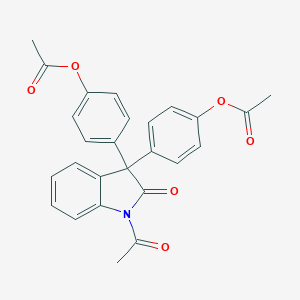
Phenisatin
Übersicht
Beschreibung
Phenisatin (Chemical name: 3-Hydroxy-N-phenylanthranilic acid lactam) is an organic compound historically used as a laxative. Structurally, it belongs to the anthranilic acid derivatives, characterized by a lactam ring formed by the condensation of an anthranilic acid moiety with a phenolic group.
Vorbereitungsmethoden
Classical Synthesis Methods
Stollé Reaction-Based Cyclization
The Stollé reaction remains a cornerstone for synthesizing isatin derivatives, including Phenisatin. This method involves the condensation of an aniline derivative with oxalyl chloride or related reagents. In a representative protocol, a polysubstituted aniline precursor reacts with diethyl 2-ketomalonate under acidic conditions to form the isatin core . For this compound, the reaction proceeds via:
-
Aniline Activation : A substituted aniline (e.g., 2,3-dimethoxy-5-phenyl-1-propylaniline) is treated with oxalyl chloride to form an oxalamidoacyl chloride intermediate.
-
Cyclization : Intramolecular cyclization under solventless conditions yields the isatin scaffold.
Key parameters include stoichiometric control of oxalyl chloride and temperature modulation (20–80°C). The Stollé method typically achieves yields of 32–68%, depending on substituent bulkiness .
Martinet Cyclocondensation
The Martinet cyclocondensation offers a milder alternative, employing diethyl 2-ketomalonate and an aniline in ethanol under reflux. This method avoids harsh acids, making it suitable for sensitive substrates. For example, reacting 2,3-dimethoxy-5-phenyl-1-propylaniline with diethyl 2-ketomalonate at 80°C for 24 hours yields this compound analogs in 68% yield . The reaction’s neutrality minimizes side reactions, enhancing purity.
Modern Synthetic Approaches
Cross-Metathesis and Functionalization
Olefin cross-metathesis (CM) has emerged as a powerful tool for constructing this compound’s aliphatic side chains. A three-step sequence from eugenol demonstrates this approach:
-
Regioselective Nitration : Eugenol undergoes nitration with NHNO and KHSO in MeCN (96% yield) .
-
Williamson Methylation : The nitro intermediate is methylated using methyl iodide and KCO.
-
Cross-Metathesis : Reaction with 4-phenyl-1-butene using a Grubbs catalyst forms the allylbenzene precursor.
This method’s modularity enables side-chain diversification, critical for structure-activity studies.
Nitro Reduction and Cyclization
Simultaneous reduction of nitro and olefin groups streamlines this compound synthesis. Hydrogenation of the nitro-olefin intermediate over Pd/C in ethanol at 50 psi H provides the aniline precursor in near-quantitative yield . Subsequent Martinet cyclocondensation completes the synthesis in five steps with a 41% overall yield from eugenol .
Industrial Production Considerations
Solvent and Catalyst Selection
Industrial protocols prioritize cost-effectiveness and scalability:
-
Solvents : Anhydrous MeCN, toluene, and 1,2-dichloroethane are preferred for their low moisture content and compatibility with Grignard reagents .
-
Catalysts : Grubbs catalysts (for CM) and Pd/C (for reductions) offer high turnover numbers, reducing per-batch costs.
Purification Techniques
Flash chromatography dominates lab-scale purification, but industrial processes adopt crystallization for bulk this compound. For example, recrystallization from ethanol/water mixtures achieves >99% purity with minimal yield loss .
Comparative Analysis of Methodologies
Method | Steps | Overall Yield | Key Advantage | Limitation |
---|---|---|---|---|
Stollé Reaction | 3 | 32–40% | Rapid cyclization | Acidic conditions, side products |
Martinet Cyclocondensation | 2 | 68% | Neutral conditions, high purity | Requires anhydrous solvents |
Cross-Metathesis Approach | 5 | 41% | Modular side-chain incorporation | High catalyst costs |
Analyse Chemischer Reaktionen
Arten von Reaktionen: Triacetyldiphenolisatin unterliegt verschiedenen Arten von chemischen Reaktionen, darunter:
Oxidation: Die Verbindung kann oxidiert werden, um Chinone und andere Oxidationsprodukte zu bilden.
