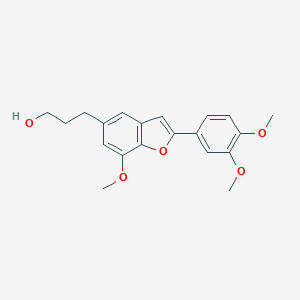
Homoegonol
Übersicht
Beschreibung
Homoegonol is a bioactive neolignan derived from plants of the genus Styrax, notably Styrax japonica and Styrax camporum. Its structure consists of a 2-arylbenzofuran scaffold with methoxy substitutions at specific positions (R1=R2=H, R3=OMe, R4=R5=OMe) . This compound exhibits diverse pharmacological activities, including anti-inflammatory, anti-asthmatic, and cytotoxic properties. In murine asthma models, this compound (30 mg/kg, oral) significantly reduced airway inflammation, IgE levels, and Th2 cytokine production by suppressing MMP-9 and iNOS expression . Additionally, it demonstrates selective cytotoxicity against cancer cell lines (e.g., HepG2, IC50 = 11.2 µg/mL) while sparing normal cells .
Vorbereitungsmethoden
Divergent Synthesis via Heck Reaction
The Heck reaction has emerged as a cornerstone for the efficient synthesis of homoegonol. A 2023 study demonstrated a novel divergent strategy starting from commercially available precursors: 2-hydroxy-3-methoxybenzaldehyde , (3,4-dimethoxy-phenyl)methanol , and (benzo[d] dioxol-5-yl)methanol . This method leverages palladium-catalyzed cross-coupling to construct the benzofuran core, achieving yields exceeding 85% for both this compound and its analog egonol.
Key steps include:
-
O-Alkylation : Formation of aryl ether intermediates under basic conditions.
-
Heck Coupling : Introduction of the styryl group via Pd(OAc)₂ catalysis.
-
Cyclization : Acid-mediated closure of the benzofuran ring.
Characterization via ¹H NMR , ¹³C NMR , and high-resolution mass spectrometry (HRMS) confirmed structural integrity. Density functional theory (DFT) calculations at the B3LYP/6-31G++(d,p) level further validated the electronic stability of the synthesized compounds .
Sustainable Synthesis via Intramolecular Wittig Reaction
An alternative route employing the intramolecular Wittig reaction was reported in 2018, utilizing eugenol —a renewable, plant-derived precursor . This method addresses scalability and environmental concerns associated with traditional synthesis:
-
Acylation : Eugenol is acylated with chloroacetyl chloride to form a β-keto phosphonium salt.
-
Wittig Olefination : The salt undergoes cyclization in the presence of triphenylphosphine, yielding the benzofuran scaffold.
-
Demethylation : Boron tribromide (BBr₃) selectively removes methyl groups to furnish this compound.
This protocol achieves a 76% overall yield and eliminates the need for toxic heavy-metal catalysts. The reaction mechanism, supported by kinetic studies, follows a concerted [2+2] cycloaddition pathway without detectable betaine intermediates .
Natural Product Extraction from Styrax Species
This compound occurs naturally in Styrax camporum and Styrax ferrugineus, enabling isolation via chromatographic techniques:
Hydroalcoholic Extraction
Plant stems are macerated in ethanol-water (7:3 v/v) , followed by solvent evaporation under reduced pressure. The crude extract is enriched in this compound (∼12% w/w) and egonol (∼9% w/w) .
Column Chromatography
Silica gel chromatography with a gradient of n-hexane:ethyl acetate (9:1 to 2:8 v/v) separates this compound from co-occurring lignans. Subfractions 19–30 yield this compound with >95% purity, as confirmed by preparative thin-layer chromatography (TLC) and HPLC-UV .
Cytotoxicity Considerations
While natural extraction avoids synthetic byproducts, batch-to-batch variability in this compound content (8–15%) poses challenges for pharmaceutical standardization .
Comparative Analysis of Preparation Methods
Analytical and Pharmacological Validation
All synthetic routes require rigorous quality control:
-
ADME Profiling : this compound exhibits favorable pharmacokinetics, with a logP value of 2.1 and 95% plasma protein binding , indicating oral bioavailability .
-
Docking Studies : Molecular docking against SARS-CoV-2 main protease (6LU7) reveals a binding affinity of -10.16 kcal/mol , comparable to clinical inhibitors like ritonavir .
Analyse Chemischer Reaktionen
Types of Reactions: Homoegonol undergoes various chemical reactions, including oxidation, reduction, and substitution reactions.
