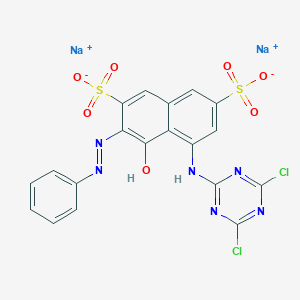
C.I. Reactive red 2
Übersicht
Beschreibung
C.I. Reactive Red 2 (RR2) is a sulfonated azo dye widely used in the textile industry for coloring cellulose-based fabrics. Its chemical formula is C₁₉H₁₀Cl₂N₆Na₂O₇S₂, with a molecular weight of 615.33 g/mol and CAS numbers 17804-49-8 and 12226-03-8 . Structurally, RR2 features two monochlorotriazine reactive groups and an azo (-N=N-) chromophore, enabling covalent bonding with fibers during dyeing . The dye exhibits strong absorption at λmax = 543 nm (visible region) and 288 nm (UV region), contributing to its vivid coloration .
RR2 is recalcitrant to conventional wastewater treatments due to its stable aromatic structure, necessitating advanced oxidation processes (AOPs) for degradation. Studies highlight its susceptibility to ozone-based systems (e.g., O₃/H₂O₂/Fe³⁺), achieving >95% decolorization under optimal conditions .
Wirkmechanismus
Target of Action
C.I. Reactive Red 2 (RR2) is primarily targeted towards organic pollutants in the environment . It is used in various industrial processes, particularly in textile industries, and can often be found in wastewater .
Mode of Action
The mode of action of RR2 involves its interaction with other substances to bring about a change in color or decolorization. This is particularly evident in processes such as ozonation catalyzed by Fe (II) and UV , and photodegradation in the Bi2WO6 system . In these processes, RR2 interacts with the catalysts to undergo a color change, which is a key aspect of its functionality.
Biochemical Pathways
The biochemical pathways affected by RR2 primarily involve the degradation of organic pollutants. In the presence of catalysts like Fe (II), UV, or Bi2WO6, RR2 undergoes a series of reactions that lead to its decolorization . These reactions are part of the larger process of pollutant degradation in the environment.
Result of Action
The primary result of RR2’s action is the decolorization of the dye itself and the degradation of organic pollutants. This is achieved through its interaction with catalysts in processes such as ozonation and photodegradation . The rate of RR2 removal or decolorization can be influenced by factors such as the concentration of RR2, the dose of the catalyst, and the pH of the solution .
Action Environment
The action, efficacy, and stability of RR2 are influenced by various environmental factors. For instance, the pH of the solution can affect the rate of RR2’s decolorization . Similarly, the presence and concentration of catalysts like Fe (II), UV, or Bi2WO6 can significantly impact the effectiveness of RR2’s decolorization and pollutant degradation processes .
Biochemische Analyse
Biochemical Properties
The exact biochemical properties of C.I. Reactive Red 2 are not well-studied. It is known that reactive dyes can interact with various biomolecules. For instance, they can bind to proteins and enzymes, potentially altering their function . The nature of these interactions is largely dependent on the specific structure of the dye and the biomolecule involved .
Cellular Effects
The effects of this compound on cells are not well-documented. It is known that reactive dyes can have various effects on cellular processes. For instance, they can influence cell signaling pathways, gene expression, and cellular metabolism The exact effects of CI
Molecular Mechanism
The molecular mechanism of action of this compound is not well-understood. It is known that reactive dyes can interact with biomolecules at the molecular level. For instance, they can bind to enzymes, potentially inhibiting or activating them . They can also interact with DNA, potentially causing changes in gene expression . Further research would be needed to determine the specific molecular mechanisms of this compound.
