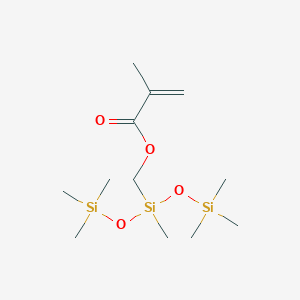
(Methacryloxymethyl)bis(trimethylsiloxy)methylsilane
Übersicht
Beschreibung
(Methacryloxymethyl)bis(trimethylsiloxy)methylsilane (CAS 56051-48-0) is a methacrylate-functionalized organosilicon compound with the molecular formula C₁₂H₂₈O₄Si₃ and a molecular weight of 320.60 g/mol . Its IUPAC name, [methyl-bis(trimethylsilyloxy)silyl]methyl 2-methylprop-2-enoate, highlights its methacrylate group linked to a siloxane backbone. This structure enables radical polymerization, making it valuable in silicone hydrogels, elastomers, and crosslinked polymers .
Vorbereitungsmethoden
Synthetic Routes and Reaction Conditions
The synthesis of T-588 involves several key steps:
Formation of Dimethyl (methylene)-lambda4-sulfane: The reaction of dimethyl sulfate with dimethyl sulfide in the presence of sodium methoxide in acetonitrile yields dimethyl (methylene)-lambda4-sulfane.
Condensation with Benzo[b]thiophene-5-carbaldehyde: This intermediate is then condensed with benzo[b]thiophene-5-carbaldehyde in the same solvent to produce 2-(benzo[b]thiophen-5-yl)oxirane.
Reaction with 2-(Dimethylamino)ethanol: The oxirane is reacted with 2-(dimethylamino)ethanol using potassium tert-butoxide in hot dimethyl sulfoxide or hot dimethylformamide to afford racemic T-588.
Optical Resolution: The racemic mixture undergoes optical resolution by treatment with acetic anhydride in refluxing toluene, followed by reaction with D-dibenzoyltartaric acid in ethyl acetate/acetone/water.
Final Steps: The ®-isomer is deacetylated with sodium methoxide in methanol and salified with ethanolic hydrochloric acid to obtain T-588.
Industrial Production Methods
The industrial production of T-588 follows similar synthetic routes but on a larger scale, ensuring high purity and yield through optimized reaction conditions and purification techniques.
Analyse Chemischer Reaktionen
Arten von Reaktionen
T-588 durchläuft verschiedene chemische Reaktionen, darunter:
Oxidation: T-588 kann unter bestimmten Bedingungen oxidiert werden, um entsprechende Oxide zu bilden.
Reduktion: Reduktionsreaktionen können T-588 in seine reduzierten Formen umwandeln.
Substitution: T-588 kann Substitutionsreaktionen eingehen, insbesondere an seinen Ethoxy- und Aminogruppen.
Häufige Reagenzien und Bedingungen
Oxidation: Häufige Oxidationsmittel sind Kaliumpermanganat und Wasserstoffperoxid.
Reduktion: Es werden Reduktionsmittel wie Lithiumaluminiumhydrid und Natriumborhydrid verwendet.
Substitution: Reagenzien wie Alkylhalogenide und Acylchloride werden in Substitutionsreaktionen eingesetzt.
Hauptprodukte
Die Hauptprodukte, die bei diesen Reaktionen gebildet werden, hängen von den spezifischen Reagenzien und Bedingungen ab, die verwendet werden. Beispielsweise kann Oxidation Oxide ergeben, während Substitution verschiedene substituierte Derivate erzeugen kann .
Wissenschaftliche Forschungsanwendungen
T-588 hat eine breite Palette an wissenschaftlichen Forschungsanwendungen:
Neuroprotektion: Es wurde gezeigt, dass T-588 Motoneuronen vor Glutamat-induzierter Neurotoxizität schützt und das Neuritenwachstum fördert.
Kognitive Verbesserung: Es lindert Lern- und Gedächtnisdefizite in Tiermodellen für Demenz und ist damit eine potenzielle Behandlung für die Alzheimer-Krankheit.
Synaptische Plastizität: T-588 erleichtert die langfristige synaptische Plastizität im Gyrus dentatus frei bewegungsfähiger Ratten, was zu verbesserten Lern- und Gedächtnisfunktionen beiträgt.
Motoneuronerkrankung: T-588 verlangsamt die motorische Verschlechterung bei der Wobbler-Maus-Motoneuronerkrankung, was auf sein Potenzial zur Behandlung von Motoneuronerkrankungen hindeutet.