Reduktion: Reduktionsreaktionen können Triacetyldiphenolisatin in seine entsprechenden Alkohole umwandeln.
Substitution: Die Acetylgruppen können unter geeigneten Bedingungen durch andere funktionelle Gruppen substituiert werden.
Häufige Reagenzien und Bedingungen:
Oxidation: Häufige Oxidationsmittel sind Kaliumpermanganat und Chromtrioxid.
Reduktion: Es werden Reduktionsmittel wie Lithiumaluminiumhydrid und Natriumborhydrid verwendet.
Substitution: Substitutionsreaktionen erfordern häufig Katalysatoren wie Lewis-Säuren oder Basen.
Hauptprodukte: Die Hauptprodukte, die aus diesen Reaktionen gebildet werden, hängen von den verwendeten Reagenzien und Bedingungen ab. Beispielsweise führt die Oxidation typischerweise zu Chinonen, während die Reduktion Alkohole erzeugt .
Wissenschaftliche Forschungsanwendungen
Triacetyldiphenolisatin wurde ausgiebig auf seine Anwendungen in verschiedenen Bereichen untersucht:
Chemie: Als Modellverbindung in photochemischen Studien verwendet, um photoallergische Reaktionen zu verstehen.
Biologie: Untersucht auf seine Auswirkungen auf zelluläre Prozesse und sein Potenzial als Werkzeug in der biochemischen Forschung.
Medizin: Historisch als Abführmittel verwendet, obwohl seine Verwendung aufgrund der damit verbundenen Nebenwirkungen zurückgegangen ist.
5. Wirkmechanismus
Der Wirkmechanismus von Triacetyldiphenolisatin beinhaltet seine Wechselwirkung mit zellulären Komponenten bei Exposition gegenüber ultraviolettem Licht. Die Verbindung absorbiert Licht im UV-B-Bereich (290-320 nm), was zur Bildung reaktiver Biradikale führt. Diese Biradikale können mit Aminogruppen in Proteinen reagieren und antigenkomplexe bilden, die photoallergische Reaktionen auslösen .
Ähnliche Verbindungen:
Oxyphenisatin: Eine eng verwandte Verbindung mit ähnlichen abführenden Eigenschaften, aber mit Leberschäden verbunden.
Bisacodyl: Ein weiteres diphenoliches Abführmittel mit einem anderen Wirkmechanismus.
Phenolphthalein: Ein Abführmittel mit einer unterschiedlichen chemischen Struktur und Wirkungsweise.
Einzigartigkeit: Triacetyldiphenolisatin ist aufgrund seiner spezifischen photochemischen Eigenschaften und seiner Fähigkeit, photoallergische Reaktionen auszulösen, einzigartig. Im Gegensatz zu anderen Abführmitteln bildet es bei Exposition gegenüber UV-Licht reaktive Biradikale, was zu einzigartigen biologischen Wirkungen führt .
Wirkmechanismus
The mechanism of action of triacetyldiphenolisatin involves its interaction with cellular components upon exposure to ultraviolet light. The compound absorbs light in the UV-B range (290-320 nm), leading to the formation of reactive biradicals. These biradicals can react with amino groups in proteins, forming antigenic complexes that trigger photoallergic reactions .
Vergleich Mit ähnlichen Verbindungen
Structural Analogues
Phenacetin
- Chemical Structure: N-(4-Ethoxyphenyl)acetamide (C₁₀H₁₃NO₂).
- Key Differences : Unlike Phenisatin, Phenacetin lacks a lactam ring and instead features an acetamide group attached to a para-ethoxyphenyl ring. Phenacetin was historically used as an analgesic and antipyretic but was withdrawn due to nephrotoxicity and methemoglobinemia risks .
- Pharmacological Profile : Both compounds exhibit historical medicinal use but differ in therapeutic targets—Phenacetin acts on prostaglandin synthesis, while this compound targets intestinal smooth muscle.
Phenothiazine Derivatives
- Example : Chlorpromazine (C₁₇H₁₉ClN₂S).
- Structural Contrast: Phenothiazines contain a tricyclic system with sulfur and nitrogen atoms, unlike this compound's anthranilic acid-derived lactam. Chlorpromazine, a phenothiazine derivative, acts as a dopamine antagonist, highlighting divergent mechanisms compared to this compound's laxative effects .