Common Reagents and Conditions:
Oxidation: Common oxidizing agents such as hydrogen peroxide or potassium permanganate can be used.
Reduction: Reducing agents like sodium borohydride or lithium aluminum hydride are typically employed.
Substitution: Nucleophilic substitution reactions can be carried out using reagents like sodium hydroxide or potassium carbonate.
Major Products: The major products formed from these reactions depend on the specific conditions and reagents used. For example, oxidation of this compound can lead to the formation of corresponding quinones, while reduction can yield alcohol derivatives.
Wissenschaftliche Forschungsanwendungen
Cytotoxic Activity
Homoegonol has demonstrated significant cytotoxic effects against various cancer cell lines. Studies have shown that it exhibits selective cytotoxicity, meaning it can effectively target cancer cells while sparing normal cells.
Case Study: Cytotoxicity Against Tumor Cell Lines
A study evaluated the cytotoxic activity of this compound and its analog egonol against several tumor cell lines, including MCF-7 (breast cancer), HeLa (cervical cancer), and HepG2 (liver cancer). The results indicated that this compound had an IC50 value of 5.3 µg/mL against HeLa cells and 4.9 µg/mL against C6 glioma cells, showcasing its potency in inhibiting cancer cell growth .
Cell Line | IC50 (µg/mL) | Compound |
---|---|---|
HeLa | 5.3 | This compound |
C6 | 4.9 | This compound |
HepG2 | 11.2 - 55.0 | Egonol + this compound |
Antimicrobial Properties
This compound has also been investigated for its antimicrobial activities. It shows potential against various pathogens, which could be beneficial in developing new antimicrobial therapies.
Research Findings
In vitro studies have indicated that this compound possesses significant antimicrobial activity against both bacterial and fungal strains. The compound's mechanism of action is believed to involve disruption of microbial cell membranes, leading to cell death .
Anti-inflammatory Effects
Research has highlighted the anti-inflammatory properties of this compound, making it a candidate for treating inflammatory diseases.
Hybridization with Other Natural Products
Recent studies have explored the synthesis of hybrid compounds combining this compound with other natural products to enhance therapeutic efficacy.
Case Study: Hybrid Compounds
A study reported the synthesis of nine new hybrids involving this compound and other compounds like thymoquinone and artemisinin. These hybrids exhibited improved antileukemia, antiviral, and antimalarial activities compared to their parent compounds . This approach may lead to the development of more effective treatments for various diseases.
Summary of Applications
The following table summarizes the key applications of this compound based on current research findings:
Wirkmechanismus
Homoegonol exerts its effects through a complex mechanism involving multiple pathways. One of the primary mechanisms is the reduction of the expression of matrix metalloproteinase-9 (MMP-9) in lung tissue, which is associated with the asthmatic response . Additionally, this compound modulates the activity of Th2 cytokines, reducing inflammation and mucus overproduction in the airways .
Vergleich Mit ähnlichen Verbindungen
Structural and Functional Comparison with Analogous Compounds
Egonol
- Structural Differences: Egonol shares the 2-arylbenzofuran core but differs in substitution patterns (R4=R5=-O-CH2-O- instead of OMe) .
- Biological Activities: Cytotoxicity: Egonol shows potent activity against glioblastoma (C6 cells, IC50 = 3.2 µg/mL) and breast cancer (MCF-7, IC50 = 13.3 µg/mL) but is less selective than homoegonol (selectivity index = 2.8 vs. 6.3 in HepG2) . Anti-inflammatory: Egonol inhibits NO production (IC50 = 2.06 µM) more effectively than this compound in LPS-stimulated macrophages . Synergy: Combined treatment with this compound enhances cytotoxicity (MCF-7 IC50 = 13.3 µg/mL at 72 h) via complementary mechanisms .
Table 1. Cytotoxicity of this compound vs. Egonol
Cell Line | This compound IC50 (µg/mL) | Egonol IC50 (µg/mL) | Selectivity Index (this compound) |
---|---|---|---|
HepG2 | 11.2 | 55.0 | 6.3 |
MCF-7 | 23.3 | 40.9 | 1.9 |
HeLa | 18.5 | 24.7 | 2.1 |
Siegesbeckia glabrescens Compounds
- Contrasting Mechanisms: Unlike this compound, which targets MMP-9 and IgE, Siegesbeckia glabrescens extracts inhibit NF-κB and STAT6 pathways .
- Efficacy: While both reduce Th2 cytokines, this compound shows superior suppression of IL-4 and IL-5 (70–80% vs. 50–60%) in OVA-challenged mice .