Wirkmechanismus
T-588 übt seine Wirkungen durch mehrere Mechanismen aus:
Neurotrophe Wirkungen: Es verstärkt das Neuritenwachstum und die Cholinacetyltransferase-Aktivität und fördert so das Überleben und die Erhaltung von Neuronen.
Synaptische Plastizität: T-588 erleichtert die Langzeitpotenzierung im Gyrus dentatus und verstärkt so die synaptische Stärke und Plastizität.
Wissenschaftliche Forschungsanwendungen
2.1. Coupling Agents
(Methacryloxymethyl)bis(trimethylsiloxy)methylsilane is primarily used as a coupling agent in composite materials. Its dual functionality allows it to bond organic polymers with inorganic substrates effectively. This property is particularly beneficial in:
- Glass Fiber Reinforced Plastics (GFRP) : Enhances mechanical properties and durability.
- Mineral-Filled Thermosetting Resins : Improves adhesion and overall performance of the composite materials .
2.2. Adhesion Promoters
This silane compound significantly enhances the adhesion of coatings and sealants to various substrates, including metals and ceramics. It is commonly used in:
- Paints and Coatings : Provides improved adhesion and durability, especially in waterborne systems.
- Sealants : Increases resistance to environmental factors, thereby extending the lifespan of the sealants .
3.1. Dental Materials
In dental applications, this compound plays a crucial role in improving the mechanical properties of dental sealants and composites. It provides better bonding between the dental material and tooth structure, leading to enhanced performance in restorative dentistry .
3.2. Intraocular Lens Materials
Recent studies have explored its use in developing hydrophobic acrylic materials for intraocular lenses. These materials benefit from low tackiness and high refractive indices, making them suitable for ophthalmic devices .
4.1. Composite Materials Research
A study demonstrated that incorporating this compound into glass fiber composites resulted in a substantial increase in tensile strength and flexural modulus compared to composites without silane treatment. The research highlighted its effectiveness in improving interfacial bonding within the composite matrix .
4.2. Coating Technologies
In an industrial application, a coating formulated with this silane exhibited superior water repellency and adhesion properties on metal substrates compared to traditional coatings. This was attributed to the silane's ability to create a robust interface between the coating and substrate, enhancing durability under harsh environmental conditions .
Summary of Benefits
Application Area | Benefits |
---|---|
Composite Materials | Improved mechanical properties, durability |
Dental Materials | Enhanced bonding and performance in restorative applications |
Coatings | Increased adhesion, water repellency |
Intraocular Lenses | Low tackiness, high refractive index |
Wirkmechanismus
T-588 exerts its effects through several mechanisms:
Neurotrophic Effects: It enhances neurite outgrowth and choline acetyltransferase activity, promoting the survival and maintenance of neurons.
Synaptic Plasticity: T-588 facilitates long-term potentiation in the dentate gyrus, enhancing synaptic strength and plasticity.
Calcium Regulation: T-588 reduces intracellular calcium concentration by blocking calcium release from intracellular stores, preventing excitotoxicity and promoting neuroprotection.
Vergleich Mit ähnlichen Verbindungen
Comparison with Structurally Similar Siloxane Compounds
Chemical Structure and Functional Groups
The compound is compared to key analogs based on functional groups and backbone complexity:
Compound Name | Molecular Formula | Molecular Weight (g/mol) | Functional Group | Key Structural Feature |
---|---|---|---|---|
(Methacryloxymethyl)bis(trimethylsiloxy)methylsilane | C₁₂H₂₈O₄Si₃ | 320.60 | Methacrylate | Methacrylate + bis(trimethylsiloxy)methyl |
TRIS (Methacryloxypropyltris(trimethylsiloxy)silane) | C₁₆H₃₄O₅Si₃ | 406.77 | Methacrylate | Tris(trimethylsiloxy) + propyl linkage |
3-glycidoxypropylbis(trimethylsiloxy)methylsilane | C₁₃H₃₀O₅Si₃ | 362.62 | Epoxy, siloxane | Epoxy + bis(trimethylsiloxy)methyl |
Bis(trimethylsiloxy)methylsilane | C₇H₂₂O₂Si₃ | 222.51 | Silane (Si-H) | No polymerizable group |
Key Insights :
- Methacrylate vs. Epoxy Groups : The methacrylate group enables radical polymerization, whereas epoxy-functional siloxanes (e.g., 3-glycidoxypropylbis(trimethylsiloxy)methylsilane) undergo ring-opening reactions for surface coupling .
