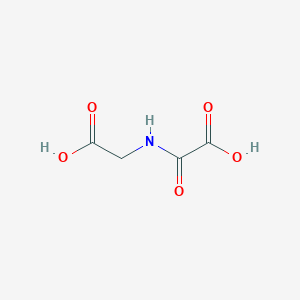
N-Oxalylglycin
Übersicht
Beschreibung
N-Oxalylglycine is an organic compound with the chemical formula HO₂CC(O)NHCH₂CO₂H. This colorless solid is primarily known for its role as an inhibitor of α-ketoglutarate-dependent enzymes . It is isosteric with α-Ketoglutaric acid, meaning it shares a similar structure but differs in certain properties .
Wissenschaftliche Forschungsanwendungen
N-Oxalylglycin hat ein breites Anwendungsspektrum in der wissenschaftlichen Forschung:
Medizin: Es wird in Studien im Zusammenhang mit der Hypoxie-Antwort und der Chromatin-Modifikation eingesetzt.
5. Wirkmechanismus
This compound übt seine Wirkungen aus, indem es α-Ketoglutarat-abhängige Enzyme hemmt. Es imitiert die ersten Schritte des Hydroxylierungsprozesses, initiiert aber die Hydroxylierung selbst nicht . Diese Hemmung betrifft verschiedene molekulare Ziele und Signalwege, darunter den Hypoxie-induzierbaren Faktor-Signalweg und Chromatin-Modifikationsprozesse .
Wirkmechanismus
Target of Action
N-Oxalylglycine (NOG) is primarily used as an inhibitor of α-ketoglutarate-dependent enzymes . These enzymes are pervasive and play a crucial role in various biological processes, including the synthesis of 4-hydroxyproline . NOG has also been extensively used as a tool to study prolyl hydroxylases (PHDs) .
Mode of Action
It interacts with its targets by inhibiting their function, thereby affecting the downstream processes that these enzymes are involved in .
Biochemical Pathways
The inhibition of α-ketoglutarate-dependent enzymes by NOG affects several biochemical pathways. For instance, it impacts the synthesis of 4-hydroxyproline . Furthermore, NOG can be used in studies on the hypoxic response and chromatin modifications in animals .
Result of Action
The primary result of NOG’s action is the inhibition of α-ketoglutarate-dependent enzymes, which leads to changes in the processes these enzymes are involved in . For example, it can affect the synthesis of 4-hydroxyproline .
Biochemische Analyse
Biochemical Properties
N-Oxalylglycine functions primarily as an inhibitor of α-ketoglutarate-dependent enzymes. These enzymes are involved in numerous biochemical processes, including the synthesis of 4-hydroxyproline . N-Oxalylglycine interacts with enzymes such as Jumonji C-domain-containing histone lysine demethylases, inhibiting their activity . This inhibition is crucial for studying the role of these enzymes in cellular processes and disease mechanisms.
Cellular Effects
N-Oxalylglycine has profound effects on various cell types and cellular processes. It influences cell function by inhibiting α-ketoglutarate-dependent enzymes, which are essential for cell signaling pathways, gene expression, and cellular metabolism . By inhibiting these enzymes, N-Oxalylglycine can alter the expression of genes involved in critical cellular functions, leading to changes in cell behavior and metabolism.
Molecular Mechanism
The molecular mechanism of N-Oxalylglycine involves its binding to the active site of α-ketoglutarate-dependent enzymes, preventing the binding of α-ketoglutarate . This competitive inhibition blocks the enzyme’s activity, leading to downstream effects on cellular processes. N-Oxalylglycine’s ability to inhibit these enzymes makes it a valuable tool for studying their roles in various biological pathways.
Temporal Effects in Laboratory Settings
In laboratory settings, the effects of N-Oxalylglycine can change over time. The compound’s stability and degradation are critical factors that influence its long-term effects on cellular function. Studies have shown that N-Oxalylglycine remains stable under specific conditions, allowing for prolonged inhibition of target enzymes . Its degradation over time can lead to a decrease in its inhibitory effects, necessitating careful monitoring in experimental setups.
Dosage Effects in Animal Models
The effects of N-Oxalylglycine vary with different dosages in animal models. At lower doses, N-Oxalylglycine effectively inhibits α-ketoglutarate-dependent enzymes without causing significant adverse effects . At higher doses, toxic effects may be observed, including disruptions in cellular metabolism and potential damage to tissues. These dosage-dependent effects highlight the importance of optimizing N-Oxalylglycine concentrations in experimental studies.
