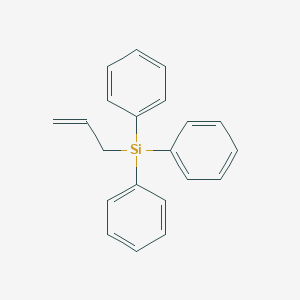
Allyltriphenylsilane
Übersicht
Beschreibung
Allyltriphenylsilane (CAS: 18752-21-1, molecular formula: C₂₁H₂₀Si, molecular weight: 300.47 g/mol) is an organosilicon compound featuring a silicon atom bonded to three phenyl groups and an allyl group . Its bulky triphenyl substituents confer unique steric and electronic properties, making it a versatile reagent in organic synthesis. Key applications include fluorohydrin synthesis , diene preparation via Peterson elimination , and participation in silyl-Prins cyclizations . Unlike smaller allylsilanes (e.g., allyltrimethylsilane), its reactivity is often moderated by steric hindrance, which can influence regioselectivity and reaction rates .
Vorbereitungsmethoden
Grignard Reagent-Mediated Synthesis
Reaction Mechanism and General Procedure
The Grignard method involves the reaction of allyl magnesium halides (generated in situ) with chlorotriphenylsilane (Ph₃SiCl). This one-pot, two-step process proceeds via nucleophilic substitution, where the allyl Grignard reagent displaces the chloride ligand on the silicon center . The general reaction is summarized as:
3\text{Si-Cl} \rightarrow \text{Allyl-Si-Ph}3 + \text{Mg-X-Cl}
Key Steps :
-
Formation of the Grignard Reagent : Allyl halides (e.g., allyl chloride or bromide) react with magnesium metal in a mixed ether-hydrocarbon solvent (e.g., diethyl ether/toluene).
-
Nucleophilic Substitution : The allyl Grignard reagent reacts with chlorotriphenylsilane under reflux, yielding Allyltriphenylsilane.
Optimization of Reaction Conditions
Critical parameters influencing yield and selectivity include solvent composition, temperature, and stoichiometry.
Solvent System
A mixed solvent of ethers (e.g., THF, diethyl ether) and hydrocarbons (e.g., toluene, xylene) in a molar ratio of 0.05–4:1 reduces flammability risks while maintaining reaction efficiency . For example, a 1:1 ether/toluene mixture enables safer handling compared to pure ether.
Temperature and Time
-
Grignard Formation : Initiated at 25–40°C, followed by reflux (70–100°C) for 24–70 hours .
-
Quenching : Performed with saturated ammonium chloride to hydrolyze unreacted Grignard reagent.
Workup and Isolation
-
Neutralization : Adjust pH to neutral to prevent siloxane formation.
-
Distillation : Under reduced pressure (e.g., 0.1–1 mmHg) to isolate this compound as a colorless liquid .
Performance Data
The table below summarizes optimized conditions derived from patent literature :
Parameter | Optimal Range | Impact on Yield |
---|---|---|
Solvent (ether:hydrocarbon) | 1:1 (v/v) | Maximizes reagent solubility |
Temperature | 70°C (reflux) | Completes reaction in 48h |
Mg:Allyl Halide Ratio | 1:1.1 | Minimizes side reactions |
Silane Stoichiometry | 1.05 equiv | Ensures complete conversion |
Comparative Analysis of Allyl Halide Substrates
The choice of allyl halide (X = Cl, Br) significantly affects reaction kinetics:
-
Allyl Bromide : Higher reactivity due to better leaving-group ability, but requires stringent moisture control.
-
Allyl Chloride : Cost-effective but necessitates prolonged reaction times .
Yield Comparison :
Recent Advances and Applications
Role in Catalytic Isomerization
This compound serves as a substrate in B(C₆F₅)₃-catalyzed isomerization to form E-alkenes, achieving 97:3 E:Z selectivity . This application underscores the compound’s utility in stereoselective synthesis.
Hiyama Coupling
Pd-catalyzed coupling of this compound with aryl iodides enables access to functionalized styrenes (e.g., 16–31 in Scheme 3B of Ref ), though this pertains to downstream applications rather than preparation.
Challenges and Limitations
-
Moisture Sensitivity : Requires anhydrous conditions throughout synthesis.
-
Silane Purity : Trace impurities (e.g., siloxanes) necessitate rigorous distillation.