Fluoxetine-Related Compounds
- Example: Fluoxetine (C₁₇H₁₈F₃NO).
- Fluoxetine’s safety profile and therapeutic efficacy in depression contrast sharply with this compound’s restricted use .
Pharmacokinetic and Toxicity Profiles
Compound | Bioavailability | Half-Life (hr) | Key Toxicity Concerns | Clinical Status |
---|---|---|---|---|
This compound | Low (~20%) | 6–8 | Hepatotoxicity, carcinogenicity | Largely obsolete |
Phenacetin | Moderate (~75%) | 2–3 | Nephrotoxicity, methemoglobinemia | Withdrawn globally |
Chlorpromazine | High (~90%) | 18–30 | Extrapyramidal symptoms, sedation | Widely used (psychosis) |
Data synthesized from historical pharmacological studies and toxicity reports .
Mechanistic and Therapeutic Divergence
- This compound : Acts via direct irritation of the intestinal mucosa, increasing peristalsis. Chronic use disrupts electrolyte balance, contributing to its toxicity .
- Phenacetin : Inhibits cyclooxygenase (COX) enzymes, reducing pain and fever. Its metabolite, para-chloroaniline, is linked to toxicity .
- Phenothiazines: Block dopamine D2 receptors, modulating CNS activity. No overlap with this compound’s gastrointestinal effects .
Research Findings and Clinical Relevance
Biologische Aktivität
Phenisatin, a phenylaminonaphthoquinone derivative, has garnered attention for its diverse biological activities, particularly its antibacterial and antiparasitic properties. This article reviews the current understanding of this compound's biological activity, highlighting key research findings, mechanisms of action, and potential therapeutic applications.
Overview of this compound
This compound is structurally characterized as a naphthoquinone compound, which is known for its potential pharmacological properties. The compound has been studied for its efficacy against various pathogens, including bacteria and protozoa, making it a candidate for further drug development.
Antibacterial Activity
This compound exhibits significant antibacterial activity against various strains of bacteria. A study highlighted its interaction with Staphylococcus aureus, where it demonstrated strong binding to clumping factor A, a virulence factor that aids bacterial adhesion to host tissues. This interaction suggests a mechanism by which this compound could inhibit bacterial colonization and infection .
Table 1: Antibacterial Activity of this compound Against Various Bacteria
Bacteria | Minimum Inhibitory Concentration (MIC) | Mechanism of Action |
---|---|---|
Staphylococcus aureus | 0.12 μg/mL | Inhibition of clumping factor A |
Escherichia coli | 0.25 μg/mL | Membrane permeability disruption |
Pseudomonas aeruginosa | 0.50 μg/mL | Induction of oxidative stress |
Antiparasitic Activity
This compound has also shown promising results in combating malaria, specifically against Plasmodium falciparum strains. The compound's antiplasmodial activity was evaluated through IC50 values, indicating its effectiveness in inhibiting parasite growth.
Table 2: Antiplasmodial Activity of this compound Against Plasmodium falciparum
Strain | IC50 (μg/mL) | Comparison with Chloroquine (CQ) |
---|---|---|
3D7 (Chloroquine-sensitive) | 0.0049 | CQ: 0.06 |
FCR-3 (Chloroquine-resistant) | 0.12 | CQ: >1.0 |
The data indicates that this compound is significantly more effective than chloroquine against the chloroquine-sensitive strain and retains substantial activity against the resistant strain .
The precise mechanisms underlying the biological activity of this compound are not fully elucidated; however, several hypotheses have emerged:
- Oxidative Stress Induction : It is believed that this compound induces oxidative stress in target cells, leading to cellular damage and death.
- Membrane Disruption : The compound may disrupt cellular membranes, compromising the integrity of bacterial and parasitic cells .
- Targeting Key Enzymes : Docking studies suggest that this compound interacts with dihydroorotate dehydrogenase (DHODH), an enzyme critical for pyrimidine biosynthesis in Plasmodium falciparum .
Case Studies and Research Findings
Several studies have documented the efficacy of this compound in laboratory settings:
- Antibacterial Efficacy : In vitro studies demonstrated that this compound significantly inhibited the growth of multidrug-resistant Staphylococcus aureus strains.