Metabolic Pathways
N-Oxalylglycine is involved in metabolic pathways that require α-ketoglutarate-dependent enzymes. By inhibiting these enzymes, N-Oxalylglycine can affect metabolic flux and metabolite levels . This inhibition can lead to alterations in the synthesis of critical metabolites, impacting various biochemical pathways and cellular functions.
Transport and Distribution
Within cells and tissues, N-Oxalylglycine is transported and distributed through interactions with specific transporters and binding proteins . These interactions influence its localization and accumulation, affecting its inhibitory activity. Understanding the transport and distribution of N-Oxalylglycine is essential for optimizing its use in experimental and therapeutic applications.
Subcellular Localization
N-Oxalylglycine’s subcellular localization is determined by targeting signals and post-translational modifications that direct it to specific compartments or organelles . This localization is crucial for its activity and function, as it ensures that N-Oxalylglycine reaches its target enzymes within the cell. By understanding its subcellular localization, researchers can better predict and control its effects on cellular processes.
Vorbereitungsmethoden
N-Oxalylglycin kann durch die Reaktion von Glycin mit Oxalsäure synthetisiert werden. Die Synthese umfasst die folgenden Schritte :
Reaktion von Glycin mit Oxalsäure: Glycin reagiert mit Oxalsäure in Gegenwart eines Dehydratisierungsmittels zu this compound.
Reinigung: Das Rohprodukt wird durch Umkristallisation oder Chromatographie gereinigt, um reines this compound zu erhalten.
Industrielle Produktionsverfahren können ähnliche Schritte umfassen, jedoch in größerem Maßstab und mit optimierten Reaktionsbedingungen, um hohe Ausbeute und Reinheit zu gewährleisten.
Analyse Chemischer Reaktionen
N-Oxalylglycin durchläuft verschiedene chemische Reaktionen, darunter:
Oxidation: Es kann zu Oxalsäure und Glycin oxidiert werden.
Reduktion: Reduktionsreaktionen können es in seine Vorläuferverbindungen zurückverwandeln.
Substitution: Es kann Substitutionsreaktionen eingehen, bei denen die Oxalylgruppe durch andere funktionelle Gruppen ersetzt wird.
Häufige Reagenzien und Bedingungen, die in diesen Reaktionen verwendet werden, umfassen Oxidationsmittel wie Wasserstoffperoxid, Reduktionsmittel wie Natriumborhydrid und verschiedene Katalysatoren, um Substitutionsreaktionen zu erleichtern. Die Hauptprodukte, die aus diesen Reaktionen entstehen, sind Oxalsäure, Glycin und substituierte Derivate von this compound .
Vergleich Mit ähnlichen Verbindungen
N-Oxalylglycin ist aufgrund seiner spezifischen Hemmung von α-Ketoglutarat-abhängigen Enzymen einzigartig. Zu ähnlichen Verbindungen gehören:
- N-Acetylglycinamid
- Glycylglycin
- Oxalyldiaminopropionsäure
Diese Verbindungen weisen strukturelle Ähnlichkeiten auf, unterscheiden sich aber in ihren spezifischen biologischen Aktivitäten und Anwendungen .
