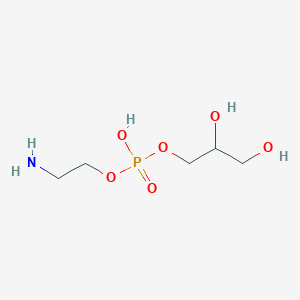
Glycerophosphoethanolamin
Übersicht
Beschreibung
Glycerophosphorylethanolamine (GPEA) is a phospholipid that is a derivative of ethanolamine and glycerol. It is found in the brain and other tissues and plays an important role in various biological processes. In
Wissenschaftliche Forschungsanwendungen
Stimulation des Lebergewebwachstums
Glycerophosphoethanolamin (GPEA) wurde in Leberauszügen als ein Stimulator des Lebergewebwachstums identifiziert . Es wirkt synergistisch mit Wachstumsfaktoren wie dem Hepatozyten-Wachstumsfaktor und dem Transforming Growth Factor-alpha, um das Lebergewebwachstum in serumfreien Kulturen zu stimulieren . Der Ethanolaminanteil des Moleküls ist für die beobachtete Aktivität entscheidend .
Alzheimer-Forschung
This compound fördert die Aggregation von Amyloid-β (1-40) (Aβ40) in vitro . Dies ist bedeutsam, da die Aβ40-Aggregation ein Schlüsselprozess bei der Entwicklung der Alzheimer-Krankheit ist. Darüber hinaus sind die Glycerophosphoethanolaminspiegel in postmortalen Gehirnen, die von Patienten mit Alzheimer-Krankheit isoliert wurden, erhöht .
Lipidbiochemie
Als aktiver Phosphodiester-Metabolit von Phosphatidylethanolamin spielt this compound eine Rolle in der Lipidbiochemie .
Neurodegenerative Erkrankungen
Angesichts seiner Rolle bei der Förderung der Aβ40-Aggregation ist this compound auch für die Erforschung anderer neurodegenerativer Erkrankungen relevant .
Linolsäureoxidation
Es gibt Hinweise darauf, dass this compound eine Rolle bei der Oxidation von Linolsäure spielt . Es werden jedoch weitere Untersuchungen benötigt, um diese Anwendung vollständig zu verstehen.
Pharmazeutische Entwicklung
Aufgrund seiner verschiedenen Rollen in biologischen Prozessen könnte this compound möglicherweise bei der Entwicklung neuer Arzneimittel eingesetzt werden .
Safety and Hazards
The safety data sheet for L-alpha-Glycerophosphorylethanolamine indicates that it is not classified under physical hazards, health hazards, environmental hazards, or OSHA defined hazards . In case of inhalation, skin contact, eye contact, or ingestion, appropriate first aid measures should be taken .
Wirkmechanismus
Target of Action
Glycerophosphorylethanolamine is an active phosphodiester metabolite of phosphatidylethanolamine .
Mode of Action
The ethanolamine portion of the molecule is critical for its observed activity .
Biochemical Pathways
Glycerophosphorylethanolamine is involved in several biochemical pathways. It is a breakdown product of the phospholipid phosphatidylethanolamine . It’s also suggested that the synthesized DHA can potentially get phosphorylated and subsequently fed into certain Embden–Meyerhof pathways .
Result of Action
Glycerophosphorylethanolamine has been found to promote the aggregation of amyloid-β (1-40) (Aβ40) in vitro . Moreover, levels of Glycerophosphorylethanolamine are elevated in postmortem brains isolated from patients with Alzheimer’s disease .
Biochemische Analyse
Biochemical Properties
Glycerophosphorylethanolamine interacts with various enzymes, proteins, and other biomolecules. It is an active metabolite of phosphatidylethanolamine, promoting the aggregation of amyloid-beta (1-40) in vitro . The nature of these interactions is complex and involves various biochemical reactions.
Cellular Effects
Glycerophosphorylethanolamine has significant effects on various types of cells and cellular processes. It influences cell function, including impacts on cell signaling pathways, gene expression, and cellular metabolism . For instance, it has been identified as a hepatocyte growth stimulator in liver extracts .