Temporal Effects in Laboratory Settings
The temporal effects of CIIt is known that reactive dyes can have long-term effects on cellular function . For instance, they can cause changes in cell signaling pathways and gene expression that persist over time The stability and degradation of CI
Dosage Effects in Animal Models
The effects of different dosages of CIIt is known that reactive dyes can have toxic or adverse effects at high doses . For instance, they can cause damage to various organs and tissues The specific dosage effects of CI
Metabolic Pathways
The metabolic pathways that CIIt is known that reactive dyes can interact with various enzymes and cofactors . They can also have effects on metabolic flux and metabolite levels The specific metabolic pathways that CI
Transport and Distribution
The transport and distribution of CIIt is known that reactive dyes can interact with various transporters and binding proteins . They can also have effects on their localization or accumulation The specific transport and distribution of CI
Subcellular Localization
The subcellular localization of CIIt is known that reactive dyes can be directed to specific compartments or organelles through targeting signals or post-translational modifications The specific subcellular localization of CI
Biologische Aktivität
C.I. Reactive Red 2 is a synthetic dye commonly used in textile industries due to its vibrant color and ease of application. However, its widespread use raises concerns regarding its environmental impact and biological activity. This article explores the biological activity of this compound, including its effects on various organisms, mechanisms of degradation, and potential applications in bioremediation.
This compound is classified as a reactive dye with the chemical formula . It is characterized by its high solubility in water and ability to form covalent bonds with textile fibers. The dye's structure includes multiple functional groups that contribute to its reactivity and interaction with biological systems.
Toxicity and Ecotoxicology
Research indicates that this compound exhibits varying degrees of toxicity to aquatic organisms. For instance, studies have shown that exposure to this dye can lead to significant mortality rates in fish and invertebrates, disrupting aquatic ecosystems . The toxic effects are attributed to the dye's ability to induce oxidative stress, leading to cellular damage.
Table 1: Toxicity of this compound on Aquatic Organisms
Organism | LC50 (mg/L) | Observations |
---|---|---|
Fish (e.g., Carassius auratus) | 12.5 | Significant mortality at concentrations above LC50 |
Daphnia magna | 5.0 | Reduced reproduction and mobility |
Algae (e.g., Chlorella vulgaris) | 10.0 | Inhibition of growth observed |
Biodegradation Mechanisms
The biodegradation of this compound has been extensively studied, particularly using fungal species known for their ligninolytic enzyme production. White rot fungi, such as Phanerochaete chrysosporium, have shown remarkable capabilities in decolorizing this dye through enzymatic pathways involving laccase and manganese peroxidase .
Table 2: Decolorization Efficiency of Fungi on this compound
Fungal Species | % Decolorization | Enzyme Activity (U/ml) |
---|---|---|
Phanerochaete chrysosporium | 95% | Laccase: 250 |
Trametes versicolor | 85% | MnP: 120 |
Ganoderma lucidum | 78% | Laccase: 200 |
Case Studies
- Fungal Bioremediation : A study demonstrated the use of Phanerochaete chrysosporium for the effective decolorization of this compound in wastewater treatment systems. The research highlighted that under optimal conditions (pH 5, temperature 30°C), the fungus achieved over 95% decolorization within five days .
- Ozone Treatment : Another investigation explored the combination of ozone treatment with Fenton-like processes to enhance the degradation of this compound. Results indicated that this hybrid approach significantly improved decolorization rates compared to traditional methods, achieving over 90% removal within a short period .
Applications in Environmental Remediation
The biological activity of this compound has implications for environmental remediation strategies. The ability of certain fungi to degrade this dye presents opportunities for developing sustainable wastewater treatment solutions that minimize environmental impact while effectively removing contaminants.
Q & A
Basic Research Questions
Q. What are the key physicochemical properties of C.I. Reactive Red 2 that influence its reactivity in aqueous solutions?
this compound (RR2) is an azo dye characterized by sulfonic acid groups, which enhance its solubility in water. Key properties include its absorption maxima (λmax) in the visible spectrum (e.g., ~540 nm), pH-dependent stability, and redox behavior. Researchers should measure its logP (partition coefficient) to assess hydrophobicity and use techniques like UV-Vis spectroscopy to monitor degradation kinetics. Stability under varying pH (e.g., 3–10) must be tested to determine optimal reaction conditions for studies involving photodegradation or catalytic oxidation .
Q. How can researchers design experiments to synthesize and characterize this compound derivatives for enhanced stability?