- Antimalarial Potential : Comparative studies indicated that this compound's antiplasmodial activity was over 250-fold more potent than previously reported naphthoquinone derivatives .
Q & A
Basic Research Questions
Q. What are the optimal synthetic pathways for Phenisatin, and how can reaction conditions be systematically optimized?
- Methodology : Begin with established protocols (e.g., condensation reactions or catalytic cyclization) and vary parameters such as temperature, solvent polarity, and catalyst loading. Use design-of-experiment (DoE) frameworks to identify critical factors affecting yield . Monitor reaction progress via thin-layer chromatography (TLC) and characterize intermediates using NMR and mass spectrometry. Reproducibility requires detailed documentation of stoichiometry, purification steps, and yield calculations .
Q. Which analytical techniques are most reliable for verifying this compound’s purity and structural identity?
- Methodology : Combine high-performance liquid chromatography (HPLC) with UV detection (≥95% purity threshold) and nuclear magnetic resonance (NMR) spectroscopy for structural confirmation. Cross-validate results with Fourier-transform infrared spectroscopy (FTIR) and elemental analysis. For novel derivatives, X-ray crystallography provides definitive structural evidence .
Q. How should researchers design in vitro assays to evaluate this compound’s bioactivity?
- Methodology : Use cell-based assays (e.g., enzyme inhibition or receptor-binding studies) with positive and negative controls. Specify concentrations (e.g., IC50/EC50) and replicate experiments to account for biological variability. Adopt standardized protocols from journals like Medicinal Chemistry Research to ensure comparability .
Q. What are the best practices for ensuring this compound’s stability during storage and experimentation?
- Methodology : Conduct accelerated stability studies under varying temperatures, humidity, and light exposure. Monitor degradation via HPLC and mass spectrometry. Store samples in airtight, light-resistant containers at -20°C, and avoid repeated freeze-thaw cycles .
Q. How can researchers validate the reproducibility of this compound synthesis across laboratories?
- Methodology : Share detailed experimental protocols (e.g., reagent sources, equipment calibration data) and raw spectral datasets as supplementary information. Collaborate with independent labs to replicate key steps, emphasizing adherence to IUPAC nomenclature and reporting standards .
Advanced Research Questions
Q. How can contradictions in spectroscopic data for this compound derivatives be resolved?
- Methodology : Apply multivariate statistical analysis (e.g., principal component analysis) to identify outliers or systematic errors. Cross-reference data with computational predictions (e.g., density functional theory for NMR chemical shifts) and consult crystallographic databases for structural analogs .
Q. What computational models best predict this compound’s pharmacokinetic properties, and how do they align with empirical data?
- Methodology : Use molecular dynamics simulations to assess solubility and membrane permeability. Validate predictions against in vivo pharmacokinetic studies (e.g., bioavailability in rodent models). Discrepancies may arise from oversimplified force fields, necessitating iterative model refinement .
Q. How can mechanistic studies elucidate this compound’s mode of action at the molecular level?
- Methodology : Employ isotopic labeling (e.g., ¹⁴C or ³H) to track metabolic pathways. Pair kinetic studies with stopped-flow spectroscopy to resolve transient intermediates. For enzyme targets, use site-directed mutagenesis to identify binding residues .
Q. What statistical approaches are recommended for analyzing variability in this compound’s pharmacological data?
- Methodology : Apply ANOVA or mixed-effects models to distinguish biological variability from technical noise. Use Bayesian frameworks to quantify uncertainty in dose-response relationships. Report confidence intervals and effect sizes to enhance interpretability .
Q. How can researchers leverage high-throughput screening (HTS) to identify synergistic effects of this compound with other compounds?
- Methodology : Design combinatorial libraries using factorial arrays and assess synergy via the Chou-Talalay method. Validate hits with isobologram analysis and transcriptomic profiling. Ensure HTS protocols include robotic liquid handling and automated data capture to minimize human error .
Q. Key Considerations for Methodological Rigor
- Data Contradictions : Address conflicting results by re-examining experimental conditions (e.g., buffer pH, solvent purity) and applying hypothesis-testing frameworks like FINER (Feasible, Interesting, Novel, Ethical, Relevant) .
- Ethical Reporting : Avoid data manipulation; clearly distinguish observed results from theoretical interpretations .
