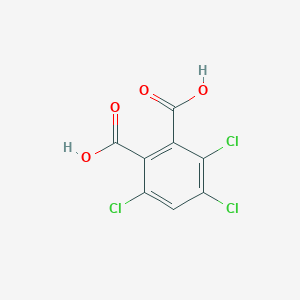
3,4,6-trichlorophthalic Acid
Übersicht
Beschreibung
3,4,6-Trichlorophthalic acid is an aromatic carboxylic acid derived from phthalic acid, characterized by the presence of three chlorine atoms at positions 3, 4, and 6 on the benzene ring
Vorbereitungsmethoden
Synthetic Routes and Reaction Conditions: 3,4,6-Trichlorophthalic acid can be synthesized through the selective hydrodechlorination of 3,4,5,6-tetrachlorophthalic anhydride. This process involves the use of zinc dust in aqueous sodium hydroxide (5 wt %) to achieve high yield and selectivity . The reaction conditions typically include heating the mixture to facilitate the hydrodechlorination process.
Industrial Production Methods: On an industrial scale, the preparation of this compound follows similar synthetic routes but is optimized for large-scale production. The use of continuous reactors and efficient separation techniques ensures high purity and yield of the final product.
Analyse Chemischer Reaktionen
Types of Reactions: 3,4,6-Trichlorophthalic acid undergoes various chemical reactions, including:
Reduction: The compound can be reduced to form less chlorinated derivatives.
Substitution: The chlorine atoms can be substituted with other functional groups through nucleophilic substitution reactions.
Esterification: The carboxylic acid groups can react with alcohols in the presence of acid catalysts to form esters.
Common Reagents and Conditions:
Reduction: Zinc dust and aqueous sodium hydroxide are commonly used for hydrodechlorination.
Substitution: Nucleophiles such as amines or thiols can be used under basic or acidic conditions.
Esterification: Alcohols and acid catalysts like sulfuric acid are used to form esters.
Major Products:
Reduction: Less chlorinated phthalic acids.
Substitution: Substituted phthalic acids with various functional groups.
Esterification: Esters of this compound.
Wissenschaftliche Forschungsanwendungen
3,4,6-Trichlorophthalic acid has diverse applications in scientific research:
Chemistry: It is used as an intermediate in the synthesis of various organic compounds.
Biology: The compound is studied for its potential biological activity, including antimicrobial and anti-tumor properties.
Medicine: Research explores its use in developing pharmaceuticals with specific biological activities.
Industry: It is used in the production of polymers, dyes, and other industrial chemicals.
Wirkmechanismus
The mechanism by which 3,4,6-trichlorophthalic acid exerts its effects involves interactions with molecular targets and pathways. The presence of chlorine atoms influences its reactivity and interactions with other molecules. The compound can act as an electrophile in substitution reactions, facilitating the formation of new chemical bonds.
Vergleich Mit ähnlichen Verbindungen
3,4,5-Trichlorophthalic acid: Similar in structure but with chlorine atoms at positions 3, 4, and 5.
3,4,5,6-Tetrachlorophthalic anhydride: The precursor used in the synthesis of 3,4,6-trichlorophthalic acid.
Uniqueness: this compound is unique due to the specific positioning of chlorine atoms, which affects its chemical properties and reactivity. This distinct structure allows for targeted applications in various fields, making it a valuable compound for research and industrial use.
Biologische Aktivität
TCPA's biological activity is primarily attributed to its ability to interact with various biochemical pathways. The presence of chlorine atoms enhances its lipophilicity, allowing it to penetrate cellular membranes more effectively. Its mode of action may involve:
- Inhibition of Enzymatic Activity : TCPA has shown potential to inhibit certain enzymes involved in metabolic pathways.
- Alteration of Gene Expression : Studies suggest that TCPA can modulate gene expression related to inflammation and cell proliferation.
Toxicity Studies
Toxicological evaluations have been critical in assessing the safety profile of TCPA. A notable study involved administering TCPA to F344/N rats and B6C3F1 mice via oral gavage for 13 weeks. Key findings from this study include:
Parameter | Observation |
---|---|
Survival Rate | No significant mortality in low-dose groups |
Body Weight Changes | Decreased body weight in high-dose groups |
Organ Weights | Increased kidney weights; no significant liver changes |
Histopathological Findings | Renal tubule necrosis observed at higher doses |
These findings indicate that while TCPA exhibits some toxic effects at high concentrations, it may not pose significant risks at lower doses.
Environmental Impact
TCPA's persistence in the environment has raised concerns regarding its ecological effects. Studies have indicated that TCPA can bioaccumulate in aquatic organisms, potentially disrupting endocrine functions and leading to adverse ecological outcomes.
Case Study 1: Antimicrobial Activity
Research has explored the antimicrobial properties of TCPA against various pathogens. In vitro studies demonstrated that TCPA exhibited significant antibacterial activity against Staphylococcus aureus and Escherichia coli, suggesting its potential use as an antimicrobial agent.
Case Study 2: Antitumor Effects
Another area of investigation focused on the antitumor effects of TCPA. In cell line studies, TCPA was found to induce apoptosis in cancer cells through the activation of caspase pathways. This suggests a potential role for TCPA in cancer therapy, although further studies are needed to elucidate its efficacy in vivo.
Research Findings
Recent research has highlighted several important aspects of TCPA's biological activity:
- Anti-inflammatory Properties : TCPA has been shown to reduce inflammatory markers in animal models, indicating potential therapeutic applications in treating inflammatory diseases.
