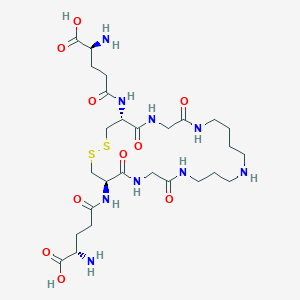
Trypanothione
Übersicht
Beschreibung
Trypanothione [N¹,N⁸-bis(glutathionyl)spermidine, T(SH)₂] is a unique low-molecular-weight thiol found exclusively in kinetoplastid parasites, including Trypanosoma and Leishmania species. It serves as the central redox buffer in these organisms, replacing the glutathione/glutathione reductase (GR) system present in mammals and other eukaryotes . Biosynthesis of this compound involves the ATP-dependent conjugation of two glutathione molecules to spermidine, catalyzed by this compound synthetase (TryS) . This compound reductase (TR), a flavoenzyme homologous to GR, regenerates reduced this compound from its oxidized disulfide form (TS₂), enabling the parasite to neutralize reactive oxygen species (ROS), maintain thiol homeostasis, and resist oxidative stress induced by host immune responses .
The absence of this compound metabolism in mammals and its essentiality for parasite survival make TR and TryS prime targets for antiparasitic drug development .
Vorbereitungsmethoden
Synthesewege und Reaktionsbedingungen
Die Synthese von Trypanothion beinhaltet die Konjugation von zwei Glutathionmolekülen mit Spermidin. Der Prozess umfasst typischerweise die folgenden Schritte:
Aktivierung von Glutathion: Glutathion wird unter Verwendung eines Kupplungsreagenzes wie Dicyclohexylcarbodiimid (DCC) aktiviert.
Konjugation mit Spermidin: Das aktivierte Glutathion wird dann unter kontrollierten pH-Bedingungen mit Spermidin umgesetzt, um Trypanothion zu bilden.
Industrielle Produktionsverfahren
Die industrielle Produktion von Trypanothion ist aufgrund seiner spezifischen biologischen Rolle und der Komplexität seiner Synthese nicht üblich. Die Synthesemethoden im Labor können mit einer geeigneten Optimierung der Reaktionsbedingungen und Reinigungsprozesse hochskaliert werden.
Analyse Chemischer Reaktionen
Arten von Reaktionen
Oxidation: Trypanothion wird oxidiert, um Trypanothiondisulfid zu bilden.
Reduktion: Die Disulfidform kann durch Trypanothionreduktase wieder in die Thiolform reduziert werden.
Substitution: Es kann an Substitutionsreaktionen teilnehmen, bei denen die Thiolgruppen beteiligt sind.
Häufige Reagenzien und Bedingungen
Oxidation: Wasserstoffperoxid oder andere Peroxide unter milden Bedingungen.
Reduktion: NADPH in Gegenwart von Trypanothionreduktase.
Substitution: Verschiedene Elektrophile können unter neutralen bis leicht basischen Bedingungen mit den Thiolgruppen reagieren.
Hauptprodukte
Oxidation: Trypanothiondisulfid.
Reduktion: Reduziertes Trypanothion.
Substitution: Thiol-substituierte Derivate, abhängig vom verwendeten Elektrophil.
Wissenschaftliche Forschungsanwendungen
Target for Antiparasitic Drug Development
Trypanothione synthetase (TryS), the enzyme responsible for synthesizing this compound, has been identified as a potential drug target due to its essentiality for parasite survival. High-throughput screening of chemical libraries has led to the identification of several inhibitors that effectively decrease intracellular this compound levels in T. brucei, demonstrating their potential as therapeutic agents against trypanosomiasis .
Inhibitors and Synergistic Drug Combinations
Research has shown that existing FDA-approved drugs can be repurposed to target this compound-related pathways. For instance, combinations of antihistamines and antifungal agents have exhibited synergistic effects against Trypanosoma cruzi, the causative agent of Chagas disease. These combinations have demonstrated significant efficacy in reducing parasitemia in murine models, highlighting the potential for developing new treatment regimens based on this compound modulation .
Mechanism of Action Studies
Studies investigating the mechanism of action of various compounds on this compound reductase (the enzyme that recycles this compound) have provided insights into how these drugs can disrupt the redox balance within parasites. For example, epigallocatechin gallate (EGCG) has been shown to inhibit this enzyme in Leishmania promastigotes, leading to increased oxidative stress and parasite death .