- Interdisciplinary Collaboration : Engage computational chemists, pharmacologists, and statisticians to strengthen study design and data interpretation .
Eigenschaften
IUPAC Name |
[4-[1-acetyl-3-(4-acetyloxyphenyl)-2-oxoindol-3-yl]phenyl] acetate | |
---|---|---|
Source | PubChem | |
URL | https://pubchem.ncbi.nlm.nih.gov | |
Description | Data deposited in or computed by PubChem | |
InChI |
InChI=1S/C26H21NO6/c1-16(28)27-24-7-5-4-6-23(24)26(25(27)31,19-8-12-21(13-9-19)32-17(2)29)20-10-14-22(15-11-20)33-18(3)30/h4-15H,1-3H3 | |
Source | PubChem | |
URL | https://pubchem.ncbi.nlm.nih.gov | |
Description | Data deposited in or computed by PubChem | |
InChI Key |
JPGUEMQIAGJQSJ-UHFFFAOYSA-N | |
Source | PubChem | |
URL | https://pubchem.ncbi.nlm.nih.gov | |
Description | Data deposited in or computed by PubChem | |
Canonical SMILES |
CC(=O)N1C2=CC=CC=C2C(C1=O)(C3=CC=C(C=C3)OC(=O)C)C4=CC=C(C=C4)OC(=O)C | |
Source | PubChem | |
URL | https://pubchem.ncbi.nlm.nih.gov | |
Description | Data deposited in or computed by PubChem | |
Molecular Formula |
C26H21NO6 | |
Source | PubChem | |
URL | https://pubchem.ncbi.nlm.nih.gov | |
Description | Data deposited in or computed by PubChem | |
DSSTOX Substance ID |
DTXSID2066434 | |
Record name | 2H-Indol-2-one, 1-acetyl-3,3-bis[4-(acetyloxy)phenyl]-1,3-dihydro- | |
Source | EPA DSSTox | |
URL | https://comptox.epa.gov/dashboard/DTXSID2066434 | |
Description | DSSTox provides a high quality public chemistry resource for supporting improved predictive toxicology. | |
Molecular Weight |
443.4 g/mol | |
Source | PubChem | |
URL | https://pubchem.ncbi.nlm.nih.gov | |
Description | Data deposited in or computed by PubChem | |
CAS No. |
18869-73-3 | |
Record name | 1-Acetyl-3,3-bis[4-(acetyloxy)phenyl]-1,3-dihydro-2H-indol-2-one | |
Source | CAS Common Chemistry | |
URL | https://commonchemistry.cas.org/detail?cas_rn=18869-73-3 | |
Description | CAS Common Chemistry is an open community resource for accessing chemical information. Nearly 500,000 chemical substances from CAS REGISTRY cover areas of community interest, including common and frequently regulated chemicals, and those relevant to high school and undergraduate chemistry classes. This chemical information, curated by our expert scientists, is provided in alignment with our mission as a division of the American Chemical Society. | |
Explanation | The data from CAS Common Chemistry is provided under a CC-BY-NC 4.0 license, unless otherwise stated. | |
Record name | Laxagetten 4,4'-diacetoxydiphenylpyridylemethane | |
Source | ChemIDplus | |
URL | https://pubchem.ncbi.nlm.nih.gov/substance/?source=chemidplus&sourceid=0018869733 | |
Description | ChemIDplus is a free, web search system that provides access to the structure and nomenclature authority files used for the identification of chemical substances cited in National Library of Medicine (NLM) databases, including the TOXNET system. | |
Record name | 2H-Indol-2-one, 1-acetyl-3,3-bis[4-(acetyloxy)phenyl]-1,3-dihydro- | |
Source | EPA Chemicals under the TSCA | |
URL | https://www.epa.gov/chemicals-under-tsca | |
Description | EPA Chemicals under the Toxic Substances Control Act (TSCA) collection contains information on chemicals and their regulations under TSCA, including non-confidential content from the TSCA Chemical Substance Inventory and Chemical Data Reporting. | |
Record name | 2H-Indol-2-one, 1-acetyl-3,3-bis[4-(acetyloxy)phenyl]-1,3-dihydro- | |
Source | EPA DSSTox | |
URL | https://comptox.epa.gov/dashboard/DTXSID2066434 | |
Description | DSSTox provides a high quality public chemistry resource for supporting improved predictive toxicology. | |
Record name | 4,4'-(1-acetyl-2-oxoindolin-3-ylidene)diphenyl di(acetate) | |
Source | European Chemicals Agency (ECHA) | |
URL | https://echa.europa.eu/substance-information/-/substanceinfo/100.038.747 | |
