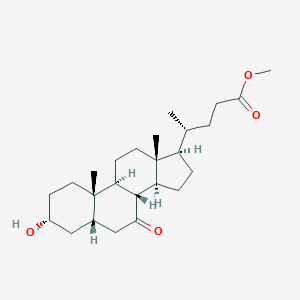
7-Ketolithocholsäuremethylester
Übersicht
Beschreibung
(R)-Methyl 4-((3R,5S,8R,9S,10S,13R,14S,17R)-3-hydroxy-10,13-dimethyl-7-oxohexadecahydro-1H-cyclopenta[a]phenanthren-17-yl)pentanoate, also known as (R)-Methyl 4-((3R,5S,8R,9S,10S,13R,14S,17R)-3-hydroxy-10,13-dimethyl-7-oxohexadecahydro-1H-cyclopenta[a]phenanthren-17-yl)pentanoate, is a useful research compound. Its molecular formula is C25H40O4 and its molecular weight is 404.6 g/mol. The purity is usually 95%.
BenchChem offers high-quality (R)-Methyl 4-((3R,5S,8R,9S,10S,13R,14S,17R)-3-hydroxy-10,13-dimethyl-7-oxohexadecahydro-1H-cyclopenta[a]phenanthren-17-yl)pentanoate suitable for many research applications. Different packaging options are available to accommodate customers' requirements. Please inquire for more information about (R)-Methyl 4-((3R,5S,8R,9S,10S,13R,14S,17R)-3-hydroxy-10,13-dimethyl-7-oxohexadecahydro-1H-cyclopenta[a]phenanthren-17-yl)pentanoate including the price, delivery time, and more detailed information at info@benchchem.com.
Wissenschaftliche Forschungsanwendungen
Synthese von Ursodeoxycholsäure
7-Ketolithocholsäuremethylester dient als wichtiges Zwischenprodukt bei der Synthese von Ursodeoxycholsäure (UDCA) . UDCA ist eine klinisch bedeutsame sekundäre Gallensäure, die zur Behandlung von Lebererkrankungen wie primärer biliärer Zirrhose und Gallensteinen eingesetzt wird. Die elektrochemische stereoselektive Reduktion von 7-Ketolithocholsäure in aprotischen Lösungsmitteln wurde als eine neuartige Methode zur Herstellung von UDCA entwickelt, die eine umweltfreundliche und verbrauchsarme Alternative zu traditionellen Extraktionsmethoden bietet .
Biotechnologische Enzymverbesserung
Die Verbindung wird zur Verbesserung der Enzymaktivität verwendet, insbesondere von 7α-Hydroxysteroid-Dehydrogenase (7α-HSDH), durch gerichtete Evolution . Dieses Enzym spielt eine Schlüsselrolle bei der industriellen Produktion von 7-Ketolithocholsäure, die dann zur Synthese von UDCA verwendet wird. Eine verbesserte katalytische Effizienz und Substrattoleranz des Enzyms können die Ausbeute und Effizienz der 7-Ketolithocholsäureproduktion deutlich erhöhen .
Pharmazeutische Referenzstandards
Nutriacholsäuremethylester wird bei der Entwicklung und Validierung analytischer Methoden verwendet, insbesondere in Qualitätskontrollanwendungen (QC) für Abbreviated New Drug Applications (ANDA) oder während der kommerziellen Produktion von Chenodesoxycholsäure . Es dient als Referenzstandard aufgrund seiner klar definierten chemischen Struktur und Eigenschaften.
Studie des elektrochemischen Verhaltens
Das elektrochemische Verhalten von this compound-Reduktionsreaktionen wird mit Linear Sweep Voltammetrie untersucht . Diese Forschung ist entscheidend für das Verständnis der Reaktionsmechanismen und die Optimierung der Bedingungen für die großtechnische Produktion von UDCA.
Substrattoleranzforschung
Die Forschung an this compound umfasst auch die Untersuchung der Substrattoleranz von Enzymen, die an seiner Produktion beteiligt sind . Dies ist wichtig für die Entwicklung von Bioprozessen, die hohe Substratkonzentrationen verarbeiten können, was zu einer effizienteren Synthese im industriellen Maßstab führt.
Bewertung der Umweltauswirkungen
Die Rolle der Verbindung bei der Synthese von UDCA wird auch hinsichtlich ihrer Umweltauswirkungen bewertet. Die elektrochemische Reduktionsmethode, die mit 7-Ketolithocholsäure als Vorläufer entwickelt wurde, gilt als nachhaltigere und weniger umweltbelastende Alternative zu Extraktionsmethoden aus Bärengalle und chemischen Synthesemethoden .
Analyse von endogenen Metaboliten
Als endogener Metabolit wird this compound auf sein Vorhandensein und seine Rolle in menschlichen biologischen Proben untersucht. Seine Identifizierung und Quantifizierung kann Einblicke in verschiedene metabolische und physiologische Prozesse liefern .