- Siloxane Backbone Complexity : TRIS has a tris(trimethylsiloxy) group, enhancing hydrophobicity and oxygen permeability in contact lenses compared to the bis(trimethylsiloxy) structure of the target compound .
Reactivity and Polymerization Behavior
Radical Polymerization
- This compound copolymerizes with hydrophilic monomers like HEMA (2-hydroxyethyl methacrylate) to form silicone hydrogels. However, its methacrylate group exhibits slower reactivity compared to acrylates like TRISA (3-[tris-(trimethylsiloxy)silyl]propyl acrylate), leading to microphase separation in networks with PEGDA .
- SiGMA (a hydroxypropyl-modified variant) shows improved compatibility with PEGDA due to hydrogen bonding but still requires diluents for homogeneous networks .
Hydrosilylation and Coupling Reactions
- In contrast, 3-glycidoxypropylbis(trimethylsiloxy)methylsilane undergoes rapid epoxy-amine coupling (8 hours at 25°C), useful for surface functionalization .
Silicone Hydrogels
Property | This compound | TRIS | SiGMA |
---|---|---|---|
Oxygen Permeability (Dk) | Moderate (~60–80 barrer) | High (~100–120 barrer) | Moderate (~70–90 barrer) |
Water Content | 30–40% | 20–30% | 35–45% |
Mechanical Strength | High (crosslinked networks) | Brittle | Flexible |
Notes:
- TRIS’s trisiloxane structure provides higher oxygen permeability but lower water content, limiting comfort in contact lenses .
- The target compound balances oxygen transmission and mechanical stability due to its bis-siloxane and methacrylate groups .
Silicone Elastomers and Composites
Biologische Aktivität
(Methacryloxymethyl)bis(trimethylsiloxy)methylsilane is a siloxane compound that has garnered attention for its diverse applications in organic synthesis, particularly in the fields of pharmaceuticals, coatings, and adhesives. This article delves into its biological activity, synthesis, and potential applications based on current research findings.
- Molecular Formula : CHOSi
- Molecular Weight : 222.51 g/mol
- CAS Number : 1873-88-7
- Boiling Point : 141-142 °C
- Density : 0.8136 g/mL
- Flash Point : 27 °C
Biological Activity
The biological activity of this compound is primarily attributed to its silicone backbone, which allows for various functional modifications. This compound exhibits properties that make it suitable for use in biocompatible materials and coatings.
Antimicrobial Properties
Research indicates that siloxane compounds can possess antimicrobial properties when incorporated into coatings or polymers. For instance, studies have shown that siloxane-based coatings can effectively inhibit microbial growth on surfaces, which is crucial in medical settings to prevent infections associated with implants and devices .
Biocompatibility
The biocompatibility of this compound has been explored in various studies. Its application in medical devices and wound dressings shows promise due to its ability to form stable films that protect underlying tissues while allowing for moisture vapor transmission. This property is essential in maintaining a moist environment conducive to healing .
Applications in Medical and Industrial Fields
- Medical Coatings : The compound is utilized in developing coatings for medical devices that require biocompatibility and antimicrobial properties.
- Adhesives and Sealants : Its chemical structure enables it to function effectively as an adhesive or sealant in various industrial applications.
- Cosmetic Formulations : The compound's silicone nature makes it suitable for use in cosmetic products, enhancing texture and stability.
Case Study 1: Antimicrobial Coating Development
A study focused on the development of a liquid polymer coating containing this compound demonstrated significant antimicrobial activity against common pathogens. The coating was applied to surgical instruments, resulting in a notable reduction in microbial colonization compared to untreated surfaces .
Case Study 2: Biocompatibility Assessment
In another study assessing the biocompatibility of siloxane-based materials, this compound was tested for cytotoxic effects on human fibroblast cells. The results indicated low cytotoxicity, suggesting its potential for use in wound dressings and other medical applications .
Comparative Analysis of Siloxane Compounds
Property | This compound | Other Siloxanes |
---|---|---|
Molecular Weight | 222.51 g/mol | Varies by compound |
Antimicrobial Activity | Yes | Varies; some exhibit activity |
Biocompatibility | High | Varies; some are low |
Applications | Medical coatings, adhesives | Various industrial uses |
Q & A
Q. Basic: What are the recommended synthetic routes and purification methods for (Methacryloxymethyl)bis(trimethylsiloxy)methylsilane?