Analyse Chemischer Reaktionen
Types of Reactions: Allyltriphenylsilane undergoes several types of chemical reactions, including:
Electrophilic Substitution: The compound can participate in electrophilic substitution reactions, where an electrophile attacks the allyl group, leading to the formation of new carbon-carbon bonds.
Oxidation and Reduction: this compound can be oxidized or reduced under specific conditions, although these reactions are less common compared to electrophilic substitutions.
Common Reagents and Conditions:
Electrophilic Substitution: Common reagents include strong acids like hydrobromic acid (HBr) or hydroiodic acid (HI), which facilitate the substitution process.
Oxidation and Reduction: These reactions may involve oxidizing agents like potassium permanganate (KMnO4) or reducing agents such as lithium aluminum hydride (LiAlH4).
Major Products:
Wissenschaftliche Forschungsanwendungen
Organic Synthesis
Allyltriphenylsilane is primarily recognized for its role as a reagent in organic synthesis. It facilitates the formation of carbon-carbon bonds, particularly in the synthesis of 1,3-dienes and other complex organic molecules. This capability is crucial for constructing various chemical architectures essential in pharmaceuticals and fine chemicals.
Key Reactions:
- Formation of 1,3-Dienes: this compound serves as a key reagent in stereoselective syntheses, allowing for the efficient construction of 1,3-dienes that are valuable intermediates in organic synthesis .
- Electrophilic Substitution: The compound exhibits significant reactivity with electrophiles, enabling the formation of new carbon-carbon bonds selectively at the γ-position. This selectivity is influenced by the steric and electronic properties of the triphenyl moiety .
Catalysis
In catalysis, this compound has been employed in several processes due to its ability to stabilize reactive intermediates and enhance reaction rates.
Examples of Catalytic Applications:
- Isomerization Reactions: Research has demonstrated that this compound can be used effectively in isomerization reactions under mild conditions, showcasing its potential as a catalyst for transforming alkenes .
- Fluorohydrin Synthesis: The compound has been utilized in synthesizing 2-fluoro-3-silylpropan-1-ols through epoxidation followed by treatment with triethylamine trihydrofluoride (HF·Et₃N), achieving high yields and regioselectivity .
Biological and Medicinal Applications
This compound's reactivity extends into biological chemistry, where it has been explored for applications in drug development and synthesis.
Potential Biological Activities:
- Antimicrobial Properties: Studies indicate that derivatives of this compound may exhibit antimicrobial activities, making them candidates for further exploration in medicinal chemistry .
- Pharmaceutical Intermediates: Its ability to form complex organic structures positions this compound as a valuable intermediate in the synthesis of pharmaceutical agents .
Comparison with Related Compounds
This compound can be compared with other silanes to highlight its unique properties:
Compound Name | Structure Type | Unique Features |
---|---|---|
Allyltrimethylsilane | Trimethylsilyl group | More reactive due to smaller substituents |
Vinyltrimethylsilane | Vinyl group | Different reactivity patterns compared to allyls |
Allyldimethylphenylsilane | Dimethylphenyl group | Offers different steric effects and reactivity |
Triphenylsilane | Triphenyl group | Lacks unsaturation, affecting reactivity |
This compound's combination of bulky phenyl groups and an allylic double bond provides distinct reactivity patterns compared to its analogs, particularly in electrophilic substitution reactions .
Wirkmechanismus
The mechanism of action for allyltriphenylsilane primarily involves its role as a nucleophile in electrophilic substitution reactions. The silicon atom’s electron-donating properties stabilize the transition state, facilitating the formation of new carbon-carbon bonds. The molecular targets and pathways involved are largely dictated by the specific electrophile and reaction conditions .
Vergleich Mit ähnlichen Verbindungen
Comparison with Similar Allylsilanes and Organometallic Reagents
Chemical and Structural Properties
The table below summarizes structural and physicochemical differences between allyltriphenylsilane and analogous compounds:
Compound | Molecular Formula | Molecular Weight (g/mol) | Substituents on Si | Key Structural Features |
---|---|---|---|---|
This compound | C₂₁H₂₀Si | 300.47 | 3 Ph, 1 allyl | High steric bulk, aromatic stabilization |
Allyltrimethylsilane | C₆H₁₄Si | 114.27 | 3 Me, 1 allyl | Low steric hindrance, higher volatility |
Allyltributylstannane | C₁₅H₃₂Sn | 347.13 | 3 Bu, 1 allyl | Tin center, higher toxicity |
Triphenylgermane | C₁₈H₁₅Ge | 324.50 | 3 Ph, 1 H | Germanium analog, similar bulk |
Key Observations :
- Steric Effects : this compound’s bulky triphenyl groups hinder nucleophilic attack at silicon, slowing reactions like Shi epoxidation compared to allyltrimethylsilane .