This compound Synthetase Inhibitors
A notable study involved the screening of over 63,000 compounds to identify inhibitors of TryS. The lead compound DDD86243 was found to significantly inhibit TryS activity, resulting in a marked decrease in this compound levels and impaired growth of T. brucei in vitro . This study exemplifies how targeting this compound metabolism can lead to effective antiparasitic strategies.
Synergistic Effects in Drug Combinations
In another case, researchers identified combinations of drugs that showed enhanced efficacy against T. cruzi. For instance, the combination of clemastine (an antihistamine) and posaconazole (an antifungal) was more effective than either drug alone in reducing parasitemia in infected mice. This finding underscores the importance of exploring drug interactions that target the this compound pathway .
Resistance Mechanisms
Understanding how parasites develop resistance to therapies targeting this compound is critical. Research indicates that while some parasites can adapt by altering their thiol metabolism, targeting unique pathways associated with this compound may mitigate resistance issues commonly seen with other antiparasitic drugs .
Summary Table: Applications and Findings
Wirkmechanismus
Trypanothione exerts its effects primarily through its role in redox reactions. It acts as an electron donor in the reduction of peroxides and other reactive oxygen species. The key molecular targets include:
This compound Reductase: Reduces this compound disulfide back to its thiol form using NADPH.
Tryparedoxin Peroxidase: Reduces peroxides using electrons from this compound, either directly or via tryparedoxin.
Vergleich Mit ähnlichen Verbindungen
Trypanothione shares functional and structural similarities with other thiol-based redox systems but exhibits distinct biochemical properties. Below is a detailed comparison:
Glutathione (GSH)
- Structure: Glutathione (γ-L-glutamyl-L-cysteinylglycine) is a tripeptide, whereas this compound is a spermidine-glutathione conjugate.
- Function: Both act as antioxidants, but GSH is regenerated by glutathione reductase (GR) in mammals, while this compound is reduced by TR in parasites .
- Enzyme Specificity: TR cannot reduce GSH disulfide (GSSG), and GR cannot reduce TS₂, highlighting evolutionary divergence in substrate recognition .
Homothis compound
- Structure: Homothis compound replaces spermidine with homospermidine in the conjugate.
- Activity: It is a substrate for TR but is less abundant in parasites. T. cruzi TR reduces homothis compound disulfide with ~60% efficiency compared to TS₂ .
Bis(glutathionyl)spermine
- Structure : A spermine-glutathione conjugate analog.
- Substrate Activity : Bis(glutathionyl)spermine disulfide is efficiently reduced by TR, with kinetic parameters (kcat/Km) comparable to TS₂, suggesting broad substrate flexibility in TR .
Thioredoxin (Trx) System
- Function: The Trx system (Trx/Trx reductase/NADPH) overlaps with this compound in redox regulation but is mechanistically distinct.
- Structural Differences : TR shares a similar fold with GR and Trx reductase but has a divergent C-terminal domain that prevents NADPH binding in a manner analogous to GR .
Table 1: Comparative Biochemical Properties of Thiol Systems
Table 2: Inhibitor Binding Affinities for this compound Reductase
*Estimated values from docking studies.
Key Differences in Drug Targeting
- Species Specificity: this compound synthetase (TryS) inhibitors show species-specific efficacy. For example, T. brucei TryS is inhibited by N,N'-bis(benzyl)diamines (IC₅₀ = 12 µM), while L. infantum TryS is more sensitive to paullone derivatives (IC₅₀ = 8 µM) .
- Structural Determinants : Mutagenesis studies reveal that TR’s catalytic cysteine residues (Cys52/Cys57 in T. congolense) are critical for activity, analogous to GR but distinct from TrxR .
Research Findings and Implications
Inhibitor Design: Aryl-substituted imidazoles and quinoxaline derivatives mimic TS₂ binding, achieving nM-level Ki values .
Natural Products : Theaflavin and camptothecin dock into TR’s active site with moderate affinity, offering scaffolds for antiparasitic drug development .
Subversive Substrates : Bis(glutathionyl)spermine acts as a "turncoat" inhibitor, competing with TS₂ while being metabolized by TR .
Biologische Aktivität
Introduction
Trypanothione, a unique thiol compound found predominantly in trypanosomatids, plays a crucial role in the redox metabolism of these parasites. Its biological activity is primarily mediated through its interaction with various enzymes, particularly this compound reductase (TR), which is essential for maintaining cellular redox homeostasis. This article delves into the biological activity of this compound, highlighting its mechanisms, therapeutic potential, and recent research findings.
Structure and Function
This compound is chemically defined as N1,N8-bis(glutathionyl)spermidine (T(SH)₂). It consists of two glutathione molecules linked to a spermidine backbone. This structure allows it to participate in various biochemical processes, including detoxification of xenobiotics, reduction of oxidized proteins, and maintenance of thiol-redox homeostasis.