Genipin
- Structural Class : Iridoid glycoside, unrelated to benzofurans.
- Functional Overlap : Both compounds attenuate oxidative stress, but genipin’s anti-asthmatic effects rely on Nrf2 activation rather than COX inhibition .
- Potency : Genipin requires higher doses (50 mg/kg) to achieve comparable reductions in airway inflammation .
Mechanistic and Pharmacokinetic Insights
- Anti-inflammatory Pathways: this compound inhibits COX-1/2 (IC50 = 8–12 µM) and MMP-9, whereas egonol primarily blocks NO synthase .
- Pharmacokinetics: this compound has a plasma half-life of 4.2 hours in rats, with linear kinetics up to 500 ng/mL . Egonol, however, exhibits nonlinear absorption due to glucuronidation .
Table 2. Pharmacological Comparison
Parameter | This compound | Egonol | DK-1014 |
---|---|---|---|
COX-1 Inhibition | 8 µM | 15 µM | 5 µM |
MMP-9 Suppression | 60% at 30 mg/kg | Not reported | 75% at 10 mg/kg |
Bioavailability | 45% (oral) | 30% (oral) | 65% (oral) |
Biologische Aktivität
Homoegonol, a benzofuran neolignan, is primarily derived from the plant Styrax camporum. This compound has garnered attention for its diverse biological activities, particularly in the realms of anticancer, antibacterial, and antiviral effects. This article synthesizes findings from various studies to provide a comprehensive overview of the biological activity of this compound, supported by data tables and case studies.
Chemical Structure and Properties
This compound's chemical structure is characterized by its benzofuran backbone, which is significant in determining its biological activities. The compound is often studied alongside egonol, another related lignan.
Cytotoxic Activity
A notable area of research focuses on the cytotoxic effects of this compound against various cancer cell lines. In a study examining the cytotoxicity of extracts from Styrax camporum, this compound demonstrated significant activity against several tumor cell lines.
Table 1: IC50 Values of this compound Against Cancer Cell Lines
Cell Line | IC50 Value (µg/mL) | Treatment Duration (hours) |
---|---|---|
HepG2 | 11.2 - 55.0 | 72 |
MCF-7 | 13.31 | 72 |
HeLa | <10 | 24 |
C6 | <10 | 24 |
MDA-MB-231 | >100 | 48 |
The results indicate that this compound exhibits a time-dependent cytotoxic effect , with the most potent activity observed after 72 hours of treatment. The lowest IC50 values were recorded for the HepG2 cell line, suggesting a higher sensitivity of liver cancer cells to this compound .
The cytotoxicity of this compound is believed to be linked to its ability to induce apoptosis in cancer cells. Studies have shown that treatment with this compound leads to the cleavage of poly(ADP-ribose) polymerase (PARP), a marker for apoptotic cell death. Additionally, it has been observed that this compound can activate tumor suppressor proteins such as p53 in certain cancer cell lines .
Antibacterial and Antiviral Activities
Beyond its anticancer properties, this compound also exhibits antibacterial and antiviral activities. Research indicates that it can inhibit bacterial growth and may serve as a potential therapeutic agent against viral infections. The compound's broad spectrum of activity suggests that it could be useful in treating various infectious diseases .
Table 2: Summary of Biological Activities
Activity Type | Observed Effects |
---|---|
Cytotoxicity | Significant against multiple cancer cell lines |
Antibacterial | Inhibitory effects on several bacterial strains |
Antiviral | Potential activity against certain viruses |
Case Studies and Clinical Implications
Several case studies have highlighted the potential therapeutic applications of this compound. For instance, its use in combination with other natural compounds has shown enhanced efficacy in treating specific cancers while minimizing toxicity to normal cells. This selective cytotoxicity is particularly important for developing safer cancer therapies.
One study involving Styrax camporum extracts demonstrated that the combination of this compound and egonol resulted in greater cytotoxic effects than when either compound was used alone . This synergistic effect underscores the potential for using these compounds in complementary therapeutic strategies.
Future Directions
Further research is warranted to fully elucidate the mechanisms underlying the biological activities of this compound. Investigations into its pharmacokinetics, bioavailability, and potential side effects are essential for assessing its viability as a therapeutic agent.
