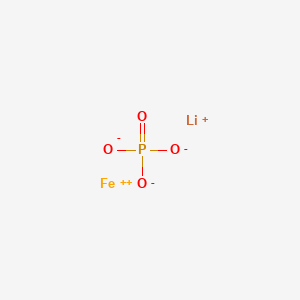
Lithium-Eisenphosphat
Übersicht
Beschreibung
Lithium iron phosphate (LiFePO4), a member of the lithium metal phosphate family, has emerged as a significant cathode material for lithium-ion batteries. It is renowned for its stability, safety, and long cycle life, making it a prime candidate for large format applications such as electric and hybrid electric vehicles . The material's intrinsic thermal stability and continual performance improvements have accelerated its adoption in the industry .
Synthesis Analysis
Several synthesis methods have been developed to optimize the properties of LiFePO4. A low-cost aqueous precipitation technique has been employed to create phase pure, homogeneous, and well-crystallized LiFePO4 by co-precipitating an Fe(II) precursor and subsequent heat treatment in nitrogen . Hydrothermal methods have also been successful, with LiFePO4 being synthesized in a glucose solution, leading to carbon-coated particles that exhibit excellent electrochemical performance . Additionally, spray-pyrolysis followed by sintering has been used to synthesize Mg-doped LiFePO4, which significantly improves electronic conductivity . Supercritical hydrothermal synthesis has been highlighted as a potential industrial-scale method due to its ability to control key parameters like temperature and pressure .
Molecular Structure Analysis
The molecular structure of LiFePO4 has been extensively studied. It is known to crystallize in the olivine structure, which is conducive to the stability and electrochemical performance of the material . The FTIR spectroscopy has been used to probe the local environment in LiFePO4, distinguishing between different phases and types of cationic environments . The structure of novel lithium iron phosphates like LiFe2P3O10 has been determined, showing that it crystallizes in the monoclinic system .
Chemical Reactions Analysis
LiFePO4 undergoes electrochemical reactions during battery operation. The material's electrochemical properties have been systematically measured, showing that Mg-doping can drastically improve its performance . The electrochemical oxidation of lithium iron thio-phosphate, a related material, has shown a potential plateau at around 3.0 V, which is the highest among the sulfides examined .
Physical and Chemical Properties Analysis
The physical and electrochemical properties of LiFePO4 are influenced by the synthesis method. For instance, the sol-gel method has been used to create a porous structure with a carbon layer, which enhances the electrochemical performance . The hydrothermal synthesis of LiFePO4 has resulted in crystalline material with no impurities, which is comparable to those formed by conventional high-temperature synthesis . The electrochemical performance of LiFePO4 has been enhanced by in situ carbon coating, leading to high discharge capacities and excellent cycling stability .
Wissenschaftliche Forschungsanwendungen
LiFePO4 LiFePO_4 LiFePO4
, ist ein vielversprechendes Material mit einer Vielzahl von Anwendungen in der wissenschaftlichen Forschung. Nachfolgend finden Sie eine umfassende Analyse seiner einzigartigen Anwendungen in verschiedenen Bereichen:Batterien für Elektrofahrzeuge
LFP wird häufig als Kathodenmaterial in Lithium-Ionen-Batterien für Elektrofahrzeuge (EVs) verwendet. Seine Popularität beruht auf seiner exzellenten Energiedichte, seiner schnellen Leistungsfähigkeit und seiner Lebensdauer, die für die Automobilindustrie entscheidend sind. LFP-Batterien bieten eine kostengünstige und sicherere Alternative zu anderen Lithium-basierten Batterien und machen sie ideal für die „Dual-Carbon“-Anforderungen der Kohlenstoffbegrenzung und -neutralität .