Key Functions of this compound
- Redox Regulation : Acts as a reducing agent in cellular environments, counteracting oxidative stress.
- Detoxification : Involved in the neutralization of harmful compounds such as methylglyoxal and nitric oxide.
- Antioxidant Defense : Protects cellular components from oxidative damage.
This compound Reductase (TR)
TR is a flavoenzyme that catalyzes the reduction of oxidized this compound (T(S)₂) back to its reduced form (T(SH)₂) using NADPH as an electron donor. This enzyme is critical for the survival of trypanosomatids, making it an attractive target for drug development.
Inhibition Studies
Recent studies have identified several inhibitors of TR that show promise as therapeutic agents against diseases caused by Trypanosoma species. For instance:
- High-Throughput Screening : A screening campaign identified nine novel classes of TR inhibitors that demonstrated significant antiparasitic activity against Trypanosoma brucei .
- Chemical Validation : Inhibitors targeting this compound synthetase (TryS), another enzyme involved in this compound biosynthesis, have shown potent effects on parasite growth .
Case Study 1: Antiparasitic Activity
A study reported the identification of substituted 2-(3-aminophenyl) oxazolopyridines that exhibited an EC50 of 2 nM against Trypanosoma brucei. These compounds were orally bioavailable and effectively cured infected mice at low doses .
Case Study 2: TR Inhibitors in T. cruzi Infection
Research demonstrated that TR inhibitors significantly reduced parasitemia and mortality in animal models infected with Trypanosoma cruzi. However, the exact mechanism by which these compounds exert their antiparasitic effects remains to be fully elucidated .
Table 1: Summary of TR Inhibitors and Their Biological Activities
Compound Class | Target Enzyme | EC50 (nM) | Effectiveness |
---|---|---|---|
Substituted Oxazolopyridines | This compound Reductase | 2 | Cured mice infected with T. brucei |
Urea Derivatives | This compound Synthetase | Varies | Inhibited growth in vitro |
Novel Chemical Scaffolds | This compound Reductase | Varies | Reduced parasitemia in vivo |
The biological activity of this compound can be attributed to several mechanisms:
- Electron Transfer : TR facilitates electron transfer from NADPH to T(S)₂, maintaining the reduced state necessary for cellular functions.
- Oxidative Stress Response : By reducing reactive oxygen species (ROS), this compound protects cells from oxidative damage.
- Protein Thiol Homeostasis : It plays a role in maintaining protein thiol groups in their reduced forms, which is vital for protein function.
Q & A
Basic Research Questions
Q. What experimental methods are most reliable for quantifying intracellular trypanothione levels in Trypanosoma parasites?
- Methodological Answer : Use high-performance liquid chromatography (HPLC) coupled with fluorescence detection or liquid chromatography-mass spectrometry (LC-MS) to measure this compound concentrations. Ensure protocols account for rapid thiol oxidation by including stabilizing agents like N-ethylmaleimide . For live-cell imaging, genetically encoded redox biosensors (e.g., Grx1-roGFP2) can monitor real-time this compound dynamics in parasites .
Q. How do this compound-dependent enzymes (e.g., this compound reductase, TryR) differ functionally from their glutathione-dependent counterparts in mammals?
- Methodological Answer : Conduct comparative enzyme kinetics using recombinant TryR and human glutathione reductase. Measure substrate specificity (e.g., NADPH vs. NADH dependence), inhibitor sensitivity (e.g., phenothiazines), and redox potential via spectrophotometric assays under anaerobic conditions . Structural studies (X-ray crystallography) can highlight active-site variations influencing drug selectivity .
Q. What genetic tools are available to study this compound biosynthesis in Leishmania species?
- Methodological Answer : Use RNA interference (RNAi) or CRISPR-Cas9 to knock down/out genes in the this compound pathway (e.g., TryS, TryR). Validate via qRT-PCR and enzymatic activity assays. Phenotypic screening under oxidative stress (e.g., H₂O₂ exposure) can assess pathway essentiality .
Advanced Research Questions
Q. How can molecular dynamics (MD) simulations address structural gaps in this compound synthetase (TryS) for inhibitor design?
- Methodological Answer : Perform MD simulations on TryS crystal structures to model missing flexible loops critical for substrate binding. Use trajectory analysis to identify conformational states for docking studies. Validate predictions with mutagenesis and enzymatic inhibition assays . Reference public MD datasets (e.g., GPCRdb) for reproducibility .