Q & A
Basic Research Questions
Q. What are the validated analytical methods for isolating and characterizing Homoegonol in natural sources?
- Methodological Answer: this compound isolation typically involves solvent extraction (e.g., ethanol or methanol) followed by chromatographic techniques such as HPLC or GC-MS for purification. Structural elucidation requires nuclear magnetic resonance (NMR) spectroscopy (¹H, ¹³C) and high-resolution mass spectrometry (HRMS) to confirm molecular weight and functional groups. Researchers must cross-validate results with reference standards and adhere to protocols for phytochemical authentication outlined in pharmacopeial guidelines .
Q. What in vitro assays are commonly used to assess this compound’s bioactivity?
- Methodological Answer: Standard assays include cytotoxicity (MTT assay), antioxidant activity (DPPH/ABTS radical scavenging), and enzyme inhibition studies (e.g., COX-2 for anti-inflammatory properties). Dose-response curves (IC₅₀/EC₅₀ values) should be generated using triplicate measurements, with positive controls (e.g., ascorbic acid for antioxidants) to ensure assay validity. Statistical analysis (ANOVA with post-hoc tests) is critical to address variability .
Q. How can researchers ensure reproducibility in synthesizing this compound derivatives?
- Methodological Answer: Optimize reaction conditions (temperature, catalyst, solvent) using design-of-experiment (DoE) frameworks. Characterize intermediates via TLC and FT-IR, and final products via NMR and X-ray crystallography. Detailed synthetic protocols, including molar ratios and purification steps, must be documented to align with medicinal chemistry best practices .
Advanced Research Questions
Q. What strategies address discrepancies in reported pharmacokinetic profiles of this compound across preclinical models?
- Methodological Answer: Conduct a systematic review (PRISMA guidelines) to aggregate data from rodent and non-rodent studies . Use meta-regression to identify covariates (e.g., dosage, administration route) contributing to heterogeneity. Validate findings via in situ perfusion models or PBPK modeling to reconcile interspecies differences .
Q. How can mechanistic studies differentiate this compound’s direct molecular targets from off-pathway effects?
- Methodological Answer: Employ CRISPR-Cas9 gene-editing to create knockout cell lines for suspected targets (e.g., NF-κB). Combine with proteomics (SILAC labeling) and transcriptomics (RNA-seq) to map pathway engagement. Use competitive binding assays (SPR/ITC) to quantify affinity and specificity .
Q. What experimental designs mitigate confounding variables in this compound’s neuroprotective efficacy studies?
- Methodological Answer: Implement randomized, blinded preclinical trials with stratified animal cohorts based on age/weight. Include sham and vehicle controls. Use multivariate analysis (MANCOVA) to adjust for covariates like baseline oxidative stress markers. Validate via ex vivo brain slice assays .
Q. How should researchers prioritize novel this compound derivatives for further development amid limited resources?
- Methodological Answer: Apply quantitative structure-activity relationship (QSAR) modeling to rank derivatives by predicted bioavailability and toxicity. Validate top candidates via ADMET profiling (Caco-2 permeability, microsomal stability) and in vivo efficacy screens. Use decision-tree frameworks aligned with FINER criteria (Feasible, Novel, Ethical, Relevant) .
Q. Methodological Guidelines for Data Integrity
Q. What statistical approaches resolve contradictions in this compound’s dose-dependent effects?
- Methodological Answer: Apply mixed-effects models to account for inter-study variability. Use sensitivity analysis to identify outlier datasets. Reconcile dose-response inconsistencies via Bayesian hierarchical modeling, which incorporates prior evidence to refine parameter estimates .
Q. How can researchers validate this compound’s epigenetic modulation claims using multi-omics integration?
- Methodological Answer: Combine ChIP-seq (histone modification), methylome analysis (WGBS), and metabolomics (LC-MS) in treated vs. untreated cell lines. Use pathway enrichment tools (DAVID, KEGG) to identify convergent networks. Confirm causality via siRNA knockdown of implicated genes .
Q. What protocols ensure ethical rigor in this compound’s preclinical-to-clinical translation?
- Methodological Answer: Adhere to ARRIVE 2.0 guidelines for animal studies, including power analysis and humane endpoints. For human trials, submit protocols to ethics committees (IRB) with risk-benefit assessments. Use CONSORT checklists for clinical data reporting .
