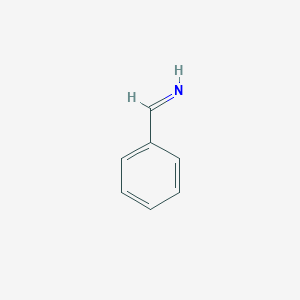
Phenylmethanimine
Übersicht
Beschreibung
Phenylmethanimine is an aromatic imine with a molecular formula of C7H7N . It has an average mass of 105.137 Da and a monoisotopic mass of 105.057846 Da . It’s relevant in two main areas of chemistry: organic synthesis and astro-chemistry .
Synthesis Analysis
A new, easily accessible synthetic approach has been introduced to generate stable imine-intermediates of Phenylmethanimine in the gas phase and in solution . This formation pathway is based on the thermal decomposition of hydrobenzamide .Molecular Structure Analysis
The molecular structure of Phenylmethanimine is represented by the formula C7H7N . More detailed structural analysis would require specific spectroscopic techniques.Chemical Reactions Analysis
Phenylmethanimine is involved in [3 + 2] cycloaddition reactions with N-methyl-1-phenylmethanimine oxide nitrone 1 and bicyclopropylidene 2 . This reaction is analyzed using molecular electron density theory (MEDT) at the B3LYP/6–311 + + G (d,p) level .Wissenschaftliche Forschungsanwendungen
Synthesis and Spectroscopy : Phenylmethanimine is significant in organic synthesis and astrochemistry. A study by Melli et al. (2020) introduced a new synthetic approach for generating stable imine intermediates both in the gas phase and in solution. This method is useful for the laboratory observation of phenylmethanimine and aids in guiding future astronomical observations.
Corrosion Inhibition : Phenylmethanimine derivatives, specifically Schiff bases, were designed for their corrosion inhibition efficiencies on steel. Fernandes et al. (2020) demonstrated their application as effective corrosion inhibitors for steel in acidic media, highlighting their potential in material science and engineering.
Living Radical Polymerization : A study by Wang et al. (2001) explored the use of pyridyl methanimine-based ligands in the living radical polymerization of hydrophilic monomers. This process is significant for polymer chemistry, particularly in creating polymers with controlled molecular weights and low polydispersities.
Molecular Structure Analysis : The molecular structure of phenylmethanimine-related compounds has been analyzed in various studies. For instance, Puranik et al. (1992) determined the structure of a tetranucleating ligand related to phenylmethanimine, which is crucial for understanding its chemical behavior and interactions.
Drug Development and COVID-19 Research : A novel series of methanimines, including phenylmethanimine derivatives, were synthesized and evaluated for their potential as COVID-19 therapeutic agents. Abu-Melha et al. (2022) conducted molecular docking studies, suggesting these compounds as promising candidates for further investigation in drug development for COVID-19 treatment.
Electrophilic Aromatic Substitution in Mass Spectrometry : Shen et al. (2014) investigated the gas-phase fragmentations of protonated N-benzylbenzaldimines, including phenylmethanimine derivatives, using mass spectrometry. This study is significant in the field of analytical chemistry, particularly in understanding the fragmentation mechanisms of complex molecules.
Zukünftige Richtungen
Phenylmethanimine has a twofold relevance in chemistry: organic synthesis and astro-chemistry . Its synthesis and characterization have been explored, providing a foundation for future astronomical observations . Future directions will include both a complementary report covering synthetic strategies to access 2-phenethylamine derivatives and a second, satellite review of 2-heteroaryl-ethylamines in medicinal chemistry .