Q. What strategies reconcile contradictory data on this compound reductase (TryR) inhibitor efficacy across parasite strains?
- Methodological Answer : Standardize assays using identical conditions (pH, temperature, cofactor concentrations). Test inhibitors against TryR isoforms from diverse strains (e.g., T. cruzi vs. T. brucei). Combine enzyme inhibition data with in vivo efficacy studies in infected macrophages to assess parasite-specific resistance mechanisms .
Q. How do redox biosensors differentiate this compound from other low-molecular-weight thiols in parasite-host interactions?
- Methodological Answer : Engineer biosensors with specificity for this compound by fusing Trypanosoma-specific glutaredoxin domains to redox-sensitive fluorescent proteins. Validate specificity via knockout parasites lacking this compound. Apply confocal microscopy to map subcellular redox changes during host-cell invasion .
Q. Data Contradiction & Validation
Q. Why do some studies report this compound-independent antioxidant mechanisms in Trypanosoma under oxidative stress?
- Methodological Answer : Investigate compensatory pathways (e.g., thioredoxin or ascorbate systems) using dual RNA-seq/proteomics in this compound-deficient mutants. Use ROS-specific dyes (e.g., CellROX) to quantify residual antioxidant capacity. Compare findings across life-cycle stages (e.g., insect vs. mammalian stages) .
Q. How can conflicting results on this compound reductase (TryR) as a drug target be resolved?
- Methodological Answer : Apply orthogonal validation methods:
- In vitro: High-throughput screening with recombinant TryR and counter-screening against human homologs.
- In vivo: Use conditional knockout parasites to assess target vulnerability in infection models.
- Clinical correlation: Cross-reference inhibitor efficacy with patient-derived parasite strains .
Q. Experimental Design & Optimization
Q. What controls are essential when screening this compound pathway inhibitors in in vitro assays?
- Methodological Answer : Include:
- Positive controls (e.g., ethidium bromide for T. brucei growth inhibition).
- Negative controls (e.g., untreated parasites and solvent-only wells).
- Selectivity controls (e.g., human cell lines to assess toxicity).
- Redox controls (e.g., diamide or BSO to induce oxidative stress) .
Q. How can machine learning improve virtual screening for this compound synthetase (TryS) inhibitors?
- Methodological Answer : Train models on curated datasets of known TryS inhibitors (e.g., from ChEMBL) using features like molecular descriptors, docking scores, and MD-derived binding energies. Validate predictions with enzymatic assays and in vitro parasite viability tests. Use SHAP analysis to interpret model decisions .
Eigenschaften
IUPAC Name |
(2S)-2-amino-5-[[(4R,23R)-4-[[(4S)-4-amino-4-carboxybutanoyl]amino]-5,8,19,22-tetraoxo-1,2-dithia-6,9,13,18,21-pentazacyclotetracos-23-yl]amino]-5-oxopentanoic acid | |
---|---|---|
Source | PubChem | |
URL | https://pubchem.ncbi.nlm.nih.gov | |
Description | Data deposited in or computed by PubChem | |
InChI |
InChI=1S/C27H47N9O10S2/c28-16(26(43)44)4-6-20(37)35-18-14-47-48-15-19(36-21(38)7-5-17(29)27(45)46)25(42)34-13-23(40)32-11-3-9-30-8-1-2-10-31-22(39)12-33-24(18)41/h16-19,30H,1-15,28-29H2,(H,31,39)(H,32,40)(H,33,41)(H,34,42)(H,35,37)(H,36,38)(H,43,44)(H,45,46)/t16-,17-,18-,19-/m0/s1 | |
Source | PubChem | |
URL | https://pubchem.ncbi.nlm.nih.gov | |
Description | Data deposited in or computed by PubChem | |
InChI Key |
LZMSXDHGHZKXJD-VJANTYMQSA-N | |
Source | PubChem | |
URL | https://pubchem.ncbi.nlm.nih.gov | |
Description | Data deposited in or computed by PubChem | |
Canonical SMILES |
C1CCNC(=O)CNC(=O)C(CSSCC(C(=O)NCC(=O)NCCCNC1)NC(=O)CCC(C(=O)O)N)NC(=O)CCC(C(=O)O)N | |
Source | PubChem | |
URL | https://pubchem.ncbi.nlm.nih.gov | |
Description | Data deposited in or computed by PubChem | |
Isomeric SMILES |
C1CCNC(=O)CNC(=O)[C@H](CSSC[C@@H](C(=O)NCC(=O)NCCCNC1)NC(=O)CC[C@@H](C(=O)O)N)NC(=O)CC[C@@H](C(=O)O)N | |
Source | PubChem | |
URL | https://pubchem.ncbi.nlm.nih.gov | |
Description | Data deposited in or computed by PubChem | |