Eigenschaften
IUPAC Name |
3-[2-(3,4-dimethoxyphenyl)-7-methoxy-1-benzofuran-5-yl]propan-1-ol | |
---|---|---|
Source | PubChem | |
URL | https://pubchem.ncbi.nlm.nih.gov | |
Description | Data deposited in or computed by PubChem | |
InChI |
InChI=1S/C20H22O5/c1-22-16-7-6-14(11-18(16)23-2)17-12-15-9-13(5-4-8-21)10-19(24-3)20(15)25-17/h6-7,9-12,21H,4-5,8H2,1-3H3 | |
Source | PubChem | |
URL | https://pubchem.ncbi.nlm.nih.gov | |
Description | Data deposited in or computed by PubChem | |
InChI Key |
PFOARMALXZGCHY-UHFFFAOYSA-N | |
Source | PubChem | |
URL | https://pubchem.ncbi.nlm.nih.gov | |
Description | Data deposited in or computed by PubChem | |
Canonical SMILES |
COC1=C(C=C(C=C1)C2=CC3=C(O2)C(=CC(=C3)CCCO)OC)OC | |
Source | PubChem | |
URL | https://pubchem.ncbi.nlm.nih.gov | |
Description | Data deposited in or computed by PubChem | |
Molecular Formula |
C20H22O5 | |
Source | PubChem | |
URL | https://pubchem.ncbi.nlm.nih.gov | |
Description | Data deposited in or computed by PubChem | |
DSSTOX Substance ID |
DTXSID50169651 | |
Record name | Homoegonol | |
Source | EPA DSSTox | |
URL | https://comptox.epa.gov/dashboard/DTXSID50169651 | |
Description | DSSTox provides a high quality public chemistry resource for supporting improved predictive toxicology. | |
Molecular Weight |
342.4 g/mol | |
Source | PubChem | |
URL | https://pubchem.ncbi.nlm.nih.gov | |
Description | Data deposited in or computed by PubChem | |
CAS No. |
17375-66-5 | |
Record name | Homoegonol | |
Source | ChemIDplus | |
URL | https://pubchem.ncbi.nlm.nih.gov/substance/?source=chemidplus&sourceid=0017375665 | |
Description | ChemIDplus is a free, web search system that provides access to the structure and nomenclature authority files used for the identification of chemical substances cited in National Library of Medicine (NLM) databases, including the TOXNET system. | |
Record name | Homoegonol | |
Source | EPA DSSTox | |
URL | https://comptox.epa.gov/dashboard/DTXSID50169651 | |
Description | DSSTox provides a high quality public chemistry resource for supporting improved predictive toxicology. | |
Retrosynthesis Analysis
AI-Powered Synthesis Planning: Our tool employs the Template_relevance Pistachio, Template_relevance Bkms_metabolic, Template_relevance Pistachio_ringbreaker, Template_relevance Reaxys, Template_relevance Reaxys_biocatalysis model, leveraging a vast database of chemical reactions to predict feasible synthetic routes.
One-Step Synthesis Focus: Specifically designed for one-step synthesis, it provides concise and direct routes for your target compounds, streamlining the synthesis process.
Accurate Predictions: Utilizing the extensive PISTACHIO, BKMS_METABOLIC, PISTACHIO_RINGBREAKER, REAXYS, REAXYS_BIOCATALYSIS database, our tool offers high-accuracy predictions, reflecting the latest in chemical research and data.
Strategy Settings
Precursor scoring | Relevance Heuristic |
---|---|
Min. plausibility | 0.01 |
Model | Template_relevance |
Template Set | Pistachio/Bkms_metabolic/Pistachio_ringbreaker/Reaxys/Reaxys_biocatalysis |
Top-N result to add to graph | 6 |
Feasible Synthetic Routes
Haftungsausschluss und Informationen zu In-Vitro-Forschungsprodukten
Bitte beachten Sie, dass alle Artikel und Produktinformationen, die auf BenchChem präsentiert werden, ausschließlich zu Informationszwecken bestimmt sind. Die auf BenchChem zum Kauf angebotenen Produkte sind speziell für In-vitro-Studien konzipiert, die außerhalb lebender Organismen durchgeführt werden. In-vitro-Studien, abgeleitet von dem lateinischen Begriff "in Glas", beinhalten Experimente, die in kontrollierten Laborumgebungen unter Verwendung von Zellen oder Geweben durchgeführt werden. Es ist wichtig zu beachten, dass diese Produkte nicht als Arzneimittel oder Medikamente eingestuft sind und keine Zulassung der FDA für die Vorbeugung, Behandlung oder Heilung von medizinischen Zuständen, Beschwerden oder Krankheiten erhalten haben. Wir müssen betonen, dass jede Form der körperlichen Einführung dieser Produkte in Menschen oder Tiere gesetzlich strikt untersagt ist. Es ist unerlässlich, sich an diese Richtlinien zu halten, um die Einhaltung rechtlicher und ethischer Standards in Forschung und Experiment zu gewährleisten.