Molecular Mechanism
At the molecular level, Glycerophosphorylethanolamine exerts its effects through binding interactions with biomolecules, enzyme inhibition or activation, and changes in gene expression . The ethanolamine portion of the molecule is critical for the observed activity .
Dosage Effects in Animal Models
The effects of Glycerophosphorylethanolamine vary with different dosages in animal models
Metabolic Pathways
Glycerophosphorylethanolamine is involved in several metabolic pathways. It interacts with enzymes or cofactors and can affect metabolic flux or metabolite levels
Transport and Distribution
The transport and distribution of Glycerophosphorylethanolamine within cells and tissues involve various transporters or binding proteins
Eigenschaften
IUPAC Name |
2-aminoethyl 2,3-dihydroxypropyl hydrogen phosphate | |
---|---|---|
Source | PubChem | |
URL | https://pubchem.ncbi.nlm.nih.gov | |
Description | Data deposited in or computed by PubChem | |
InChI |
InChI=1S/C5H14NO6P/c6-1-2-11-13(9,10)12-4-5(8)3-7/h5,7-8H,1-4,6H2,(H,9,10) | |
Source | PubChem | |
URL | https://pubchem.ncbi.nlm.nih.gov | |
Description | Data deposited in or computed by PubChem | |
InChI Key |
JZNWSCPGTDBMEW-UHFFFAOYSA-N | |
Source | PubChem | |
URL | https://pubchem.ncbi.nlm.nih.gov | |
Description | Data deposited in or computed by PubChem | |
Canonical SMILES |
C(COP(=O)(O)OCC(CO)O)N | |
Source | PubChem | |
URL | https://pubchem.ncbi.nlm.nih.gov | |
Description | Data deposited in or computed by PubChem | |
Molecular Formula |
C5H14NO6P | |
Source | PubChem | |
URL | https://pubchem.ncbi.nlm.nih.gov | |
Description | Data deposited in or computed by PubChem | |
DSSTOX Substance ID |
DTXSID20862586 | |
Record name | 2-Aminoethyl 2,3-dihydroxypropyl hydrogen phosphate | |
Source | EPA DSSTox | |
URL | https://comptox.epa.gov/dashboard/DTXSID20862586 | |
Description | DSSTox provides a high quality public chemistry resource for supporting improved predictive toxicology. | |
Molecular Weight |
215.14 g/mol | |
Source | PubChem | |
URL | https://pubchem.ncbi.nlm.nih.gov | |
Description | Data deposited in or computed by PubChem | |
Physical Description |
Solid | |
Record name | Glycerylphosphorylethanolamine | |
Source | Human Metabolome Database (HMDB) | |
URL | http://www.hmdb.ca/metabolites/HMDB0000114 | |
Description | The Human Metabolome Database (HMDB) is a freely available electronic database containing detailed information about small molecule metabolites found in the human body. | |
Explanation | HMDB is offered to the public as a freely available resource. Use and re-distribution of the data, in whole or in part, for commercial purposes requires explicit permission of the authors and explicit acknowledgment of the source material (HMDB) and the original publication (see the HMDB citing page). We ask that users who download significant portions of the database cite the HMDB paper in any resulting publications. | |
CAS No. |
1190-00-7 | |
Record name | Glycerophosphoethanolamine | |
Source | CAS Common Chemistry | |
URL | https://commonchemistry.cas.org/detail?cas_rn=1190-00-7 | |
Description | CAS Common Chemistry is an open community resource for accessing chemical information. Nearly 500,000 chemical substances from CAS REGISTRY cover areas of community interest, including common and frequently regulated chemicals, and those relevant to high school and undergraduate chemistry classes. This chemical information, curated by our expert scientists, is provided in alignment with our mission as a division of the American Chemical Society. | |
Explanation | The data from CAS Common Chemistry is provided under a CC-BY-NC 4.0 license, unless otherwise stated. | |
Record name | Glycerophosphoethanolamine | |
Source | ChemIDplus | |
URL | https://pubchem.ncbi.nlm.nih.gov/substance/?source=chemidplus&sourceid=0001190007 | |