- Electronic Effects : The phenyl groups stabilize the silicon center through σ–π conjugation, enhancing thermal stability .
a) Silyl-Prins Cyclization
In diastereoselective cyclizations to form tetrahydrofurans (THFs) and tetrahydropyrans (THPs), this compound produces complex mixtures due to competing pathways, whereas allyltrimethylsilane yields separable products (60% combined yield of THP and DHP) . For example:
- This compound : Complex mixtures, low selectivity.
- Allyltrimethylsilane : THP22b + DHP23 (60% yield) .
c) Epoxidation
In Shi epoxidation, this compound reacts slower than allyltrimethylsilane due to steric hindrance. However, extended reaction times (16–72 h) achieve comparable yields (61:39 enantiomeric ratio) .
Advantages and Limitations
This compound
- Pros : High thermal stability, regioselectivity in fluorination, and compatibility with transition-metal catalysts.
- Cons : Slow reaction kinetics in sterically demanding processes; complex mixtures in cyclizations.
Allyltrimethylsilane
- Pros : Faster reaction rates, cost-effective.
- Cons : Lower selectivity due to reduced steric control.
Allyltributylstannane
- Pros : High reactivity in Stille couplings.
- Cons : Toxicity and environmental concerns.
Biologische Aktivität
Allyltriphenylsilane (ATPS), an organosilicon compound with the formula C₁₈H₁₈Si, features a silicon atom bonded to three phenyl groups and an allyl group. This unique structure contributes to its diverse reactivity and potential biological applications. This article reviews the biological activity of ATPS, focusing on its synthesis, mechanisms of action, and relevant case studies.
Synthesis and Reactivity
This compound can be synthesized through various methods, often involving palladium-catalyzed reactions that generate allyl silanes from alkenes. For instance, the silyl-Heck reaction allows for the formation of ATPS from terminal alkenes under mild conditions, yielding high selectivity and efficiency . The compound's reactivity is characterized by its ability to form new carbon-carbon bonds and interact selectively with electrophiles and nucleophiles, making it a valuable reagent in organic synthesis.
Biological Activity Overview
The biological activity of ATPS is primarily linked to its structural motif, which is common among many bioactive compounds. The allyl group is known for its role in various natural products with significant pharmacological properties, including anticancer activities . Below are some key findings regarding the biological activity of ATPS:
Case Study 1: Anticancer Activity
A study reviewed several allyl derivatives' effects on cancer cell lines. While specific data on ATPS was not highlighted, related compounds demonstrated significant cytotoxicity against breast cancer cells. The presence of the allyl group was crucial for this activity, indicating that ATPS may share similar properties .
Case Study 2: Synthesis of Bioactive Compounds
Research has shown that ATPS can be utilized in synthesizing bioactive molecules through various reactions. For instance, it has been employed in regioselective transformations leading to fluorohydrins, which are precursors for several pharmaceuticals . The efficiency of these reactions underscores the potential of ATPS as a building block in drug development.
Comparative Analysis with Related Compounds
The following table summarizes the characteristics of ATPS compared to other related silanes:
Compound Name | Structure Type | Unique Features |
---|---|---|
Allyltrimethylsilane | Trimethylsilyl group | More reactive due to smaller substituents |
Vinyltrimethylsilane | Vinyl group | Different reactivity patterns compared to allyls |
Allyldimethylphenylsilane | Dimethylphenyl group | Offers different steric effects and reactivity |
Triphenylsilane | Triphenyl group | Lacks unsaturation, affecting reactivity |
This compound | Triphenyl + Allyl | Unique combination enhances selectivity |
Q & A
Q. Basic: What synthetic methods are used to prepare Allyltriphenylsilane, and how can its purity be validated for research use?
Answer:
this compound is typically synthesized via nucleophilic substitution between triphenylsilane and allyl halides (e.g., allyl chloride) in anhydrous conditions. A common protocol involves reacting allyl magnesium bromide (Grignard reagent) with triphenylchlorosilane in tetrahydrofuran (THF) under inert atmosphere . Post-synthesis, purification is achieved via column chromatography using hexane/ethyl acetate gradients. Purity validation requires 1H/13C NMR to confirm molecular structure (e.g., allylic proton signals at δ 4.8–5.2 ppm and aromatic protons at δ 7.2–7.6 ppm) and gas chromatography (GC) or HPLC to quantify impurities (<1%) . For new batches, elemental analysis (C, H, Si) is recommended to verify stoichiometric consistency.