Eigenschaften
IUPAC Name |
2-(carboxymethylamino)-2-oxoacetic acid | |
---|---|---|
Source | PubChem | |
URL | https://pubchem.ncbi.nlm.nih.gov | |
Description | Data deposited in or computed by PubChem | |
InChI |
InChI=1S/C4H5NO5/c6-2(7)1-5-3(8)4(9)10/h1H2,(H,5,8)(H,6,7)(H,9,10) | |
Source | PubChem | |
URL | https://pubchem.ncbi.nlm.nih.gov | |
Description | Data deposited in or computed by PubChem | |
InChI Key |
BIMZLRFONYSTPT-UHFFFAOYSA-N | |
Source | PubChem | |
URL | https://pubchem.ncbi.nlm.nih.gov | |
Description | Data deposited in or computed by PubChem | |
Canonical SMILES |
C(C(=O)O)NC(=O)C(=O)O | |
Source | PubChem | |
URL | https://pubchem.ncbi.nlm.nih.gov | |
Description | Data deposited in or computed by PubChem | |
Molecular Formula |
C4H5NO5 | |
Source | PubChem | |
URL | https://pubchem.ncbi.nlm.nih.gov | |
Description | Data deposited in or computed by PubChem | |
DSSTOX Substance ID |
DTXSID20200601 | |
Record name | Oxalylglycine | |
Source | EPA DSSTox | |
URL | https://comptox.epa.gov/dashboard/DTXSID20200601 | |
Description | DSSTox provides a high quality public chemistry resource for supporting improved predictive toxicology. | |
Molecular Weight |
147.09 g/mol | |
Source | PubChem | |
URL | https://pubchem.ncbi.nlm.nih.gov | |
Description | Data deposited in or computed by PubChem | |
CAS No. |
5262-39-5 | |
Record name | N-Oxalylglycine | |
Source | CAS Common Chemistry | |
URL | https://commonchemistry.cas.org/detail?cas_rn=5262-39-5 | |
Description | CAS Common Chemistry is an open community resource for accessing chemical information. Nearly 500,000 chemical substances from CAS REGISTRY cover areas of community interest, including common and frequently regulated chemicals, and those relevant to high school and undergraduate chemistry classes. This chemical information, curated by our expert scientists, is provided in alignment with our mission as a division of the American Chemical Society. | |
Explanation | The data from CAS Common Chemistry is provided under a CC-BY-NC 4.0 license, unless otherwise stated. | |
Record name | Oxalylglycine | |
Source | ChemIDplus | |
URL | https://pubchem.ncbi.nlm.nih.gov/substance/?source=chemidplus&sourceid=0005262395 | |
Description | ChemIDplus is a free, web search system that provides access to the structure and nomenclature authority files used for the identification of chemical substances cited in National Library of Medicine (NLM) databases, including the TOXNET system. | |
Record name | Oxalylglycine | |
Source | EPA DSSTox | |
URL | https://comptox.epa.gov/dashboard/DTXSID20200601 | |
Description | DSSTox provides a high quality public chemistry resource for supporting improved predictive toxicology. | |
Record name | N-OXALYLGLYCINE | |
Source | European Chemicals Agency (ECHA) | |
URL | https://echa.europa.eu/information-on-chemicals | |
Description | The European Chemicals Agency (ECHA) is an agency of the European Union which is the driving force among regulatory authorities in implementing the EU's groundbreaking chemicals legislation for the benefit of human health and the environment as well as for innovation and competitiveness. | |
Explanation | Use of the information, documents and data from the ECHA website is subject to the terms and conditions of this Legal Notice, and subject to other binding limitations provided for under applicable law, the information, documents and data made available on the ECHA website may be reproduced, distributed and/or used, totally or in part, for non-commercial purposes provided that ECHA is acknowledged as the source: "Source: European Chemicals Agency, http://echa.europa.eu/". Such acknowledgement must be included in each copy of the material. ECHA permits and encourages organisations and individuals to create links to the ECHA website under the following cumulative conditions: Links can only be made to webpages that provide a link to the Legal Notice page. | |
Record name | N-(CARBOXYCARBONYL)GLYCINE | |
Source | FDA Global Substance Registration System (GSRS) | |
URL | https://gsrs.ncats.nih.gov/ginas/app/beta/substances/VVW38EB8YS | |
Description | The FDA Global Substance Registration System (GSRS) enables the efficient and accurate exchange of information on what substances are in regulated products. Instead of relying on names, which vary across regulatory domains, countries, and regions, the GSRS knowledge base makes it possible for substances to be defined by standardized, scientific descriptions. | |
Explanation | Unless otherwise noted, the contents of the FDA website (www.fda.gov), both text and graphics, are not copyrighted. They are in the public domain and may be republished, reprinted and otherwise used freely by anyone without the need to obtain permission from FDA. Credit to the U.S. Food and Drug Administration as the source is appreciated but not required. | |
Retrosynthesis Analysis
AI-Powered Synthesis Planning: Our tool employs the Template_relevance Pistachio, Template_relevance Bkms_metabolic, Template_relevance Pistachio_ringbreaker, Template_relevance Reaxys, Template_relevance Reaxys_biocatalysis model, leveraging a vast database of chemical reactions to predict feasible synthetic routes.