Synthesis of derivatives often involves modifying the azo bond or sulfonic groups. A methodological approach includes:
Synthesis : Use diazotization-coupling reactions under controlled pH (e.g., 0–5°C, pH 8–10).
Characterization : Employ FTIR to confirm functional groups, XRD for crystallinity, and SEM for surface morphology.
Stability Testing : Compare degradation rates under UV light or oxidizing agents (e.g., H2O2) .
Advanced Research Questions
Q. How can conflicting data on the degradation efficiency of this compound using heterogeneous Fenton-like catalysts be resolved?
Discrepancies often arise from variations in catalyst preparation (e.g., Fe loading in ZSM-5 zeolites) or reaction conditions (pH, H2O2 concentration). To address contradictions:
- Systematic Replication : Reproduce studies using identical catalysts (e.g., FeZSM-5 with 42 Fe ions) and parameters (pH 3.5, 0.067 M H2O2).
- Kinetic Modeling : Apply rate equations (e.g., −rA₀ = 9.4×10⁴e^(−38.8/RT)CRR141,0CH2O2,0^0.184) to compare theoretical and experimental degradation rates.
- Leachate Analysis : Monitor Fe ion leakage via ICP-AES to distinguish homogeneous vs. heterogeneous catalytic contributions .
Q. What experimental strategies optimize the mineralization of this compound while minimizing secondary pollution from iron-based catalysts?
Mineralization requires balancing catalyst efficiency and Fe leaching:
Catalyst Optimization : Use FeZSM-5 with higher Si/Al ratios to reduce Fe leaching.
Hybrid Systems : Combine Fenton-like oxidation with adsorption (e.g., activated carbon) to capture leached Fe ions.
Operando Spectroscopy : Track intermediate byproducts (e.g., aromatic amines) via LC-MS to ensure complete mineralization .
Q. Methodological Challenges
Q. How should researchers design controlled experiments to isolate the effects of pH on this compound degradation kinetics?
- Variable Isolation : Fix all parameters (e.g., H2O2 concentration, temperature) while varying pH (2–10).
- Buffer Selection : Use non-complexing buffers (e.g., phosphate for pH 3–7, borate for pH 8–10) to avoid side reactions.
- Kinetic Profiling : Collect time-resolved UV-Vis data to calculate pseudo-first-order rate constants (kobs) at each pH .
Q. What statistical approaches are recommended for analyzing contradictory data on catalytic efficiency across studies?
- Meta-Analysis : Pool datasets from published studies and apply ANOVA to identify significant variables (e.g., catalyst type, H2O2 concentration).
- Sensitivity Analysis : Use Monte Carlo simulations to quantify uncertainty in kinetic parameters (e.g., activation energy).
- Machine Learning : Train models on experimental conditions to predict optimal degradation parameters .
Q. Data Interpretation and Validation
Q. How can researchers validate the completeness of this compound degradation in complex wastewater matrices?
- TOC vs. COD : Measure Total Organic Carbon (TOC) to confirm mineralization, not just decolorization (COD reduction).
- Toxicity Assays : Use bioassays (e.g., Daphnia magna mortality) to ensure degradation byproducts are non-toxic.
- Cross-Validation : Compare HPLC and UV-Vis results to rule out interference from matrix components .
Q. Experimental Design Tables
Parameter | Optimal Range for Degradation | Key Techniques |
---|---|---|
pH | 3.5–4.5 | pH-stat, UV-Vis kinetics |
H2O2 Concentration | 0.007–0.067 M | Titration, kinetic modeling |
Catalyst Loading (FeZSM-5) | 0.5–2.0 g/L | ICP-AES, SEM-EDS |
Temperature | 298–333 K | Arrhenius plot analysis |
Vergleich Mit ähnlichen Verbindungen
Reactive Red 141 (RR141)
Structural Comparison :
- Molecular Formula : C₅₂H₂₂Na₈O₂₆S₈Cl₂N₁₄ (MW = 1774 g/mol) .
- Reactive Groups: Two monochlorotriazine groups, similar to RR2 .