Q. Advanced: How does the steric and electronic profile of this compound influence its reactivity in electrophilic addition reactions compared to other allylsilanes?
Answer:
The triphenylsilyl group imposes significant steric hindrance, reducing reaction rates in crowded transition states. For example, in carbocation trapping experiments (e.g., with benzhydryl cations), this compound exhibits ~30% slower kinetics compared to allyltrimethylsilane due to steric shielding of the allyl group . However, the electron-withdrawing nature of phenyl groups stabilizes the β-silyl carbocation intermediate, favoring regioselective allylation in polar solvents like CH2Cl2. Researchers should optimize equivalents (5–10 equiv.) and reaction temperature (−70°C to 0°C) to balance steric and electronic effects . Kinetic studies using UV-Vis spectroscopy or stopped-flow techniques are critical for quantifying these effects .
Q. Basic: What safety protocols are essential when handling this compound in laboratory settings?
Answer:
this compound is moisture-sensitive and may release flammable gases (e.g., hydrogen) upon contact with water. Key safety measures include:
- Personal protective equipment (PPE): Nitrile gloves, lab coat, and safety goggles.
- Handling: Use in a fume hood under nitrogen/argon atmosphere to prevent hydrolysis.
- Storage: In airtight containers with desiccants, away from oxidizers and humidity .
- Spill management: Neutralize with dry sand or vermiculite; avoid aqueous solutions to prevent exothermic reactions . Toxicity data (e.g., LD50) should be reviewed prior to use, though limited ecotoxicological studies necessitate cautious waste disposal via licensed chemical waste services .
Q. Advanced: How can researchers resolve contradictory data on this compound’s stereoselectivity in multicomponent cascade reactions?
Answer:
Contradictions often arise from variable reaction conditions (e.g., solvent polarity, catalyst loading) or competing pathways. For example, in tetrahydrofuran synthesis, this compound may yield complex mixtures due to kinetic vs. thermodynamic control . To resolve this:
- Experimental design: Use Design of Experiments (DoE) to systematically vary parameters (temperature, solvent, stoichiometry).
- Analytical tools: Employ chiral HPLC or GC-MS with enantiomeric columns to distinguish diastereomers.
- Mechanistic probes: Isotopic labeling (e.g., deuterated substrates) or in-situ IR spectroscopy can track intermediate formation .
Cross-validation with computational models (DFT calculations for transition state energies) is advised to reconcile experimental and theoretical data .
Q. Basic: What solvent systems and catalytic conditions optimize this compound’s performance in allylation reactions?
Answer:
Nonpolar solvents (e.g., CH2Cl2, toluene) enhance electrophilic allylation by stabilizing carbocation intermediates. For Lewis acid-catalyzed reactions (e.g., with TiCl4 or GaCl3), maintain low temperatures (−70°C to −20°C) to suppress side reactions like polymerization . Catalyst loading (5–10 mol%) and slow reagent addition (syringe pump) improve yield in stoichiometrically sensitive reactions. Pre-drying solvents over molecular sieves (3Å) is critical to avoid silane hydrolysis .
Q. Advanced: How can computational chemistry aid in predicting this compound’s regioselectivity in organocatalytic cycles?
Answer:
Density Functional Theory (DFT) simulations (e.g., B3LYP/6-31G*) can model transition states to predict regioselectivity. Key steps include:
Geometry optimization of this compound and electrophilic partners.
NBO (Natural Bond Orbital) analysis to quantify orbital interactions (e.g., σ→π* hyperconjugation).
Kinetic profiling using Eyring equations to compare activation barriers for competing pathways .
Experimental validation via laser flash photolysis (to measure carbocation lifetimes) or kinetic isotope effects (KIE) is recommended to confirm computational predictions .
