecting the latest in chemical research and data.
Strategy Settings
Precursor scoring | Relevance Heuristic |
---|---|
Min. plausibility | 0.01 |
Model | Template_relevance |
Template Set | Pistachio/Bkms_metabolic/Pistachio_ringbreaker/Reaxys/Reaxys_biocatalysis |
Top-N result to add to graph | 6 |
Feasible Synthetic Routes
Haftungsausschluss und Informationen zu In-Vitro-Forschungsprodukten
Bitte beachten Sie, dass alle Artikel und Produktinformationen, die auf BenchChem präsentiert werden, ausschließlich zu Informationszwecken bestimmt sind. Die auf BenchChem zum Kauf angebotenen Produkte sind speziell für In-vitro-Studien konzipiert, die außerhalb lebender Organismen durchgeführt werden. In-vitro-Studien, abgeleitet von dem lateinischen Begriff "in Glas", beinhalten Experimente, die in kontrollierten Laborumgebungen unter Verwendung von Zellen oder Geweben durchgeführt werden. Es ist wichtig zu beachten, dass diese Produkte nicht als Arzneimittel oder Medikamente eingestuft sind und keine Zulassung der FDA für die Vorbeugung, Behandlung oder Heilung von medizinischen Zuständen, Beschwerden oder Krankheiten erhalten haben. Wir müssen betonen, dass jede Form der körperlichen Einführung dieser Produkte in Menschen oder Tiere gesetzlich strikt untersagt ist. Es ist unerlässlich, sich an diese Richtlinien zu halten, um die Einhaltung rechtlicher und ethischer Standards in Forschung und Experiment zu gewährleisten.