Methodological Answer:
The compound is typically synthesized via hydrosilylation or siloxane functionalization. For example, derivatives can be prepared using the Piers–Rubinsztajn reaction, which involves reacting hydroxyl-containing precursors with hydrosilanes in the presence of a catalyst (e.g., B(C6F5)3) under anhydrous conditions . Purification often employs column chromatography with silica gel and non-polar solvents (e.g., hexane/ethyl acetate mixtures) to isolate the product. For lab-scale synthesis, reaction monitoring via thin-layer chromatography (TLC) is critical to confirm completion .
Q. Basic: What analytical techniques are most effective for characterizing this compound?
Methodological Answer:
Key techniques include:
- Nuclear Magnetic Resonance (NMR): ¹H, ¹³C, and ²⁹Si NMR to confirm siloxane linkages and methacryloyl group integrity .
- Mass Spectrometry (MS): High-resolution MS (e.g., ESI-TOF) to verify molecular weight and fragmentation patterns .
- Thermogravimetric Analysis (TGA): Assess thermal stability and decomposition profiles (e.g., weight loss at 200–300°C under nitrogen) .
- Gel Permeation Chromatography (GPC): Determine molecular weight distribution in polymer composites .
Q. Advanced: How does steric hindrance from trimethylsiloxy groups influence its reactivity in hydrosilylation?
Methodological Answer:
Competitive kinetic studies show that bulky trimethylsiloxy substituents reduce reaction rates in hydrosilylation. For instance, bis(trimethylsiloxy)methylsilane reacts slower with divinyltetramethyldisiloxane compared to less hindered silanes due to steric effects . To mitigate this, catalysts like Karstedt’s platinum complex (Pt₂(dvs)) are used at elevated temperatures (80–100°C) to enhance reactivity . Mechanistic studies suggest that electron-withdrawing siloxy groups also polarize Si–H bonds, altering regioselectivity .
Q. Advanced: What strategies improve its compatibility in silicone elastomer composites?
Methodological Answer:
Derivatization with bis(trimethylsiloxy)methylsilane groups via the Piers–Rubinsztajn reaction enhances compatibility with silicone matrices. For example, siliconized porphyrin additives achieve uniform dispersion at 1–10 wt% in polydimethylsiloxane (PDMS), improving permittivity (ε’ = 4.5–6.2 at 1 kHz) without phase separation . Pre-treatment of fillers with silane coupling agents (e.g., 5% dimethyldichlorosilane in toluene) reduces interfacial tension and prevents aggregation .
Q. Advanced: How can plasma-enhanced CVD parameters be optimized for thin-film deposition?
Methodological Answer:
In plasma-enhanced CVD, parameters include:
- Precursor Ratio: Co-reactants like 1,1,3,3-tetramethyldisiloxane (10–20 vol%) improve film uniformity .
- Plasma Power: 50–100 W to balance deposition rate and film integrity.
- Substrate Temperature: 100–150°C enhances adhesion and reduces pinholes.
Post-deposition annealing (200°C, 1 hr) under nitrogen densifies the film, reducing dielectric loss (tan δ < 0.01 at 1 MHz) .
Q. Basic: What handling and storage protocols prevent degradation?
Methodological Answer:
- Storage: Under inert gas (argon/nitrogen) at –20°C in amber glass vials to prevent hydrolysis of siloxane bonds .
- Handling: Use anhydrous solvents (e.g., THF dried over molecular sieves) and gloveboxes for moisture-sensitive reactions .
- Safety: Avoid prolonged skin contact; use fume hoods due to volatile byproducts (e.g., trimethylsilanol) .
Q. Advanced: What challenges arise in achieving high permittivity in elastomers with this compound?
Methodological Answer:
High permittivity (>6) requires homogeneous dispersion of additives, but aggregation occurs above 10 wt%. Strategies include:
- In-situ Polymerization: Co-polymerize methacryloyl-functionalized siloxanes with PDMS to create covalent networks .
- Nanostructuring: Blend with conductive fillers (e.g., carbon nanotubes, 0.1–0.5 wt%) to form percolation pathways without compromising elasticity .
- Crosslinking Density: Adjust PDMS crosslinker ratios (e.g., 10:1 base:curing agent) to balance mechanical and dielectric properties .