Description | The European Chemicals Agency (ECHA) is an agency of the European Union which is the driving force among regulatory authorities in implementing the EU's groundbreaking chemicals legislation for the benefit of human health and the environment as well as for innovation and competitiveness. | |
Explanation | Use of the information, documents and data from the ECHA website is subject to the terms and conditions of this Legal Notice, and subject to other binding limitations provided for under applicable law, the information, documents and data made available on the ECHA website may be reproduced, distributed and/or used, totally or in part, for non-commercial purposes provided that ECHA is acknowledged as the source: "Source: European Chemicals Agency, http://echa.europa.eu/". Such acknowledgement must be included in each copy of the material. ECHA permits and encourages organisations and individuals to create links to the ECHA website under the following cumulative conditions: Links can only be made to webpages that provide a link to the Legal Notice page. | |
Record name | PHENISATIN | |
Source | FDA Global Substance Registration System (GSRS) | |
URL | https://gsrs.ncats.nih.gov/ginas/app/beta/substances/ZHJ4JHL6HB | |
Description | The FDA Global Substance Registration System (GSRS) enables the efficient and accurate exchange of information on what substances are in regulated products. Instead of relying on names, which vary across regulatory domains, countries, and regions, the GSRS knowledge base makes it possible for substances to be defined by standardized, scientific descriptions. | |
Explanation | Unless otherwise noted, the contents of the FDA website (www.fda.gov), both text and graphics, are not copyrighted. They are in the public domain and may be republished, reprinted and otherwise used freely by anyone without the need to obtain permission from FDA. Credit to the U.S. Food and Drug Administration as the source is appreciated but not required. | |
Retrosynthesis Analysis
AI-Powered Synthesis Planning: Our tool employs the Template_relevance Pistachio, Template_relevance Bkms_metabolic, Template_relevance Pistachio_ringbreaker, Template_relevance Reaxys, Template_relevance Reaxys_biocatalysis model, leveraging a vast database of chemical reactions to predict feasible synthetic routes.
One-Step Synthesis Focus: Specifically designed for one-step synthesis, it provides concise and direct routes for your target compounds, streamlining the synthesis process.
Accurate Predictions: Utilizing the extensive PISTACHIO, BKMS_METABOLIC, PISTACHIO_RINGBREAKER, REAXYS, REAXYS_BIOCATALYSIS database, our tool offers high-accuracy predictions, reflecting the latest in chemical research and data.
Strategy Settings
Precursor scoring | Relevance Heuristic |
---|---|
Min. plausibility | 0.01 |
Model | Template_relevance |
Template Set | Pistachio/Bkms_metabolic/Pistachio_ringbreaker/Reaxys/Reaxys_biocatalysis |
Top-N result to add to graph | 6 |
Feasible Synthetic Routes
Haftungsausschluss und Informationen zu In-Vitro-Forschungsprodukten
Bitte beachten Sie, dass alle Artikel und Produktinformationen, die auf BenchChem präsentiert werden, ausschließlich zu Informationszwecken bestimmt sind. Die auf BenchChem zum Kauf angebotenen Produkte sind speziell für In-vitro-Studien konzipiert, die außerhalb lebender Organismen durchgeführt werden. In-vitro-Studien, abgeleitet von dem lateinischen Begriff "in Glas", beinhalten Experimente, die in kontrollierten Laborumgebungen unter Verwendung von Zellen oder Geweben durchgeführt werden. Es ist wichtig zu beachten, dass diese Produkte nicht als Arzneimittel oder Medikamente eingestuft sind und keine Zulassung der FDA für die Vorbeugung, Behandlung oder Heilung von medizinischen Zuständen, Beschwerden oder Krankheiten erhalten haben. Wir müssen betonen, dass jede Form der körperlichen Einführung dieser Produkte in Menschen oder Tiere gesetzlich strikt untersagt ist. Es ist unerlässlich, sich an diese Richtlinien zu halten, um die Einhaltung rechtlicher und ethischer Standards in Forschung und Experiment zu gewährleisten.