- Chromophore : Azo group, but with additional sulfonic substituents enhancing water solubility .
Degradation Efficiency :
- Heterogeneous Fenton-like oxidation using Fe-ZSM-5 zeolite achieves 100% decolorization and 81% COD reduction in 2 hours at pH 3.5 and 333 K .
- RR141 requires higher H₂O₂ concentrations (0.267 M) compared to RR2 (0.033 M in Fenton systems) for optimal degradation .
Key Difference : RR141’s larger molecular size and sulfonic groups increase solubility but reduce adsorption efficiency in physical treatments .
Reactive Red 195 (RR195)
Functional Comparison :
- Chromophore : Azo group with five sulfonic substituents , making it highly water-soluble and challenging to decolorize .
- Degradation : Electrocoagulation with iron electrodes achieves 100% color removal for RR195, similar to RR2’s performance in ozone systems .
Contrast : RR195’s multiple sulfonic groups hinder chemical oxidation efficiency, whereas RR2’s simpler structure allows faster degradation in AOPs .
Reactive Blue 4 (RB4) and RR2 in Fenton Systems
A comparative study using Fenton oxidation ([Fe²⁺]/[H₂O₂] = 1:20, pH 3) showed:
Parameter | RR2 | RB4 |
---|---|---|
Color Removal | 99.8% | 99.5% |
TOC Reduction | 39.8–42.5% | 35–40% |
Rate Constant | 0.112 min⁻¹ | 0.095 min⁻¹ |
RR2 degrades faster due to its simpler aromatic structure, while RB4’s anthraquinone chromophore resists mineralization .
Reactive Red 239 (RR239)
Photocatalytic Degradation :
- Under UV/TiO₂, RR239 (1085.84 g/mol) shows 97% degradation at 30 mg/L, outperforming RR2 (95%) under similar conditions .
Data Tables
Table 1: Structural and Degradation Comparison
Research Findings
- RR2 vs. RR141 : RR2’s smaller size and fewer sulfonic groups enable faster degradation but lower COD removal compared to RR141 .
- Energy Efficiency : Ozone systems for RR2 consume 0.5–1.2 kWh/m³ , while heterogeneous Fenton for RR141 requires higher catalyst loads (0.3 g/L) .
- Environmental Impact : RR2 generates fewer toxic intermediates than RR195 during oxidation, as sulfonic groups in RR195 prolong degradation .
Vorbereitungsmethoden
Diazotization and Coupling Reactions in RR2 Synthesis
Diazotization of Primary Aromatic Amines
The synthesis begins with diazotization, where a primary aromatic amine (e.g., aniline derivative) reacts with nitrous acid (HNO₂) in acidic medium to form a diazonium salt. Key parameters include:
-
Temperature : Maintained at 0–5°C to prevent diazonium salt decomposition.
-
Acid concentration : Excess HCl (2–3 M) stabilizes the diazonium ion .
For RR2, the amine precursor is typically 4-amino-5-hydroxy-2,7-naphthalenedisulfonic acid, which undergoes diazotization to yield a stable intermediate.
Azo Coupling with Naphthol Derivatives
The diazonium salt is coupled with a naphthol derivative (e.g., 1-naphthol-3,6-disulfonic acid) under alkaline conditions (pH 8–10). This step forms the azo chromophore:
Alkaline conditions deprotonate the naphthol, enhancing its nucleophilicity for electrophilic aromatic substitution .
Introduction of Reactive Groups
Triazination with Cyanuric Chloride
The intermediate azo compound reacts with cyanuric chloride (C₃N₃Cl₃) to introduce the reactive chlorotriazine group. This step occurs in a non-aqueous medium (e.g., acetone) at 0–5°C:
Excess cyanuric chloride is used to ensure complete triazination, followed by neutralization to remove HCl byproducts .
Sulfonation for Enhanced Solubility
Post-triazination, sulfonic acid groups are introduced via sulfonation with oleum (H₂SO₄·SO₃). Reaction conditions include:
-
Temperature : 80–100°C
-
Time : 2–4 hours
Sulfonation degrees are monitored via titration to ensure optimal solubility without over-sulfonation, which reduces dye affinity .