Energiespeicher im Netzmaßstab
Die Stabilität und Sicherheit von LFP machen es für Energiespeicherlösungen im großen Maßstab geeignet. Es wird zunehmend in Netzspeichersystemen eingesetzt, um Lasten auszugleichen, überschüssige Energie zu speichern und die Zuverlässigkeit erneuerbarer Energiequellen wie Solar- und Windkraft zu verbessern .
Elektrowerkzeuge
LFP-Batterien werden in Elektrowerkzeugen wegen ihrer langen Lebensdauer und Stabilität bevorzugt. Sie liefern eine gleichmäßige Leistungsabgabe und können die hohen Entladeraten bewältigen, die von verschiedenen Elektrowerkzeugen benötigt werden, ohne im Laufe der Zeit nennenswert zu verschlechtern .
Solaranlagen
In Solaranlagen werden LFP-Batterien verwendet, um die Energie zu speichern, die während der Spitzenstunden der Sonneneinstrahlung erzeugt wird. Diese gespeicherte Energie kann dann verwendet werden, wenn Sonnenlicht nicht verfügbar ist, um eine konstante Stromversorgung zu gewährleisten und die Effizienz von Solaranlagen zu verbessern .
Tragbare Elektronik
Obwohl nicht so häufig wie andere Lithium-basierte Batterien, wird LFP in einigen tragbaren elektronischen Geräten verwendet, bei denen Sicherheit und lange Batterielaufzeit Vorrang vor der Energiedichte haben. Seine thermische Stabilität verringert das Risiko von Überhitzung und möglichen Bränden .
Medizinische Geräte
Das zuverlässige und sichere Profil von LFP-Batterien macht sie für medizinische Geräte geeignet, insbesondere für solche, die über längere Zeit eine stabile Stromversorgung benötigen. Ihr geringes Risiko eines thermischen Durchgehens sorgt für die Sicherheit der Patienten .
Anwendungen in der Luftfahrt
Die Sicherheitsmerkmale von LFP sind vorteilhaft bei Anwendungen in der Luftfahrt, wo ein Batterieausfall schwerwiegende Folgen haben kann. Seine stabile Chemie kann den extremen Bedingungen von Luftfahrtanwendungen standhalten .
Marine Ausrüstung
In maritimen Anwendungen werden LFP-Batterien aufgrund ihrer Sicherheit eingesetzt, insbesondere in Bezug auf das geringe Risiko von Feuer und Explosion in einer Salzwasserumgebung. Sie werden auch wegen ihrer Langlebigkeit und ihrer Fähigkeit, Tiefentladungszyklen zu überstehen, ausgewählt .
Wirkmechanismus
Target of Action
Lithium Iron Phosphate (LiFePO4), also known as LFP, is an inorganic compound primarily used as a component in lithium iron phosphate batteries . The primary targets of LiFePO4 are the cathode and anode in a battery, where it facilitates the movement of lithium ions during the charging and discharging process .
Mode of Action
The operation of a LiFePO4 battery involves the movement of lithium ions between the cathode and the anode during the charging and discharging process . This movement allows the battery to efficiently store and release electrical energy . During discharge, lithium ions move from the anode to the cathode through the electrolyte, while electrons flow through the external circuit, creating an electrical current . At the cathode, the lithium ions react with the LiFePO4 material, releasing electrons and forming Li3PO4 .
Biochemical Pathways
The biochemical pathways of LiFePO4 involve the diffusion of lithium ions in the crystal lattices of LiFePO4 . Surface decoration, nanocrystallization, and lattice substitution (doping) are modification approaches widely employed to promote the conductivity of electrons and the diffusion of lithium ions in the crystal lattices of LiFePO4 .
Pharmacokinetics
While the term “pharmacokinetics” is typically used in the context of drug metabolism, in the case of LiFePO4, we can consider its analogous process in terms of its Absorption, Distribution, Metabolism, and Excretion (ADME) within a battery system. The “absorption” and “distribution” occur when lithium ions move from the anode to the cathode during the discharging process . The “metabolism” happens when lithium ions react with the LiFePO4 material at the cathode . The “excretion” is analogous to the charging process, where lithium ions move from the cathode back to the anode .