Eigenschaften
IUPAC Name |
phenylmethanimine | |
---|---|---|
Source | PubChem | |
URL | https://pubchem.ncbi.nlm.nih.gov | |
Description | Data deposited in or computed by PubChem | |
InChI |
InChI=1S/C7H7N/c8-6-7-4-2-1-3-5-7/h1-6,8H | |
Source | PubChem | |
URL | https://pubchem.ncbi.nlm.nih.gov | |
Description | Data deposited in or computed by PubChem | |
InChI Key |
AFDMODCXODAXLC-UHFFFAOYSA-N | |
Source | PubChem | |
URL | https://pubchem.ncbi.nlm.nih.gov | |
Description | Data deposited in or computed by PubChem | |
Canonical SMILES |
C1=CC=C(C=C1)C=N | |
Source | PubChem | |
URL | https://pubchem.ncbi.nlm.nih.gov | |
Description | Data deposited in or computed by PubChem | |
Molecular Formula |
C7H7N | |
Source | PubChem | |
URL | https://pubchem.ncbi.nlm.nih.gov | |
Description | Data deposited in or computed by PubChem | |
DSSTOX Substance ID |
DTXSID30330241 | |
Record name | Phenylmethanimine | |
Source | EPA DSSTox | |
URL | https://comptox.epa.gov/dashboard/DTXSID30330241 | |
Description | DSSTox provides a high quality public chemistry resource for supporting improved predictive toxicology. | |
Molecular Weight |
105.14 g/mol | |
Source | PubChem | |
URL | https://pubchem.ncbi.nlm.nih.gov | |
Description | Data deposited in or computed by PubChem | |
Product Name |
Phenylmethanimine | |
CAS RN |
16118-22-2 | |
Record name | Phenylmethanimine | |
Source | EPA DSSTox | |
URL | https://comptox.epa.gov/dashboard/DTXSID30330241 | |
Description | DSSTox provides a high quality public chemistry resource for supporting improved predictive toxicology. | |
Retrosynthesis Analysis
AI-Powered Synthesis Planning: Our tool employs the Template_relevance Pistachio, Template_relevance Bkms_metabolic, Template_relevance Pistachio_ringbreaker, Template_relevance Reaxys, Template_relevance Reaxys_biocatalysis model, leveraging a vast database of chemical reactions to predict feasible synthetic routes.
One-Step Synthesis Focus: Specifically designed for one-step synthesis, it provides concise and direct routes for your target compounds, streamlining the synthesis process.
Accurate Predictions: Utilizing the extensive PISTACHIO, BKMS_METABOLIC, PISTACHIO_RINGBREAKER, REAXYS, REAXYS_BIOCATALYSIS database, our tool offers high-accuracy predictions, reflecting the latest in chemical research and data.
Strategy Settings
Precursor scoring | Relevance Heuristic |
---|---|
Min. plausibility | 0.01 |
Model | Template_relevance |
Template Set | Pistachio/Bkms_metabolic/Pistachio_ringbreaker/Reaxys/Reaxys_biocatalysis |
Top-N result to add to graph | 6 |
Feasible Synthetic Routes
Haftungsausschluss und Informationen zu In-Vitro-Forschungsprodukten
Bitte beachten Sie, dass alle Artikel und Produktinformationen, die auf BenchChem präsentiert werden, ausschließlich zu Informationszwecken bestimmt sind. Die auf BenchChem zum Kauf angebotenen Produkte sind speziell für In-vitro-Studien konzipiert, die außerhalb lebender Organismen durchgeführt werden. In-vitro-Studien, abgeleitet von dem lateinischen Begriff "in Glas", beinhalten Experimente, die in kontrollierten Laborumgebungen unter Verwendung von Zellen oder Geweben durchgeführt werden. Es ist wichtig zu beachten, dass diese Produkte nicht als Arzneimittel oder Medikamente eingestuft sind und keine Zulassung der FDA für die Vorbeugung, Behandlung oder Heilung von medizinischen Zuständen, Beschwerden oder Krankheiten erhalten haben. Wir müssen betonen, dass jede Form der körperlichen Einführung dieser Produkte in Menschen oder Tiere gesetzlich strikt untersagt ist. Es ist unerlässlich, sich an diese Richtlinien zu halten, um die Einhaltung rechtlicher und ethischer Standards in Forschung und Experiment zu gewährleisten.