- Neurotoxicity Studies : Investigations into neurotoxic effects revealed that TCPA could impair neuronal function at high concentrations, necessitating caution in its application.
Eigenschaften
IUPAC Name |
3,4,6-trichlorophthalic acid | |
---|---|---|
Source | PubChem | |
URL | https://pubchem.ncbi.nlm.nih.gov | |
Description | Data deposited in or computed by PubChem | |
InChI |
InChI=1S/C8H3Cl3O4/c9-2-1-3(10)6(11)5(8(14)15)4(2)7(12)13/h1H,(H,12,13)(H,14,15) | |
Source | PubChem | |
URL | https://pubchem.ncbi.nlm.nih.gov | |
Description | Data deposited in or computed by PubChem | |
InChI Key |
DCACTBQREATMLX-UHFFFAOYSA-N | |
Source | PubChem | |
URL | https://pubchem.ncbi.nlm.nih.gov | |
Description | Data deposited in or computed by PubChem | |
Canonical SMILES |
C1=C(C(=C(C(=C1Cl)Cl)C(=O)O)C(=O)O)Cl | |
Source | PubChem | |
URL | https://pubchem.ncbi.nlm.nih.gov | |
Description | Data deposited in or computed by PubChem | |
Molecular Formula |
C8H3Cl3O4 | |
Source | PubChem | |
URL | https://pubchem.ncbi.nlm.nih.gov | |
Description | Data deposited in or computed by PubChem | |
DSSTOX Substance ID |
DTXSID50455920 | |
Record name | 3,4,6-trichlorophthalic Acid | |
Source | EPA DSSTox | |
URL | https://comptox.epa.gov/dashboard/DTXSID50455920 | |
Description | DSSTox provides a high quality public chemistry resource for supporting improved predictive toxicology. | |
Molecular Weight |
269.5 g/mol | |
Source | PubChem | |
URL | https://pubchem.ncbi.nlm.nih.gov | |
Description | Data deposited in or computed by PubChem | |
CAS No. |
62268-16-0 | |
Record name | 3,4,6-trichlorophthalic Acid | |
Source | EPA DSSTox | |
URL | https://comptox.epa.gov/dashboard/DTXSID50455920 | |
Description | DSSTox provides a high quality public chemistry resource for supporting improved predictive toxicology. | |
Synthesis routes and methods I
Procedure details
Synthesis routes and methods II
Procedure details
Retrosynthesis Analysis
AI-Powered Synthesis Planning: Our tool employs the Template_relevance Pistachio, Template_relevance Bkms_metabolic, Template_relevance Pistachio_ringbreaker, Template_relevance Reaxys, Template_relevance Reaxys_biocatalysis model, leveraging a vast database of chemical reactions to predict feasible synthetic routes.
One-Step Synthesis Focus: Specifically designed for one-step synthesis, it provides concise and direct routes for your target compounds, streamlining the synthesis process.
Accurate Predictions: Utilizing the extensive PISTACHIO, BKMS_METABOLIC, PISTACHIO_RINGBREAKER, REAXYS, REAXYS_BIOCATALYSIS database, our tool offers high-accuracy predictions, reflecting the latest in chemical research and data.
Strategy Settings
Precursor scoring | Relevance Heuristic |
---|---|
Min. plausibility | 0.01 |
Model | Template_relevance |
Template Set | Pistachio/Bkms_metabolic/Pistachio_ringbreaker/Reaxys/Reaxys_biocatalysis |
Top-N result to add to graph | 6 |
Feasible Synthetic Routes
Q1: How is 3,4,6-trichlorophthalic acid synthesized?
A1: this compound is synthesized via the selective hydrodechlorination of 3,4,5,6-tetrachlorophthalic anhydride. This reaction utilizes zinc dust and aqueous sodium hydroxide. [, ] You can find more details about the reaction conditions in the cited research papers.
Q2: What are the further applications of this compound in synthesis?
A2: this compound serves as a crucial precursor to other substituted phthalic acid derivatives. Further reaction with zinc dust and aqueous sodium hydroxide leads to the formation of 3,6-dichlorophthalic acid and 3-chlorophthalic anhydride. [] Furthermore, it can be converted to 3,6-dichlorophthalic anhydride, a precursor to 4,7-diaminoisoindoline-1,3-dione (also known as 3,6-diaminophthalimide). []
Haftungsausschluss und Informationen zu In-Vitro-Forschungsprodukten
Bitte beachten Sie, dass alle Artikel und Produktinformationen, die auf BenchChem präsentiert werden, ausschließlich zu Informationszwecken bestimmt sind. Die auf BenchChem zum Kauf angebotenen Produkte sind speziell für In-vitro-Studien konzipiert, die außerhalb lebender Organismen durchgeführt werden. In-vitro-Studien, abgeleitet von dem lateinischen Begriff "in Glas", beinhalten Experimente, die in kontrollierten Laborumgebungen unter Verwendung von Zellen oder Geweben durchgeführt werden. Es ist wichtig zu beachten, dass diese Produkte nicht als Arzneimittel oder Medikamente eingestuft sind und keine Zulassung der FDA für die Vorbeugung, Behandlung oder Heilung von medizinischen Zuständen, Beschwerden oder Krankheiten erhalten haben. Wir müssen betonen, dass jede Form der körperlichen Einführung dieser Produkte in Menschen oder Tiere gesetzlich strikt untersagt ist. Es ist unerlässlich, sich an diese Richtlinien zu halten, um die Einhaltung rechtlicher und ethischer Standards in Forschung und Experiment zu gewährleisten.