One-Step Synthesis Focus: Specifically designed for one-step synthesis, it provides concise and direct routes for your target compounds, streamlining the synthesis process.
Accurate Predictions: Utilizing the extensive PISTACHIO, BKMS_METABOLIC, PISTACHIO_RINGBREAKER, REAXYS, REAXYS_BIOCATALYSIS database, our tool offers high-accuracy predictions, reflecting the latest in chemical research and data.
Strategy Settings
Precursor scoring | Relevance Heuristic |
---|---|
Min. plausibility | 0.01 |
Model | Template_relevance |
Template Set | Pistachio/Bkms_metabolic/Pistachio_ringbreaker/Reaxys/Reaxys_biocatalysis |
Top-N result to add to graph | 6 |
Feasible Synthetic Routes
A: NOG acts as a competitive inhibitor of 2OG oxygenases by mimicking the 2OG co-substrate and binding to the enzyme's active site. [, , ] This disrupts the enzyme's catalytic cycle, preventing the binding and subsequent oxidation of the intended substrate. [, , ]
A: The downstream effects of NOG treatment are diverse and depend on the specific 2OG oxygenase targeted. [, , , ] For instance, NOG can:
* Stabilize hypoxia-inducible factors (HIFs) by inhibiting HIF prolyl hydroxylases (PHDs), leading to increased expression of genes involved in angiogenesis, erythropoiesis, and glucose metabolism. [, , ] * Modulate epigenetic regulation by inhibiting histone demethylases, impacting gene expression patterns. [, , ] * Affect cellular metabolism by inhibiting enzymes involved in fatty acid metabolism and other metabolic pathways. [, , ]
ANone: The molecular formula of NOG is C4H5NO5, and its molecular weight is 147.09 g/mol.
ANone: While the provided research articles primarily focus on the biological activity and structural studies of NOG in complex with enzymes, they don't provide detailed spectroscopic data like NMR or IR spectra.
A: NOG is primarily known for its inhibitory properties towards 2OG oxygenases. It functions by mimicking the co-substrate and not by directly participating in the catalytic cycle. Thus, NOG is not reported to have intrinsic catalytic properties. [, ]
ANone: NOG is widely employed as a research tool to investigate:
* The biological roles of specific 2OG oxygenases by selectively inhibiting their activity. [, , , ] * Cellular signaling pathways regulated by 2OG oxygenases, such as the hypoxic response and epigenetic modifications. [, , , ] * The therapeutic potential of targeting 2OG oxygenases for diseases like cancer, anemia, and metabolic disorders. [, , ]
A: Computational studies, including molecular docking and molecular dynamics (MD) simulations, have been instrumental in elucidating the binding mode of NOG to the active site of 2OG oxygenases. [, , , ] These studies provide insights into the structural determinants of NOG's inhibitory potency and selectivity.
A: The structure-activity relationship (SAR) of NOG and its derivatives has been explored to identify more potent and selective inhibitors of specific 2OG oxygenases. [, ] Modifications to the N-oxalyl group and the glycine moiety can impact the compound's binding affinity, cellular permeability, and pharmacokinetic properties. [, , , ]
Haftungsausschluss und Informationen zu In-Vitro-Forschungsprodukten
Bitte beachten Sie, dass alle Artikel und Produktinformationen, die auf BenchChem präsentiert werden, ausschließlich zu Informationszwecken bestimmt sind. Die auf BenchChem zum Kauf angebotenen Produkte sind speziell für In-vitro-Studien konzipiert, die außerhalb lebender Organismen durchgeführt werden. In-vitro-Studien, abgeleitet von dem lateinischen Begriff "in Glas", beinhalten Experimente, die in kontrollierten Laborumgebungen unter Verwendung von Zellen oder Geweben durchgeführt werden. Es ist wichtig zu beachten, dass diese Produkte nicht als Arzneimittel oder Medikamente eingestuft sind und keine Zulassung der FDA für die Vorbeugung, Behandlung oder Heilung von medizinischen Zuständen, Beschwerden oder Krankheiten erhalten haben. Wir müssen betonen, dass jede Form der körperlichen Einführung dieser Produkte in Menschen oder Tiere gesetzlich strikt untersagt ist. Es ist unerlässlich, sich an diese Richtlinien zu halten, um die Einhaltung rechtlicher und ethischer Standards in Forschung und Experiment zu gewährleisten.