Description | ChemIDplus is a free, web search system that provides access to the structure and nomenclature authority files used for the identification of chemical substances cited in National Library of Medicine (NLM) databases, including the TOXNET system. | |
Record name | 2-Aminoethyl 2,3-dihydroxypropyl hydrogen phosphate | |
Source | EPA DSSTox | |
URL | https://comptox.epa.gov/dashboard/DTXSID20862586 | |
Description | DSSTox provides a high quality public chemistry resource for supporting improved predictive toxicology. | |
Record name | .ALPHA.-GLYCEROPHOSPHORYLETHANOLAMINE, (±)- | |
Source | FDA Global Substance Registration System (GSRS) | |
URL | https://gsrs.ncats.nih.gov/ginas/app/beta/substances/240I539PWQ | |
Description | The FDA Global Substance Registration System (GSRS) enables the efficient and accurate exchange of information on what substances are in regulated products. Instead of relying on names, which vary across regulatory domains, countries, and regions, the GSRS knowledge base makes it possible for substances to be defined by standardized, scientific descriptions. | |
Explanation | Unless otherwise noted, the contents of the FDA website (www.fda.gov), both text and graphics, are not copyrighted. They are in the public domain and may be republished, reprinted and otherwise used freely by anyone without the need to obtain permission from FDA. Credit to the U.S. Food and Drug Administration as the source is appreciated but not required. | |
Record name | Glycerylphosphorylethanolamine | |
Source | Human Metabolome Database (HMDB) | |
URL | http://www.hmdb.ca/metabolites/HMDB0000114 | |
Description | The Human Metabolome Database (HMDB) is a freely available electronic database containing detailed information about small molecule metabolites found in the human body. | |
Explanation | HMDB is offered to the public as a freely available resource. Use and re-distribution of the data, in whole or in part, for commercial purposes requires explicit permission of the authors and explicit acknowledgment of the source material (HMDB) and the original publication (see the HMDB citing page). We ask that users who download significant portions of the database cite the HMDB paper in any resulting publications. | |
Retrosynthesis Analysis
AI-Powered Synthesis Planning: Our tool employs the Template_relevance Pistachio, Template_relevance Bkms_metabolic, Template_relevance Pistachio_ringbreaker, Template_relevance Reaxys, Template_relevance Reaxys_biocatalysis model, leveraging a vast database of chemical reactions to predict feasible synthetic routes.
One-Step Synthesis Focus: Specifically designed for one-step synthesis, it provides concise and direct routes for your target compounds, streamlining the synthesis process.
Accurate Predictions: Utilizing the extensive PISTACHIO, BKMS_METABOLIC, PISTACHIO_RINGBREAKER, REAXYS, REAXYS_BIOCATALYSIS database, our tool offers high-accuracy predictions, reflecting the latest in chemical research and data.
Strategy Settings
Precursor scoring | Relevance Heuristic |
---|---|
Min. plausibility | 0.01 |
Model | Template_relevance |
Template Set | Pistachio/Bkms_metabolic/Pistachio_ringbreaker/Reaxys/Reaxys_biocatalysis |
Top-N result to add to graph | 6 |
Feasible Synthetic Routes
Q1: What is the molecular formula and weight of Glycerophosphorylethanolamine?
A1: While the provided research papers don't explicitly state the molecular formula and weight of GPE, these can be easily derived from its structure. The molecular formula is C5H14NO6P, and the molecular weight is 215.16 g/mol.
Q2: What spectroscopic techniques are commonly used to characterize GPE?
A2: Phosphorus-31 nuclear magnetic resonance (31P NMR) spectroscopy is widely used to identify and quantify GPE in various biological samples. [, , , , , , , , , ]
Q3: What is the primary metabolic origin of GPE?
