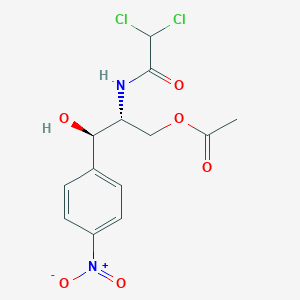
Chloramphenicol-3-acetat
Übersicht
Beschreibung
Chloramphenicol Acetate is a derivative of Chloramphenicol, a broad-spectrum antibiotic originally isolated from Streptomyces venezuelae in 1947. Chloramphenicol Acetate is synthesized to enhance the properties of Chloramphenicol, particularly its stability and solubility. It is primarily used in the medical field to treat various bacterial infections by inhibiting protein synthesis in bacteria .
Wissenschaftliche Forschungsanwendungen
Chloramphenicol-Acetat hat eine breite Palette von Anwendungen in der wissenschaftlichen Forschung:
Chemie: Wird als Modellverbindung zur Untersuchung von Acetylierungsreaktionen und der Stabilität von acetylierten Antibiotika verwendet.
Biologie: Wird in Studien zur bakteriellen Proteinsynthese und Resistenzmechanismen eingesetzt.
Medizin: Wird bei der Entwicklung von Antibiotikaformulierungen und bei der Behandlung von bakteriellen Infektionen eingesetzt, die gegen andere Antibiotika resistent sind.
5. Wirkmechanismus
Chloramphenicol-Acetat entfaltet seine Wirkung, indem es die bakterielle Proteinsynthese hemmt. Es bindet an die 50S-ribosomale Untereinheit von bakteriellen Ribosomen und verhindert so die Bildung von Peptidbindungen während der Translation. Diese Wirkung stoppt effektiv das Wachstum und die Vermehrung von Bakterien. Die molekularen Ziele umfassen das Peptidyltransferase-Zentrum des Ribosoms, das für die Proteinverlängerung entscheidend ist .
Ähnliche Verbindungen:
Chloramphenicol: Die Stammverbindung, bekannt für ihre Breitbandantibiotika-Aktivität.
Chloramphenicol-Succinat: Ein Prodrug von Chloramphenicol, das für die intravenöse Verabreichung verwendet wird.
Thiamphenicol: Ein Derivat mit ähnlichen antibakteriellen Eigenschaften, jedoch mit einem anderen Sicherheitsprofil.
Einzigartigkeit von Chloramphenicol-Acetat: Chloramphenicol-Acetat ist aufgrund seiner verbesserten Stabilität und Löslichkeit im Vergleich zu Chloramphenicol einzigartig. Dies macht es für bestimmte pharmazeutische Formulierungen und Anwendungen besser geeignet, bei denen Stabilität ein entscheidender Faktor ist .
Wirkmechanismus
Target of Action
Chloramphenicol 3-acetate, also known as chloramphenicol acetate, primarily targets the bacterial ribosome . The compound binds to the 23S rRNA on the 50S subunit of the bacterial ribosome . This interaction inhibits the action of peptidyl transferase , an enzyme that plays a crucial role in protein synthesis .
Mode of Action
Chloramphenicol 3-acetate interacts with its targets by binding to the bacterial ribosome and blocking peptidyl transferase . This action inhibits protein synthesis, thereby stopping bacterial growth . The compound is lipid-soluble, which allows it to diffuse through the bacterial cell membrane .
Biochemical Pathways
The initial biotransformation steps of chloramphenicol include the oxidization at the C1-OH and C3-OH groups, the isomerization at C2, and the acetylation at C3-OH . Among these, the isomerization represents a newly discovered biotransformation pathway of chloramphenicol . A novel glucose-methanol-choline oxidoreductase has been identified as responsible for the oxidization of the C3-OH group in certain bacterial species .
Pharmacokinetics
The pharmacokinetics of chloramphenicol 3-acetate, including its Absorption, Distribution, Metabolism, and Excretion (ADME) properties, are crucial for understanding its bioavailability. Chloramphenicol is known to be lipid-soluble, which allows it to diffuse through the bacterial cell membrane . .
Result of Action
The primary result of chloramphenicol 3-acetate’s action is the inhibition of bacterial growth . By binding to the bacterial ribosome and blocking peptidyl transferase, the compound inhibits protein synthesis, effectively stopping the growth of the bacteria .
Action Environment
Environmental factors can influence the action, efficacy, and stability of chloramphenicol 3-acetate. For instance, the compound is prone to be released into environments, resulting in the disturbance of ecosystem stability and the emergence of antibiotic resistance genes . Microbes play a vital role in the decomposition of chloramphenicol in the environment, and the biotransformation processes are especially dependent on synergistic interactions and metabolite exchanges among microbes .