Result of Action
The result of LiFePO4’s action is the efficient storage and release of electrical energy in a battery system . This allows for longer usage times, reducing the need for frequent recharging and keeping devices powered for longer .
Action Environment
The action of LiFePO4 can be influenced by various environmental factors. For instance, the ordering of LFP and the carbon additive particles facilitates the formation of evenly distributed pores owing to their distinct magnetic properties, which significantly decreases the ionic resistance of the LFP electrode . This modulation of pores and active materials enhances the lithium-ion conduction in the magnetically ordered LFP electrode . Furthermore, the environmental impact of LiFePO4 batteries has been studied, showing that electricity consumption during the manufacture and installation process is the greatest contributor to climate change .
Zukünftige Richtungen
The demand for LFP batteries is expected to grow due to their low cost, high safety, and long cycle life . Research is ongoing to improve the comprehensive performance of LFP cathode materials . There is also interest in developing low-carbon, cost-effective lithium extraction techniques and cathode material regeneration processes .
Eigenschaften
IUPAC Name |
lithium;iron(2+);phosphate | |
---|---|---|
Source | PubChem | |
URL | https://pubchem.ncbi.nlm.nih.gov | |
Description | Data deposited in or computed by PubChem | |
InChI |
InChI=1S/Fe.Li.H3O4P/c;;1-5(2,3)4/h;;(H3,1,2,3,4)/q+2;+1;/p-3 | |
Source | PubChem | |
URL | https://pubchem.ncbi.nlm.nih.gov | |
Description | Data deposited in or computed by PubChem | |
InChI Key |
GELKBWJHTRAYNV-UHFFFAOYSA-K | |
Source | PubChem | |
URL | https://pubchem.ncbi.nlm.nih.gov | |
Description | Data deposited in or computed by PubChem | |
Canonical SMILES |
[Li+].[O-]P(=O)([O-])[O-].[Fe+2] | |
Source | PubChem | |
URL | https://pubchem.ncbi.nlm.nih.gov | |
Description | Data deposited in or computed by PubChem | |
Molecular Formula |
FeLiO4P | |
Record name | Lithium iron phosphate | |
Source | Wikipedia | |
URL | https://en.wikipedia.org/wiki/Lithium_iron_phosphate | |
Description | Chemical information link to Wikipedia. | |
Source | PubChem | |
URL | https://pubchem.ncbi.nlm.nih.gov | |
Description | Data deposited in or computed by PubChem | |
DSSTOX Substance ID |
DTXSID20571409 | |
Record name | Iron(2+) lithium phosphate (1/1/1) | |
Source | EPA DSSTox | |
URL | https://comptox.epa.gov/dashboard/DTXSID20571409 | |
Description | DSSTox provides a high quality public chemistry resource for supporting improved predictive toxicology. | |
Molecular Weight |
157.8 g/mol | |
Source | PubChem | |
URL | https://pubchem.ncbi.nlm.nih.gov | |
Description | Data deposited in or computed by PubChem | |
CAS RN |
15365-14-7 | |
Record name | Phosphoric acid, iron(2+) lithium salt (1:1:1) | |
Source | ChemIDplus | |
URL | https://pubchem.ncbi.nlm.nih.gov/substance/?source=chemidplus&sourceid=0015365147 | |
Description | ChemIDplus is a free, web search system that provides access to the structure and nomenclature authority files used for the identification of chemical substances cited in National Library of Medicine (NLM) databases, including the TOXNET system. | |
Record name | Phosphoric acid, iron(2+) lithium salt (1:1:1) | |
Source | EPA Chemicals under the TSCA | |
URL | https://www.epa.gov/chemicals-under-tsca | |
Description | EPA Chemicals under the Toxic Substances Control Act (TSCA) collection contains information on chemicals and their regulations under TSCA, including non-confidential content from the TSCA Chemical Substance Inventory and Chemical Data Reporting. | |