A3: GPE is a breakdown product of phosphatidylethanolamine, a major phospholipid found in cell membranes. [, , , , ]
Q4: How do levels of GPE and its precursor, phosphorylethanolamine (PE), change during cellular proliferation?
A4: Research suggests that during periods of increased cellular proliferation, such as the luteal phase of the menstrual cycle, levels of both PE and GPE decrease. This suggests their potential involvement in membrane synthesis and degradation processes associated with cell division. []
Q5: What changes in GPE and related metabolites are observed in the brains of Alzheimer's disease patients?
A5: Studies using 31P NMR spectroscopy on brain samples from Alzheimer's patients have revealed decreased levels of phosphomonoesters, including PE, and increased levels of phosphodiesters, including GPE, in the frontal and parietal regions. This altered phospholipid metabolism may contribute to neuronal membrane dysfunction and impaired cholinergic neurotransmission in AD. [, ]
Q6: Are there any observed links between GPE levels and the severity of liver cirrhosis?
A6: Research using in vivo and in vitro 31P MRS on cirrhotic liver tissues showed elevated levels of PE and reduced levels of GPE, with more pronounced changes observed in patients with decompensated cirrhosis compared to those with compensated cirrhosis. This suggests that altered GPE metabolism may be associated with the severity of liver dysfunction. [, ]
Q7: What role might GPE play in the response of cells to stress?
A7: Studies in fish have shown that GPE and other water-soluble phosphodiesters might contribute to stress resistance. While myocardial ischemia didn't significantly affect GPE levels, osmotic stress induced by changes in water salinity caused fluctuations in GPE and related metabolites, suggesting a potential role in osmoregulation and cellular adaptation to stress. []
Q8: Can GPE serve as a substrate for enzymes?
A8: Yes, research has shown that GPE can act as a substrate for certain enzymes. For example, in Myxococcus xanthus, the short-chain alcohol dehydrogenase SocA utilizes lysophosphatidylethanolamine (lyso-PE) as a substrate. While not directly GPE, this finding highlights the potential for GPE and related compounds to participate in enzymatic reactions with potential signaling roles in bacterial development. []
Q9: What are the challenges in detecting and quantifying GPE in biological samples?
A9: The low concentration of GPE in some tissues and the spectral overlap with other phosphorus-containing metabolites can pose challenges for its detection and quantification. High-resolution NMR spectroscopy and advanced spectral analysis techniques are often required. [, , , , , ]
Q10: How does the choice of NMR field strength affect the visibility of GPE in biological samples?
A10: Studies have shown that phospholipids, including GPE-containing compounds, are more readily visible in 31P NMR spectra acquired at lower field strengths (e.g., 1.5 T) compared to higher field strengths (e.g., 9.4 T) due to a decrease in linewidth at lower fields. This highlights the importance of optimizing field strength for specific applications and target metabolites. []
Haftungsausschluss und Informationen zu In-Vitro-Forschungsprodukten
Bitte beachten Sie, dass alle Artikel und Produktinformationen, die auf BenchChem präsentiert werden, ausschließlich zu Informationszwecken bestimmt sind. Die auf BenchChem zum Kauf angebotenen Produkte sind speziell für In-vitro-Studien konzipiert, die außerhalb lebender Organismen durchgeführt werden. In-vitro-Studien, abgeleitet von dem lateinischen Begriff "in Glas", beinhalten Experimente, die in kontrollierten Laborumgebungen unter Verwendung von Zellen oder Geweben durchgeführt werden. Es ist wichtig zu beachten, dass diese Produkte nicht als Arzneimittel oder Medikamente eingestuft sind und keine Zulassung der FDA für die Vorbeugung, Behandlung oder Heilung von medizinischen Zuständen, Beschwerden oder Krankheiten erhalten haben. Wir müssen betonen, dass jede Form der körperlichen Einführung dieser Produkte in Menschen oder Tiere gesetzlich strikt untersagt ist. Es ist unerlässlich, sich an diese Richtlinien zu halten, um die Einhaltung rechtlicher und ethischer Standards in Forschung und Experiment zu gewährleisten.