Purification and Isolation
Crude RR2 is purified through:
-
Salt precipitation : Addition of NaCl or Na₂SO₄ to precipitate the dye.
-
Filtration and washing : Removes unreacted sulfonating agents and salts.
-
Drying : Spray-drying at 120–150°C yields a powdered product with <5% moisture content .
Optimization of Synthesis Parameters
Effect of pH on Coupling Efficiency
Studies show that coupling efficiency peaks at pH 9.0, achieving 92% yield (Table 1). Lower pH (<7) slows deprotonation of naphthol, while higher pH (>10) risks diazonium salt hydrolysis .
Table 1. Coupling Efficiency vs. pH
pH | Yield (%) |
---|---|
7 | 68 |
8 | 85 |
9 | 92 |
10 | 88 |
Temperature Control in Triazination
Triazination at 5°C minimizes side reactions (e.g., hydrolysis of cyanuric chloride), yielding 89% pure product versus 72% at 25°C .
Analytical Validation of Synthesis
LC-ESI-MS/MS Characterization
Post-synthesis analysis using LC-ESI-MS/MS identifies cleavage products (Table 2). For RR2, reductive cleavage with SnCl₂/HCl yields a primary amine (m/z 94), confirming the azo linkage’s integrity .
Table 2. Key Cleavage Products of RR2
Reactive Dye | Cleavage Product | m/z |
---|---|---|
Reactive Red 2 | AN | 94 |
TOC Monitoring in Waste Streams
Total Organic Carbon (TOC) analysis during synthesis reveals 85% mineralization efficiency, ensuring environmentally compliant waste management .
Industrial-Scale Production Challenges
Eigenschaften
IUPAC Name |
disodium;5-[(4,6-dichloro-1,3,5-triazin-2-yl)amino]-4-hydroxy-3-phenyldiazenylnaphthalene-2,7-disulfonate | |
---|---|---|
Source | PubChem | |
URL | https://pubchem.ncbi.nlm.nih.gov | |
Description | Data deposited in or computed by PubChem | |
InChI |
InChI=1S/C19H12Cl2N6O7S2.2Na/c20-17-23-18(21)25-19(24-17)22-12-8-11(35(29,30)31)6-9-7-13(36(32,33)34)15(16(28)14(9)12)27-26-10-4-2-1-3-5-10;;/h1-8,28H,(H,29,30,31)(H,32,33,34)(H,22,23,24,25);;/q;2*+1/p-2 | |
Source | PubChem | |
URL | https://pubchem.ncbi.nlm.nih.gov | |
Description | Data deposited in or computed by PubChem | |
InChI Key |
XWZDJOJCYUSIEY-UHFFFAOYSA-L | |
Source | PubChem | |
URL | https://pubchem.ncbi.nlm.nih.gov | |
Description | Data deposited in or computed by PubChem | |
Canonical SMILES |
C1=CC=C(C=C1)N=NC2=C(C3=C(C=C(C=C3C=C2S(=O)(=O)[O-])S(=O)(=O)[O-])NC4=NC(=NC(=N4)Cl)Cl)O.[Na+].[Na+] | |
Source | PubChem | |
URL | https://pubchem.ncbi.nlm.nih.gov | |
Description | Data deposited in or computed by PubChem | |
Molecular Formula |
C19H10Cl2N6Na2O7S2 | |
Source | PubChem | |
URL | https://pubchem.ncbi.nlm.nih.gov | |
Description | Data deposited in or computed by PubChem | |
Related CAS |
6522-86-7 (Parent) | |
Record name | C.I. Reactive Red 2 | |
Source | ChemIDplus | |
URL | https://pubchem.ncbi.nlm.nih.gov/substance/?source=chemidplus&sourceid=0017804498 | |
Description | ChemIDplus is a free, web search system that provides access to the structure and nomenclature authority files used for the identification of chemical substances cited in National Library of Medicine (NLM) databases, including the TOXNET system. | |
Record name | 2,7-Naphthalenedisulfonic acid, 5-((4,6-dichloro-1,3,5-triazin-2-yl)amino)-4-hydroxy-3-(2-phenyldiazenyl)-, sodium salt (1:?) | |
Source | ChemIDplus | |
URL | https://pubchem.ncbi.nlm.nih.gov/substance/?source=chemidplus&sourceid=0106740696 | |
Description | ChemIDplus is a free, web search system that provides access to the structure and nomenclature authority files used for the identification of chemical substances cited in National Library of Medicine (NLM) databases, including the TOXNET system. | |