Biochemische Analyse
Biochemical Properties
Chloramphenicol 3-acetate plays a role in biochemical reactions, particularly in the biotransformation of chloramphenicol . The initial biotransformation steps involve the oxidation at the C1-OH and C3-OH groups, the isomerization at C2, and the acetylation at C3-OH of chloramphenicol . A novel glucose-methanol-choline oxidoreductase was identified as responsible for the oxidation of the C3-OH group in Sphingomonas sp. and Caballeronia sp .
Cellular Effects
Chloramphenicol 3-acetate has effects on various types of cells and cellular processes. It influences cell function by interfering with bacterial protein synthesis . It binds to the bacterial ribosome, blocking peptidyl transferase, and inhibiting protein synthesis .
Molecular Mechanism
The molecular mechanism of action of chloramphenicol 3-acetate involves its ability to diffuse through the bacterial cell membrane due to its lipid solubility . It stops bacterial growth by binding to the bacterial ribosome and inhibiting protein synthesis .
Temporal Effects in Laboratory Settings
The effects of chloramphenicol 3-acetate change over time in laboratory settings. The initial biotransformation steps were the oxidization at the C1-OH and C3-OH groups, the isomerization at C2, and the acetylation at C3-OH of chloramphenicol . The isomerization is an entirely new biotransformation pathway of chloramphenicol discovered for the first time .
Dosage Effects in Animal Models
The effects of chloramphenicol 3-acetate vary with different dosages in animal models. In a study using nude mice, the cell viability of the liver, kidney, and heart tissues decreased significantly at the highest dose (100mg/kg body weight) . Histological findings indicated serious organ damage at the maximum dosage .
Metabolic Pathways
Chloramphenicol 3-acetate is involved in metabolic pathways. The initial biotransformation steps were the oxidization at the C1-OH and C3-OH groups, the isomerization at C2, and the acetylation at C3-OH of chloramphenicol . A novel glucose-methanol-choline oxidoreductase was identified as responsible for the oxidation of the C3-OH group in Sphingomonas sp. and Caballeronia sp .
Transport and Distribution
Chloramphenicol 3-acetate is transported and distributed within cells and tissues. The expression of acetate symporter (actP) located at the genome of Cupriavidus sp. was significantly upregulated during chloramphenicol biotransformation . Gene actP is responsible for the transmembrane transport of acetate .
Vorbereitungsmethoden
Synthetic Routes and Reaction Conditions: Chloramphenicol Acetate is synthesized through the acetylation of Chloramphenicol. The process involves the reaction of Chloramphenicol with acetic anhydride in the presence of a base such as pyridine. The reaction typically occurs at room temperature and yields Chloramphenicol Acetate as the primary product .
Industrial Production Methods: In industrial settings, the production of Chloramphenicol Acetate follows a similar acetylation process but on a larger scale. The reaction conditions are optimized to ensure high yield and purity. The use of continuous flow reactors and advanced purification techniques such as crystallization and chromatography are common in industrial production .
Analyse Chemischer Reaktionen
Arten von Reaktionen: Chloramphenicol-Acetat unterliegt verschiedenen chemischen Reaktionen, darunter:
Hydrolyse: In Gegenwart von Wasser oder wässrigen Lösungen kann Chloramphenicol-Acetat zu Chloramphenicol und Essigsäure hydrolysieren.
Oxidation: Es kann Oxidationsreaktionen, insbesondere an den Hydroxylgruppen, eingehen, die zur Bildung von Chinonderivaten führen.
Reduktion: Reduktionsreaktionen können an der Nitrogruppe auftreten, wodurch sie in eine Aminogruppe umgewandelt wird.
Häufige Reagenzien und Bedingungen:
Hydrolyse: Wasser oder verdünnte Säuren/Basen.
Oxidation: Oxidationsmittel wie Kaliumpermanganat oder Wasserstoffperoxid.
Reduktion: Reduktionsmittel wie Natriumborhydrid oder katalytische Hydrierung.
Hauptsächlich gebildete Produkte:
Hydrolyse: Chloramphenicol und Essigsäure.
Oxidation: Chinonderivate.
Reduktion: Aminoverbindungen von Chloramphenicol.
Vergleich Mit ähnlichen Verbindungen
Chloramphenicol: The parent compound, known for its broad-spectrum antibiotic activity.
Chloramphenicol Succinate: A prodrug of Chloramphenicol, used for intravenous administration.
Thiamphenicol: A derivative with similar antibacterial properties but with a different safety profile.
Uniqueness of Chloramphenicol Acetate: Chloramphenicol Acetate is unique due to its enhanced stability and solubility compared to Chloramphenicol. This makes it more suitable for certain pharmaceutical formulations and applications where stability is a critical factor .