Q. Advanced: How do competitive reaction kinetics inform catalytic system selection?
Methodological Answer:
Relative rate studies using bis(trimethylsiloxy)methylsilane reveal that electron-deficient alkenes (e.g., vinylpentamethyldisiloxane) react faster than electron-rich ones (e.g., divinyltetramethyldisiloxane) with Pt catalysts. Turnover frequencies (TOF) increase by 2–3× when using bidentate ligands (e.g., diphosphines) to stabilize Pt centers . Kinetic modeling (e.g., Michaelis-Menten) helps predict optimal catalyst loading (0.5–1.0 mol%) for batch reactors .
Eigenschaften
IUPAC Name |
[methyl-bis(trimethylsilyloxy)silyl]methyl 2-methylprop-2-enoate | |
---|---|---|
Source | PubChem | |
URL | https://pubchem.ncbi.nlm.nih.gov | |
Description | Data deposited in or computed by PubChem | |
InChI |
InChI=1S/C12H28O4Si3/c1-11(2)12(13)14-10-19(9,15-17(3,4)5)16-18(6,7)8/h1,10H2,2-9H3 | |
Source | PubChem | |
URL | https://pubchem.ncbi.nlm.nih.gov | |
Description | Data deposited in or computed by PubChem | |
InChI Key |
YPMNWQTVWVHXIQ-UHFFFAOYSA-N | |
Source | PubChem | |
URL | https://pubchem.ncbi.nlm.nih.gov | |
Description | Data deposited in or computed by PubChem | |
Canonical SMILES |
CC(=C)C(=O)OC[Si](C)(O[Si](C)(C)C)O[Si](C)(C)C | |
Source | PubChem | |
URL | https://pubchem.ncbi.nlm.nih.gov | |
Description | Data deposited in or computed by PubChem | |
Molecular Formula |
C12H28O4Si3 | |
Source | PubChem | |
URL | https://pubchem.ncbi.nlm.nih.gov | |
Description | Data deposited in or computed by PubChem | |
Molecular Weight |
320.60 g/mol | |
Source | PubChem | |
URL | https://pubchem.ncbi.nlm.nih.gov | |
Description | Data deposited in or computed by PubChem | |
Retrosynthesis Analysis
AI-Powered Synthesis Planning: Our tool employs the Template_relevance Pistachio, Template_relevance Bkms_metabolic, Template_relevance Pistachio_ringbreaker, Template_relevance Reaxys, Template_relevance Reaxys_biocatalysis model, leveraging a vast database of chemical reactions to predict feasible synthetic routes.
One-Step Synthesis Focus: Specifically designed for one-step synthesis, it provides concise and direct routes for your target compounds, streamlining the synthesis process.
Accurate Predictions: Utilizing the extensive PISTACHIO, BKMS_METABOLIC, PISTACHIO_RINGBREAKER, REAXYS, REAXYS_BIOCATALYSIS database, our tool offers high-accuracy predictions, reflecting the latest in chemical research and data.
Strategy Settings
Precursor scoring | Relevance Heuristic |
---|---|
Min. plausibility | 0.01 |
Model | Template_relevance |
Template Set | Pistachio/Bkms_metabolic/Pistachio_ringbreaker/Reaxys/Reaxys_biocatalysis |
Top-N result to add to graph | 6 |
Feasible Synthetic Routes
Haftungsausschluss und Informationen zu In-Vitro-Forschungsprodukten
Bitte beachten Sie, dass alle Artikel und Produktinformationen, die auf BenchChem präsentiert werden, ausschließlich zu Informationszwecken bestimmt sind. Die auf BenchChem zum Kauf angebotenen Produkte sind speziell für In-vitro-Studien konzipiert, die außerhalb lebender Organismen durchgeführt werden. In-vitro-Studien, abgeleitet von dem lateinischen Begriff "in Glas", beinhalten Experimente, die in kontrollierten Laborumgebungen unter Verwendung von Zellen oder Geweben durchgeführt werden. Es ist wichtig zu beachten, dass diese Produkte nicht als Arzneimittel oder Medikamente eingestuft sind und keine Zulassung der FDA für die Vorbeugung, Behandlung oder Heilung von medizinischen Zuständen, Beschwerden oder Krankheiten erhalten haben. Wir müssen betonen, dass jede Form der körperlichen Einführung dieser Produkte in Menschen oder Tiere gesetzlich strikt untersagt ist. Es ist unerlässlich, sich an diese Richtlinien zu halten, um die Einhaltung rechtlicher und ethischer Standards in Forschung und Experiment zu gewährleisten.