Molecular Formula |
C27H47N9O10S2 | |
Source | PubChem | |
URL | https://pubchem.ncbi.nlm.nih.gov | |
Description | Data deposited in or computed by PubChem | |
DSSTOX Substance ID |
DTXSID70242197 | |
Record name | Trypanothione | |
Source | EPA DSSTox | |
URL | https://comptox.epa.gov/dashboard/DTXSID70242197 | |
Description | DSSTox provides a high quality public chemistry resource for supporting improved predictive toxicology. | |
Molecular Weight |
721.9 g/mol | |
Source | PubChem | |
URL | https://pubchem.ncbi.nlm.nih.gov | |
Description | Data deposited in or computed by PubChem | |
CAS No. |
96304-42-6 | |
Record name | Trypanothione | |
Source | CAS Common Chemistry | |
URL | https://commonchemistry.cas.org/detail?cas_rn=96304-42-6 | |
Description | CAS Common Chemistry is an open community resource for accessing chemical information. Nearly 500,000 chemical substances from CAS REGISTRY cover areas of community interest, including common and frequently regulated chemicals, and those relevant to high school and undergraduate chemistry classes. This chemical information, curated by our expert scientists, is provided in alignment with our mission as a division of the American Chemical Society. | |
Explanation | The data from CAS Common Chemistry is provided under a CC-BY-NC 4.0 license, unless otherwise stated. | |
Record name | Trypanothione | |
Source | ChemIDplus | |
URL | https://pubchem.ncbi.nlm.nih.gov/substance/?source=chemidplus&sourceid=0096304426 | |
Description | ChemIDplus is a free, web search system that provides access to the structure and nomenclature authority files used for the identification of chemical substances cited in National Library of Medicine (NLM) databases, including the TOXNET system. | |
Record name | Trypanothione | |
Source | EPA DSSTox | |
URL | https://comptox.epa.gov/dashboard/DTXSID70242197 | |
Description | DSSTox provides a high quality public chemistry resource for supporting improved predictive toxicology. | |
Retrosynthesis Analysis
AI-Powered Synthesis Planning: Our tool employs the Template_relevance Pistachio, Template_relevance Bkms_metabolic, Template_relevance Pistachio_ringbreaker, Template_relevance Reaxys, Template_relevance Reaxys_biocatalysis model, leveraging a vast database of chemical reactions to predict feasible synthetic routes.
One-Step Synthesis Focus: Specifically designed for one-step synthesis, it provides concise and direct routes for your target compounds, streamlining the synthesis process.
Accurate Predictions: Utilizing the extensive PISTACHIO, BKMS_METABOLIC, PISTACHIO_RINGBREAKER, REAXYS, REAXYS_BIOCATALYSIS database, our tool offers high-accuracy predictions, reflecting the latest in chemical research and data.
Strategy Settings
Precursor scoring | Relevance Heuristic |
---|---|
Min. plausibility | 0.01 |
Model | Template_relevance |
Template Set | Pistachio/Bkms_metabolic/Pistachio_ringbreaker/Reaxys/Reaxys_biocatalysis |
Top-N result to add to graph | 6 |
Feasible Synthetic Routes
Haftungsausschluss und Informationen zu In-Vitro-Forschungsprodukten
Bitte beachten Sie, dass alle Artikel und Produktinformationen, die auf BenchChem präsentiert werden, ausschließlich zu Informationszwecken bestimmt sind. Die auf BenchChem zum Kauf angebotenen Produkte sind speziell für In-vitro-Studien konzipiert, die außerhalb lebender Organismen durchgeführt werden. In-vitro-Studien, abgeleitet von dem lateinischen Begriff "in Glas", beinhalten Experimente, die in kontrollierten Laborumgebungen unter Verwendung von Zellen oder Geweben durchgeführt werden. Es ist wichtig zu beachten, dass diese Produkte nicht als Arzneimittel oder Medikamente eingestuft sind und keine Zulassung der FDA für die Vorbeugung, Behandlung oder Heilung von medizinischen Zuständen, Beschwerden oder Krankheiten erhalten haben. Wir müssen betonen, dass jede Form der körperlichen Einführung dieser Produkte in Menschen oder Tiere gesetzlich strikt untersagt ist. Es ist unerlässlich, sich an diese Richtlinien zu halten, um die Einhaltung rechtlicher und ethischer Standards in Forschung und Experiment zu gewährleisten.