Eigenschaften
IUPAC Name |
[(2R,3R)-2-[(2,2-dichloroacetyl)amino]-3-hydroxy-3-(4-nitrophenyl)propyl] acetate | |
---|---|---|
Source | PubChem | |
URL | https://pubchem.ncbi.nlm.nih.gov | |
Description | Data deposited in or computed by PubChem | |
InChI |
InChI=1S/C13H14Cl2N2O6/c1-7(18)23-6-10(16-13(20)12(14)15)11(19)8-2-4-9(5-3-8)17(21)22/h2-5,10-12,19H,6H2,1H3,(H,16,20)/t10-,11-/m1/s1 | |
Source | PubChem | |
URL | https://pubchem.ncbi.nlm.nih.gov | |
Description | Data deposited in or computed by PubChem | |
InChI Key |
VVOIFRARHIZCJD-GHMZBOCLSA-N | |
Source | PubChem | |
URL | https://pubchem.ncbi.nlm.nih.gov | |
Description | Data deposited in or computed by PubChem | |
Canonical SMILES |
CC(=O)OCC(C(C1=CC=C(C=C1)[N+](=O)[O-])O)NC(=O)C(Cl)Cl | |
Source | PubChem | |
URL | https://pubchem.ncbi.nlm.nih.gov | |
Description | Data deposited in or computed by PubChem | |
Isomeric SMILES |
CC(=O)OC[C@H]([C@@H](C1=CC=C(C=C1)[N+](=O)[O-])O)NC(=O)C(Cl)Cl | |
Source | PubChem | |
URL | https://pubchem.ncbi.nlm.nih.gov | |
Description | Data deposited in or computed by PubChem | |
Molecular Formula |
C13H14Cl2N2O6 | |
Source | PubChem | |
URL | https://pubchem.ncbi.nlm.nih.gov | |
Description | Data deposited in or computed by PubChem | |
DSSTOX Substance ID |
DTXSID30908222 | |
Record name | N-[3-(Acetyloxy)-1-hydroxy-1-(4-nitrophenyl)propan-2-yl]-2,2-dichloroethanimidic acid | |
Source | EPA DSSTox | |
URL | https://comptox.epa.gov/dashboard/DTXSID30908222 | |
Description | DSSTox provides a high quality public chemistry resource for supporting improved predictive toxicology. | |
Molecular Weight |
365.16 g/mol | |
Source | PubChem | |
URL | https://pubchem.ncbi.nlm.nih.gov | |
Description | Data deposited in or computed by PubChem | |
CAS No. |
10318-16-8 | |
Record name | N-[(1R,2R)-1-[(Acetyloxy)methyl]-2-hydroxy-2-(4-nitrophenyl)ethyl]-2,2-dichloroacetamide | |
Source | CAS Common Chemistry | |
URL | https://commonchemistry.cas.org/detail?cas_rn=10318-16-8 | |
Description | CAS Common Chemistry is an open community resource for accessing chemical information. Nearly 500,000 chemical substances from CAS REGISTRY cover areas of community interest, including common and frequently regulated chemicals, and those relevant to high school and undergraduate chemistry classes. This chemical information, curated by our expert scientists, is provided in alignment with our mission as a division of the American Chemical Society. | |
Explanation | The data from CAS Common Chemistry is provided under a CC-BY-NC 4.0 license, unless otherwise stated. | |
Record name | Chloramphenicol 3-acetate | |
Source | ChemIDplus | |
URL | https://pubchem.ncbi.nlm.nih.gov/substance/?source=chemidplus&sourceid=0010318168 | |
Description | ChemIDplus is a free, web search system that provides access to the structure and nomenclature authority files used for the identification of chemical substances cited in National Library of Medicine (NLM) databases, including the TOXNET system. | |
Record name | N-[3-(Acetyloxy)-1-hydroxy-1-(4-nitrophenyl)propan-2-yl]-2,2-dichloroethanimidic acid | |
Source | EPA DSSTox | |
URL | https://comptox.epa.gov/dashboard/DTXSID30908222 | |
Description | DSSTox provides a high quality public chemistry resource for supporting improved predictive toxicology. | |
Retrosynthesis Analysis
AI-Powered Synthesis Planning: Our tool employs the Template_relevance Pistachio, Template_relevance Bkms_metabolic, Template_relevance Pistachio_ringbreaker, Template_relevance Reaxys, Template_relevance Reaxys_biocatalysis model, leveraging a vast database of chemical reactions to predict feasible synthetic routes.
One-Step Synthesis Focus: Specifically designed for one-step synthesis, it provides concise and direct routes for your target compounds, streamlining the synthesis process.