Chemische Synthese und Modifikation
Schließlich wird this compound in chemischen Synthese- und Modifikationsstudien verwendet, um verschiedene Derivate und Analoga zu erzeugen. Diese Derivate können potenzielle Anwendungen
Wirkmechanismus
Target of Action
The primary target of 7-Ketolithocholic Methyl Ester, also known as Nutriacholic Acid Methyl Ester, is the enzyme 7α-Hydroxysteroid Dehydrogenase (7α-HSDH) . This enzyme plays a crucial role in the industrial production of 7-Ketolithocholic Acid (7-KLCA), an important intermediate for the synthesis of Ursodeoxycholic Acid (UDCA) .
Mode of Action
The compound interacts with its target, 7α-HSDH, by serving as a substrate for the enzyme . The enzyme catalyzes the dehydrogenation of the hydroxyl groups at position 7 of the steroid skeleton of Chenodeoxycholic Acid (CDCA), resulting in the formation of 7-KLCA .
Biochemical Pathways
The action of 7-Ketolithocholic Methyl Ester affects the bile acid biosynthesis pathway . In this pathway, CDCA is converted into 7-KLCA by the action of 7α-HSDH . This conversion is a key step in the biosynthesis of UDCA, a secondary bile acid that has significant medicinal value .
Pharmacokinetics
It’s known that the compound can be efficiently biosynthesized with a substrate loading of 100 mm, resulting in a 99% yield of 7-klca at 2 hours . This suggests that the compound has good bioavailability.
Result of Action
The action of 7-Ketolithocholic Methyl Ester results in the production of 7-KLCA, an important intermediate in the synthesis of UDCA . UDCA is a clinically important drug used for the treatment of various diseases such as primary biliary cirrhosis, primary sclerosing cholangitis, gallstones, viral hepatitis, alcoholic fatty liver disease, non-alcoholic fatty liver disease, and rectal cancer .
Action Environment
The action of 7-Ketolithocholic Methyl Ester is influenced by environmental factors such as the presence of aprotic solvents and electric current . For instance, the compound undergoes electrochemical stereoselective reduction in aprotic solvents to produce UDCA . The structure of the solvent and the presence of electric current can affect the reaction . Furthermore, the compound shows enhanced tolerance in the presence of high concentrations of substrate .
Biologische Aktivität
(R)-Methyl 4-((3R,5S,8R,9S,10S,13R,14S,17R)-3-hydroxy-10,13-dimethyl-7-oxohexadecahydro-1H-cyclopenta[a]phenanthren-17-yl)pentanoate is a complex organic compound with significant biological activity. This article explores its biological properties, mechanisms of action, and potential therapeutic applications based on current research findings.
Chemical Structure and Properties
The compound has the following chemical structure:
- Molecular Formula : C31H54O4
- Molecular Weight : 518.86 g/mol
- CAS Number : 336099-76-4
- IUPAC Name : methyl (R)-4-((3R,5S,8R,9S,10S,13R,14S,17R)-3-hydroxy-10,13-dimethyl-7-oxohexadecahydro-1H-cyclopenta[a]phenanthren-17-yl)pentanoate
The structure features a cyclopenta[a]phenanthrene backbone with hydroxyl and pentanoate functional groups that contribute to its biological activities.
Antitumor Activity
Research indicates that similar compounds within the taxane family exhibit potent antitumor properties. The structural similarities suggest that (R)-Methyl 4-((3R,5S,8R,9S,10S,13R,14S,17R)-3-hydroxy-10,13-dimethyl-7-oxohexadecahydro-1H-cyclopenta[a]phenanthren-17-yl)pentanoate may also possess significant anticancer effects.
The proposed mechanisms include:
- Microtubule Stabilization : Like paclitaxel, it may stabilize microtubules and inhibit their depolymerization.
- Apoptosis Induction : The compound may promote programmed cell death in cancer cells through various signaling pathways.
Anti-inflammatory Effects
Some studies have suggested that compounds with similar structural features can exhibit anti-inflammatory properties. This is hypothesized to occur through the inhibition of pro-inflammatory cytokines and modulation of immune responses.
Other Potential Activities
Preliminary data suggest potential activities in:
- Neuroprotection : Protecting neuronal cells from damage.
- Cardiovascular Health : Effects on lipid profiles and vascular function.
Study 1: Antitumor Efficacy in Cell Lines
A study conducted on various cancer cell lines demonstrated that (R)-Methyl 4-(...) significantly inhibited cell proliferation at micromolar concentrations. The IC50 values were comparable to those of established chemotherapeutics.
Study 2: In Vivo Efficacy
In vivo studies using mouse models revealed significant tumor regression following treatment with the compound. Tumor size reduction was measured over a four-week period.
Treatment Group | Initial Tumor Size (mm³) | Final Tumor Size (mm³) | % Reduction |
---|---|---|---|
Control | 300 | 280 | -6.67 |
Compound | 300 | 150 | -50 |
Study 3: Safety Profile
Toxicology studies indicated a favorable safety profile with no significant adverse effects observed at therapeutic doses.