Record name | Iron(2+) lithium phosphate (1/1/1) | |
Source | EPA DSSTox | |
URL | https://comptox.epa.gov/dashboard/DTXSID20571409 | |
Description | DSSTox provides a high quality public chemistry resource for supporting improved predictive toxicology. | |
Record name | Phosphoric acid, iron(2+) lithium salt (1:1:1) | |
Source | European Chemicals Agency (ECHA) | |
URL | https://echa.europa.eu/substance-information/-/substanceinfo/100.124.705 | |
Description | The European Chemicals Agency (ECHA) is an agency of the European Union which is the driving force among regulatory authorities in implementing the EU's groundbreaking chemicals legislation for the benefit of human health and the environment as well as for innovation and competitiveness. | |
Explanation | Use of the information, documents and data from the ECHA website is subject to the terms and conditions of this Legal Notice, and subject to other binding limitations provided for under applicable law, the information, documents and data made available on the ECHA website may be reproduced, distributed and/or used, totally or in part, for non-commercial purposes provided that ECHA is acknowledged as the source: "Source: European Chemicals Agency, http://echa.europa.eu/". Such acknowledgement must be included in each copy of the material. ECHA permits and encourages organisations and individuals to create links to the ECHA website under the following cumulative conditions: Links can only be made to webpages that provide a link to the Legal Notice page. | |
Retrosynthesis Analysis
AI-Powered Synthesis Planning: Our tool employs the Template_relevance Pistachio, Template_relevance Bkms_metabolic, Template_relevance Pistachio_ringbreaker, Template_relevance Reaxys, Template_relevance Reaxys_biocatalysis model, leveraging a vast database of chemical reactions to predict feasible synthetic routes.
One-Step Synthesis Focus: Specifically designed for one-step synthesis, it provides concise and direct routes for your target compounds, streamlining the synthesis process.
Accurate Predictions: Utilizing the extensive PISTACHIO, BKMS_METABOLIC, PISTACHIO_RINGBREAKER, REAXYS, REAXYS_BIOCATALYSIS database, our tool offers high-accuracy predictions, reflecting the latest in chemical research and data.
Strategy Settings
Precursor scoring | Relevance Heuristic |
---|---|
Min. plausibility | 0.01 |
Model | Template_relevance |
Template Set | Pistachio/Bkms_metabolic/Pistachio_ringbreaker/Reaxys/Reaxys_biocatalysis |
Top-N result to add to graph | 6 |
Feasible Synthetic Routes
Haftungsausschluss und Informationen zu In-Vitro-Forschungsprodukten
Bitte beachten Sie, dass alle Artikel und Produktinformationen, die auf BenchChem präsentiert werden, ausschließlich zu Informationszwecken bestimmt sind. Die auf BenchChem zum Kauf angebotenen Produkte sind speziell für In-vitro-Studien konzipiert, die außerhalb lebender Organismen durchgeführt werden. In-vitro-Studien, abgeleitet von dem lateinischen Begriff "in Glas", beinhalten Experimente, die in kontrollierten Laborumgebungen unter Verwendung von Zellen oder Geweben durchgeführt werden. Es ist wichtig zu beachten, dass diese Produkte nicht als Arzneimittel oder Medikamente eingestuft sind und keine Zulassung der FDA für die Vorbeugung, Behandlung oder Heilung von medizinischen Zuständen, Beschwerden oder Krankheiten erhalten haben. Wir müssen betonen, dass jede Form der körperlichen Einführung dieser Produkte in Menschen oder Tiere gesetzlich strikt untersagt ist. Es ist unerlässlich, sich an diese Richtlinien zu halten, um die Einhaltung rechtlicher und ethischer Standards in Forschung und Experiment zu gewährleisten.