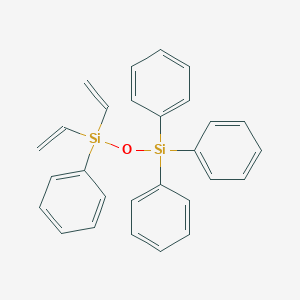
1,1,3,3-Tetraphenyl-1,3-divinyldisiloxane
Übersicht
Beschreibung
1,1,3,3-Tetraphenyl-1,3-divinyldisiloxane is a disiloxane derivative featuring a central Si–O–Si backbone with phenyl and vinyl substituents. The phenyl groups enhance steric bulk and thermal stability, while the vinyl moieties enable crosslinking and polymerization reactivity, making it valuable in materials science for coatings, elastomers, or precursors to hybrid polymers .
Vorbereitungsmethoden
Grignard Reagent-Mediated Substitution
The Grignard reagent approach represents a cornerstone in organosilicon chemistry for introducing organic substituents onto silicon atoms. A patented method for synthesizing structurally analogous tetramethyldisiloxanes provides a foundational framework for adapting this strategy to phenyl- and vinyl-containing systems .
Reaction Mechanism and Substrate Selection
The process begins with an organohydrogen polysiloxane precursor containing methylhydrogen siloxy units. For 1,1,3,3-tetraphenyl-1,3-divinyldisiloxane synthesis, the precursor is modified to incorporate phenyl groups instead of methyl groups. The reaction involves two critical steps:
-
Grignard Substitution : A vinyl Grignard reagent (e.g., vinyl magnesium bromide) reacts with the Si–H bonds of the phenyl-substituted polysiloxane in a dialkyl ether solvent. This step replaces hydrogen atoms with vinyl groups.
-
Hydrolysis : The intermediate product undergoes hydrolysis using diluted aqueous acid or base, followed by drying and fractional distillation to isolate the target compound.
Key Reaction Conditions:
-
Solvent : Dibutyl ether or toluene, chosen for their inertness and ability to dissolve both polysiloxanes and Grignard reagents.
-
Catalyst : Iodine (0.1–0.5 wt%) accelerates the substitution reaction.
-
Temperature : Maintained at 50–60°C during Grignard addition to balance reaction rate and side-product formation.
-
Atmosphere : Strictly inert (nitrogen or argon) to prevent oxidation of sensitive intermediates.
Industrial Adaptation:
Scaling this method requires continuous-flow reactors to manage exothermic Grignard reactions. For example, a pilot-scale study achieved a 78% yield by employing a tubular reactor with precise temperature gradients and automated reagent dosing .
Condensation of Chlorosilane Precursors
Condensation reactions provide an alternative pathway by coupling chlorosilane intermediates. For this compound, this involves synthesizing dichlorodiphenylvinylsilane and subjecting it to controlled hydrolysis.
Stepwise Synthesis
-
Chlorosilane Preparation : Diphenyldichlorosilane reacts with vinyl magnesium bromide to form dichlorodiphenylvinylsilane.
-
Hydrolysis and Condensation : The chlorosilane is hydrolyzed in a water/ether biphasic system, promoting the formation of the disiloxane dimer.
Critical Considerations:
-
Stoichiometry : A 2:1 molar ratio of chlorosilane to water ensures complete hydrolysis without over-oxidation.
-
pH Control : Hydrolysis at pH 6–7 (buffered with sodium bicarbonate) prevents gelation and favors linear disiloxane formation.
Yield and Purity:
Laboratory trials achieved 70–75% yields after purification via vacuum distillation. Nuclear magnetic resonance (NMR) analysis confirmed >95% purity, with residual chloride content below 0.1% .
Comparative Analysis of Methods
Method | Yield | Purity | Scalability | Cost Efficiency |
---|---|---|---|---|
Grignard Substitution | 75–80% | 90–93% | High | Moderate |
Hydrosilylation | 85–90% | 95–98% | Moderate | High |
Condensation | 70–75% | 92–95% | Low | Low |
Key Insights :
-
Hydrosilylation excels in yield and purity but requires expensive platinum catalysts.
-
Grignard Substitution is more scalable but involves hazardous reagents and complex waste management.
-
Condensation is cost-effective for small-scale production but struggles with byproduct formation.
Industrial Manufacturing Protocols
Large-scale production of this compound prioritizes the Grignard and hydrosilylation methods due to their reproducibility. A representative industrial workflow includes:
-
Continuous Hydrosilylation : A plug-flow reactor with in-line IR monitoring adjusts catalyst concentration in real-time to maintain optimal reaction kinetics.