Eigenschaften
IUPAC Name |
triphenyl(prop-2-enyl)silane | |
---|---|---|
Source | PubChem | |
URL | https://pubchem.ncbi.nlm.nih.gov | |
Description | Data deposited in or computed by PubChem | |
InChI |
InChI=1S/C21H20Si/c1-2-18-22(19-12-6-3-7-13-19,20-14-8-4-9-15-20)21-16-10-5-11-17-21/h2-17H,1,18H2 | |
Source | PubChem | |
URL | https://pubchem.ncbi.nlm.nih.gov | |
Description | Data deposited in or computed by PubChem | |
InChI Key |
DXJZZRSMGLGFPW-UHFFFAOYSA-N | |
Source | PubChem | |
URL | https://pubchem.ncbi.nlm.nih.gov | |
Description | Data deposited in or computed by PubChem | |
Canonical SMILES |
C=CC[Si](C1=CC=CC=C1)(C2=CC=CC=C2)C3=CC=CC=C3 | |
Source | PubChem | |
URL | https://pubchem.ncbi.nlm.nih.gov | |
Description | Data deposited in or computed by PubChem | |
Molecular Formula |
C21H20Si | |
Source | PubChem | |
URL | https://pubchem.ncbi.nlm.nih.gov | |
Description | Data deposited in or computed by PubChem | |
DSSTOX Substance ID |
DTXSID80299137 | |
Record name | Allyltriphenylsilane | |
Source | EPA DSSTox | |
URL | https://comptox.epa.gov/dashboard/DTXSID80299137 | |
Description | DSSTox provides a high quality public chemistry resource for supporting improved predictive toxicology. | |
Molecular Weight |
300.5 g/mol | |
Source | PubChem | |
URL | https://pubchem.ncbi.nlm.nih.gov | |
Description | Data deposited in or computed by PubChem | |
CAS No. |
18752-21-1 | |
Record name | 18752-21-1 | |
Source | DTP/NCI | |
URL | https://dtp.cancer.gov/dtpstandard/servlet/dwindex?searchtype=NSC&outputformat=html&searchlist=128375 | |
Description | The NCI Development Therapeutics Program (DTP) provides services and resources to the academic and private-sector research communities worldwide to facilitate the discovery and development of new cancer therapeutic agents. | |
Explanation | Unless otherwise indicated, all text within NCI products is free of copyright and may be reused without our permission. Credit the National Cancer Institute as the source. | |
Record name | Allyltriphenylsilane | |
Source | EPA DSSTox | |
URL | https://comptox.epa.gov/dashboard/DTXSID80299137 | |
Description | DSSTox provides a high quality public chemistry resource for supporting improved predictive toxicology. | |
Retrosynthesis Analysis
AI-Powered Synthesis Planning: Our tool employs the Template_relevance Pistachio, Template_relevance Bkms_metabolic, Template_relevance Pistachio_ringbreaker, Template_relevance Reaxys, Template_relevance Reaxys_biocatalysis model, leveraging a vast database of chemical reactions to predict feasible synthetic routes.
One-Step Synthesis Focus: Specifically designed for one-step synthesis, it provides concise and direct routes for your target compounds, streamlining the synthesis process.
Accurate Predictions: Utilizing the extensive PISTACHIO, BKMS_METABOLIC, PISTACHIO_RINGBREAKER, REAXYS, REAXYS_BIOCATALYSIS database, our tool offers high-accuracy predictions, reflecting the latest in chemical research and data.
Strategy Settings
Precursor scoring | Relevance Heuristic |
---|---|
Min. plausibility | 0.01 |
Model | Template_relevance |
Template Set | Pistachio/Bkms_metabolic/Pistachio_ringbreaker/Reaxys/Reaxys_biocatalysis |
Top-N result to add to graph | 6 |
Feasible Synthetic Routes
Haftungsausschluss und Informationen zu In-Vitro-Forschungsprodukten
Bitte beachten Sie, dass alle Artikel und Produktinformationen, die auf BenchChem präsentiert werden, ausschließlich zu Informationszwecken bestimmt sind. Die auf BenchChem zum Kauf angebotenen Produkte sind speziell für In-vitro-Studien konzipiert, die außerhalb lebender Organismen durchgeführt werden. In-vitro-Studien, abgeleitet von dem lateinischen Begriff "in Glas", beinhalten Experimente, die in kontrollierten Laborumgebungen unter Verwendung von Zellen oder Geweben durchgeführt werden. Es ist wichtig zu beachten, dass diese Produkte nicht als Arzneimittel oder Medikamente eingestuft sind und keine Zulassung der FDA für die Vorbeugung, Behandlung oder Heilung von medizinischen Zuständen, Beschwerden oder Krankheiten erhalten haben. Wir müssen betonen, dass jede Form der körperlichen Einführung dieser Produkte in Menschen oder Tiere gesetzlich strikt untersagt ist. Es ist unerlässlich, sich an diese Richtlinien zu halten, um die Einhaltung rechtlicher und ethischer Standards in Forschung und Experiment zu gewährleisten.