DSSTOX Substance ID |
DTXSID10889666, DTXSID70924123 | |
Record name | C.I. Reactive Red 2 | |
Source | EPA DSSTox | |
URL | https://comptox.epa.gov/dashboard/DTXSID10889666 | |
Description | DSSTox provides a high quality public chemistry resource for supporting improved predictive toxicology. | |
Record name | Disodium 5-[(4,6-dichloro-1,3,5-triazin-2(1H)-ylidene)amino]-4-oxo-3-(2-phenylhydrazinylidene)-3,4-dihydronaphthalene-2,7-disulfonate | |
Source | EPA DSSTox | |
URL | https://comptox.epa.gov/dashboard/DTXSID70924123 | |
Description | DSSTox provides a high quality public chemistry resource for supporting improved predictive toxicology. | |
Molecular Weight |
615.3 g/mol | |
Source | PubChem | |
URL | https://pubchem.ncbi.nlm.nih.gov | |
Description | Data deposited in or computed by PubChem | |
CAS No. |
17804-49-8, 106740-69-6, 12226-03-8 | |
Record name | C.I. Reactive Red 2 | |
Source | ChemIDplus | |
URL | https://pubchem.ncbi.nlm.nih.gov/substance/?source=chemidplus&sourceid=0017804498 | |
Description | ChemIDplus is a free, web search system that provides access to the structure and nomenclature authority files used for the identification of chemical substances cited in National Library of Medicine (NLM) databases, including the TOXNET system. | |
Record name | 2,7-Naphthalenedisulfonic acid, 5-((4,6-dichloro-1,3,5-triazin-2-yl)amino)-4-hydroxy-3-(2-phenyldiazenyl)-, sodium salt (1:?) | |
Source | ChemIDplus | |
URL | https://pubchem.ncbi.nlm.nih.gov/substance/?source=chemidplus&sourceid=0106740696 | |
Description | ChemIDplus is a free, web search system that provides access to the structure and nomenclature authority files used for the identification of chemical substances cited in National Library of Medicine (NLM) databases, including the TOXNET system. | |
Record name | 2,7-Naphthalenedisulfonic acid, 5-[(4,6-dichloro-1,3,5-triazin-2-yl)amino]-4-hydroxy-3-(2-phenyldiazenyl)-, sodium salt (1:?) | |
Source | EPA Chemicals under the TSCA | |
URL | https://www.epa.gov/chemicals-under-tsca | |
Description | EPA Chemicals under the Toxic Substances Control Act (TSCA) collection contains information on chemicals and their regulations under TSCA, including non-confidential content from the TSCA Chemical Substance Inventory and Chemical Data Reporting. | |
Record name | 2,7-Naphthalenedisulfonic acid, 5-[(4,6-dichloro-1,3,5-triazin-2-yl)amino]-4-hydroxy-3-(2-phenyldiazenyl)-, sodium salt (1:2) | |
Source | EPA Chemicals under the TSCA | |
URL | https://www.epa.gov/chemicals-under-tsca | |
Description | EPA Chemicals under the Toxic Substances Control Act (TSCA) collection contains information on chemicals and their regulations under TSCA, including non-confidential content from the TSCA Chemical Substance Inventory and Chemical Data Reporting. | |
Record name | C.I. Reactive Red 2 | |
Source | EPA DSSTox | |
URL | https://comptox.epa.gov/dashboard/DTXSID10889666 | |
Description | DSSTox provides a high quality public chemistry resource for supporting improved predictive toxicology. | |
Record name | Disodium 5-[(4,6-dichloro-1,3,5-triazin-2(1H)-ylidene)amino]-4-oxo-3-(2-phenylhydrazinylidene)-3,4-dihydronaphthalene-2,7-disulfonate | |
Source | EPA DSSTox | |
URL | https://comptox.epa.gov/dashboard/DTXSID70924123 | |
Description | DSSTox provides a high quality public chemistry resource for supporting improved predictive toxicology. | |