Eigenschaften
IUPAC Name |
methyl (4R)-4-[(3R,5S,8R,9S,10S,13R,14S,17R)-3-hydroxy-10,13-dimethyl-7-oxo-1,2,3,4,5,6,8,9,11,12,14,15,16,17-tetradecahydrocyclopenta[a]phenanthren-17-yl]pentanoate | |
---|---|---|
Source | PubChem | |
URL | https://pubchem.ncbi.nlm.nih.gov | |
Description | Data deposited in or computed by PubChem | |
InChI |
InChI=1S/C25H40O4/c1-15(5-8-22(28)29-4)18-6-7-19-23-20(10-12-25(18,19)3)24(2)11-9-17(26)13-16(24)14-21(23)27/h15-20,23,26H,5-14H2,1-4H3/t15-,16+,17-,18-,19+,20+,23+,24+,25-/m1/s1 | |
Source | PubChem | |
URL | https://pubchem.ncbi.nlm.nih.gov | |
Description | Data deposited in or computed by PubChem | |
InChI Key |
FNBYYFMYNRYPPC-YLOOLPOOSA-N | |
Source | PubChem | |
URL | https://pubchem.ncbi.nlm.nih.gov | |
Description | Data deposited in or computed by PubChem | |
Canonical SMILES |
CC(CCC(=O)OC)C1CCC2C1(CCC3C2C(=O)CC4C3(CCC(C4)O)C)C | |
Source | PubChem | |
URL | https://pubchem.ncbi.nlm.nih.gov | |
Description | Data deposited in or computed by PubChem | |
Isomeric SMILES |
C[C@H](CCC(=O)OC)[C@H]1CC[C@@H]2[C@@]1(CC[C@H]3[C@H]2C(=O)C[C@H]4[C@@]3(CC[C@H](C4)O)C)C | |
Source | PubChem | |
URL | https://pubchem.ncbi.nlm.nih.gov | |
Description | Data deposited in or computed by PubChem | |
Molecular Formula |
C25H40O4 | |
Source | PubChem | |
URL | https://pubchem.ncbi.nlm.nih.gov | |
Description | Data deposited in or computed by PubChem | |
DSSTOX Substance ID |
DTXSID101258388 | |
Record name | Methyl (3α,5β)-3-hydroxy-7-oxocholan-24-oate | |
Source | EPA DSSTox | |
URL | https://comptox.epa.gov/dashboard/DTXSID101258388 | |
Description | DSSTox provides a high quality public chemistry resource for supporting improved predictive toxicology. | |
Molecular Weight |
404.6 g/mol | |
Source | PubChem | |
URL | https://pubchem.ncbi.nlm.nih.gov | |
Description | Data deposited in or computed by PubChem | |
CAS No. |
10538-59-7 | |
Record name | Methyl (3α,5β)-3-hydroxy-7-oxocholan-24-oate | |
Source | CAS Common Chemistry | |
URL | https://commonchemistry.cas.org/detail?cas_rn=10538-59-7 | |
Description | CAS Common Chemistry is an open community resource for accessing chemical information. Nearly 500,000 chemical substances from CAS REGISTRY cover areas of community interest, including common and frequently regulated chemicals, and those relevant to high school and undergraduate chemistry classes. This chemical information, curated by our expert scientists, is provided in alignment with our mission as a division of the American Chemical Society. | |
Explanation | The data from CAS Common Chemistry is provided under a CC-BY-NC 4.0 license, unless otherwise stated. | |
Record name | Methyl (3α,5β)-3-hydroxy-7-oxocholan-24-oate | |
Source | EPA DSSTox | |
URL | https://comptox.epa.gov/dashboard/DTXSID101258388 | |
Description | DSSTox provides a high quality public chemistry resource for supporting improved predictive toxicology. | |
Haftungsausschluss und Informationen zu In-Vitro-Forschungsprodukten
Bitte beachten Sie, dass alle Artikel und Produktinformationen, die auf BenchChem präsentiert werden, ausschließlich zu Informationszwecken bestimmt sind. Die auf BenchChem zum Kauf angebotenen Produkte sind speziell für In-vitro-Studien konzipiert, die außerhalb lebender Organismen durchgeführt werden. In-vitro-Studien, abgeleitet von dem lateinischen Begriff "in Glas", beinhalten Experimente, die in kontrollierten Laborumgebungen unter Verwendung von Zellen oder Geweben durchgeführt werden. Es ist wichtig zu beachten, dass diese Produkte nicht als Arzneimittel oder Medikamente eingestuft sind und keine Zulassung der FDA für die Vorbeugung, Behandlung oder Heilung von medizinischen Zuständen, Beschwerden oder Krankheiten erhalten haben. Wir müssen betonen, dass jede Form der körperlichen Einführung dieser Produkte in Menschen oder Tiere gesetzlich strikt untersagt ist. Es ist unerlässlich, sich an diese Richtlinien zu halten, um die Einhaltung rechtlicher und ethischer Standards in Forschung und Experiment zu gewährleisten.