Accurate Predictions: Utilizing the extensive PISTACHIO, BKMS_METABOLIC, PISTACHIO_RINGBREAKER, REAXYS, REAXYS_BIOCATALYSIS database, our tool offers high-accuracy predictions, reflecting the latest in chemical research and data.
Strategy Settings
Precursor scoring | Relevance Heuristic |
---|---|
Min. plausibility | 0.01 |
Model | Template_relevance |
Template Set | Pistachio/Bkms_metabolic/Pistachio_ringbreaker/Reaxys/Reaxys_biocatalysis |
Top-N result to add to graph | 6 |
Feasible Synthetic Routes
Q1: What is the mechanism behind chloramphenicol resistance in bacteria?
A: Many bacteria, including Staphylococcus aureus and Escherichia coli, develop resistance to chloramphenicol by producing an enzyme called chloramphenicol acetyltransferase (CAT). This enzyme adds an acetyl group to chloramphenicol, specifically at the 3-hydroxyl group, forming chloramphenicol 3-acetate. [, , ] This modification prevents chloramphenicol from binding to its target, the bacterial ribosome, thereby inactivating the antibiotic. [, ] Interestingly, chloramphenicol 3-acetate itself does not induce the production of CAT. []
Q2: Are there other mechanisms of chloramphenicol inactivation in bacteria?
A: Yes, besides acetylation, a novel chloramphenicol hydrolase enzyme (EstDL136) has been identified through metagenomic analysis. [, ] This enzyme hydrolyzes chloramphenicol, breaking it down into inactive compounds, one of which has been identified as p-nitrophenylserinol. [] Notably, this enzyme also inactivates florfenicol, another antibiotic, suggesting a broader substrate specificity. []
Q3: How is chloramphenicol acetyltransferase activity typically assayed?
A: A common method for detecting and quantifying CAT activity involves separating chloramphenicol 3-acetate from unreacted chloramphenicol using adsorption chromatography with a neutral alumina column. [] Following separation, chloramphenicol 3-acetate can be quantified either through colorimetric analysis after chemical derivatization or through a microbiological assay after enzymatic deacetylation. []
Q4: Does the production of chloramphenicol acetyltransferase always confer resistance?
A: Interestingly, the presence of CAT might not guarantee chloramphenicol resistance in all microorganisms. Studies on methanogens found that chloramphenicol 3-acetate still inhibited their growth. [] This suggests that the introduction of CAT-encoding genes may not be sufficient to confer resistance in these organisms. []
Q5: Is chloramphenicol 3-acetate found naturally?
A: Chloramphenicol 3-acetate is not solely a product of bacterial resistance mechanisms. Streptomyces griseus, a species known to naturally produce chloramphenicol, possesses chloramphenicol acetyltransferase primarily located on the surface of its spores. [, ] This enzyme specifically catalyzes the formation of chloramphenicol 3-acetate. [] Interestingly, these spores can even utilize chloramphenicol 3-acetate as a substrate for further modifications, adding different acyl groups to generate other chloramphenicol esters. []
Q6: Are there alternative methods to screen for novel enzymes involved in chloramphenicol resistance?
A: Function-driven metagenomic analysis has proven to be a valuable tool for discovering new enzymes involved in antibiotic resistance. [] This approach led to the identification of two novel esterases, EstDL26 and EstDL136, from a soil metagenomic library. [] These esterases can reactivate chloramphenicol from its acetylated forms by counteracting the action of CAT. [] This highlights the potential of metagenomics for uncovering novel resistance mechanisms and developing strategies to overcome them.
Haftungsausschluss und Informationen zu In-Vitro-Forschungsprodukten
Bitte beachten Sie, dass alle Artikel und Produktinformationen, die auf BenchChem präsentiert werden, ausschließlich zu Informationszwecken bestimmt sind. Die auf BenchChem zum Kauf angebotenen Produkte sind speziell für In-vitro-Studien konzipiert, die außerhalb lebender Organismen durchgeführt werden. In-vitro-Studien, abgeleitet von dem lateinischen Begriff "in Glas", beinhalten Experimente, die in kontrollierten Laborumgebungen unter Verwendung von Zellen oder Geweben durchgeführt werden. Es ist wichtig zu beachten, dass diese Produkte nicht als Arzneimittel oder Medikamente eingestuft sind und keine Zulassung der FDA für die Vorbeugung, Behandlung oder Heilung von medizinischen Zuständen, Beschwerden oder Krankheiten erhalten haben. Wir müssen betonen, dass jede Form der körperlichen Einführung dieser Produkte in Menschen oder Tiere gesetzlich strikt untersagt ist. Es ist unerlässlich, sich an diese Richtlinien zu halten, um die Einhaltung rechtlicher und ethischer Standards in Forschung und Experiment zu gewährleisten.