Record name | Disodium 5-[(4,6-dichloro-1,3,5-triazin-2-yl)amino]-4-hydroxy-3-(phenylazo)naphthalene-2,7-disulphonate | |
Source | European Chemicals Agency (ECHA) | |
URL | https://echa.europa.eu/substance-information/-/substanceinfo/100.037.963 | |
Description | The European Chemicals Agency (ECHA) is an agency of the European Union which is the driving force among regulatory authorities in implementing the EU's groundbreaking chemicals legislation for the benefit of human health and the environment as well as for innovation and competitiveness. | |
Explanation | Use of the information, documents and data from the ECHA website is subject to the terms and conditions of this Legal Notice, and subject to other binding limitations provided for under applicable law, the information, documents and data made available on the ECHA website may be reproduced, distributed and/or used, totally or in part, for non-commercial purposes provided that ECHA is acknowledged as the source: "Source: European Chemicals Agency, http://echa.europa.eu/". Such acknowledgement must be included in each copy of the material. ECHA permits and encourages organisations and individuals to create links to the ECHA website under the following cumulative conditions: Links can only be made to webpages that provide a link to the Legal Notice page. | |
Retrosynthesis Analysis
AI-Powered Synthesis Planning: Our tool employs the Template_relevance Pistachio, Template_relevance Bkms_metabolic, Template_relevance Pistachio_ringbreaker, Template_relevance Reaxys, Template_relevance Reaxys_biocatalysis model, leveraging a vast database of chemical reactions to predict feasible synthetic routes.
One-Step Synthesis Focus: Specifically designed for one-step synthesis, it provides concise and direct routes for your target compounds, streamlining the synthesis process.
Accurate Predictions: Utilizing the extensive PISTACHIO, BKMS_METABOLIC, PISTACHIO_RINGBREAKER, REAXYS, REAXYS_BIOCATALYSIS database, our tool offers high-accuracy predictions, reflecting the latest in chemical research and data.
Strategy Settings
Precursor scoring | Relevance Heuristic |
---|---|
Min. plausibility | 0.01 |
Model | Template_relevance |
Template Set | Pistachio/Bkms_metabolic/Pistachio_ringbreaker/Reaxys/Reaxys_biocatalysis |
Top-N result to add to graph | 6 |
Feasible Synthetic Routes
Haftungsausschluss und Informationen zu In-Vitro-Forschungsprodukten
Bitte beachten Sie, dass alle Artikel und Produktinformationen, die auf BenchChem präsentiert werden, ausschließlich zu Informationszwecken bestimmt sind. Die auf BenchChem zum Kauf angebotenen Produkte sind speziell für In-vitro-Studien konzipiert, die außerhalb lebender Organismen durchgeführt werden. In-vitro-Studien, abgeleitet von dem lateinischen Begriff "in Glas", beinhalten Experimente, die in kontrollierten Laborumgebungen unter Verwendung von Zellen oder Geweben durchgeführt werden. Es ist wichtig zu beachten, dass diese Produkte nicht als Arzneimittel oder Medikamente eingestuft sind und keine Zulassung der FDA für die Vorbeugung, Behandlung oder Heilung von medizinischen Zuständen, Beschwerden oder Krankheiten erhalten haben. Wir müssen betonen, dass jede Form der körperlichen Einführung dieser Produkte in Menschen oder Tiere gesetzlich strikt untersagt ist. Es ist unerlässlich, sich an diese Richtlinien zu halten, um die Einhaltung rechtlicher und ethischer Standards in Forschung und Experiment zu gewährleisten.