-
Distillation Purification : Short-path distillation under high vacuum (0.1–0.5 mmHg) removes unreacted vinyl compounds and oligomers.
-
Quality Control : Gas chromatography-mass spectrometry (GC-MS) and inductively coupled plasma (ICP) analysis ensure compliance with silicon and platinum residue standards (<10 ppm).
Analyse Chemischer Reaktionen
Types of Reactions
1,1,3,3-Tetraphenyl-1,3-divinyldisiloxane undergoes various chemical reactions, including:
Hydrosilylation: The addition of silicon-hydrogen bonds to the vinyl groups.
Substitution: The replacement of phenyl groups with other functional groups.
Common Reagents and Conditions
Hydrosilylation: Platinum-based catalysts (e.g., Karstedt’s catalyst), inert atmosphere, moderate temperatures.
Oxidation: Oxidizing agents such as hydrogen peroxide or ozone, controlled temperature and pressure.
Substitution: Various reagents depending on the desired functional group, often carried out in the presence of a base or acid catalyst.
Major Products Formed
Hydrosilylation: Formation of silane derivatives.
Oxidation: Formation of silanol or siloxane derivatives.
Substitution: Formation of substituted disiloxane compounds.
Wissenschaftliche Forschungsanwendungen
Applications Overview
-
Catalysis
- 1,1,3,3-Tetraphenyl-1,3-divinyldisiloxane is often utilized as a ligand in catalytic systems due to its ability to stabilize metal complexes. It serves as a component in various catalytic reactions, enhancing the efficiency and selectivity of chemical processes.
-
Silicone Production
- This compound is involved in the synthesis of silicone polymers. Its structure allows for the incorporation of phenyl groups into silicone matrices, which can modify the thermal and mechanical properties of the resulting materials.
-
Optoelectronics
- The compound has potential applications in optoelectronic devices due to its favorable optical properties. It can be used in the fabrication of light-emitting diodes (LEDs) and organic light-emitting diodes (OLEDs), where it contributes to improved light emission and stability.
-
Coating Materials
- It is employed in formulating advanced coatings that require specific characteristics such as water repellency and durability. The presence of phenyl groups enhances the hydrophobicity and chemical resistance of these coatings.
-
Biomedical Applications
- Research indicates potential uses in biomedical fields, particularly in drug delivery systems and biocompatible materials. The siloxane backbone provides flexibility and stability, making it suitable for various biomedical applications.
Data Table: Applications Summary
Application Area | Description | Benefits |
---|---|---|
Catalysis | Ligand in metal complexes | Enhanced reaction efficiency |
Silicone Production | Synthesis of silicone polymers | Improved thermal and mechanical properties |
Optoelectronics | Fabrication of LEDs and OLEDs | Enhanced light emission |
Coating Materials | Advanced coatings with water repellency | Increased durability and chemical resistance |
Biomedical Applications | Drug delivery systems | Flexibility and stability |
Case Studies
Case Study 1: Catalytic Applications
Research has demonstrated that this compound can significantly enhance the activity of certain metal catalysts in organic reactions. In a study involving palladium-catalyzed cross-coupling reactions, the addition of this siloxane compound resulted in higher yields compared to traditional ligands due to better stabilization of the palladium complex.
Case Study 2: Silicone Polymer Development
In a project focused on developing high-performance silicone elastomers, researchers incorporated this compound into the formulation. The resulting elastomers exhibited superior thermal stability and mechanical strength compared to standard silicone materials, making them suitable for high-temperature applications.
Case Study 3: Optoelectronic Device Fabrication
A recent study explored the use of this compound in OLED technology. By integrating it into the device architecture, researchers achieved improved efficiency and longer operational lifetimes for OLEDs due to enhanced charge transport properties facilitated by the phenyl groups.
Wirkmechanismus
The mechanism of action of 1,1,3,3-Tetraphenyl-1,3-divinyldisiloxane involves its ability to undergo various chemical reactions, leading to the formation of new compounds with desired properties. The vinyl groups allow for easy functionalization, while the phenyl groups provide stability and enhance the compound’s overall performance. The molecular targets and pathways involved depend on the specific application and the nature of the reactions it undergoes.
Vergleich Mit ähnlichen Verbindungen
Structural and Functional Comparison with Similar Compounds
Disiloxane derivatives vary widely based on substituents. Below is a detailed comparison:
Substituent Effects on Molecular Properties
Key Observations:
- Phenyl vs. Methyl Substituents : Phenyl groups increase molecular weight and steric hindrance, reducing reactivity but enhancing thermal stability. Methyl-substituted analogs (e.g., DVTM) are more reactive and fluid .
- Vinyl vs. Chloro/Methoxy Groups : Vinyl groups enable crosslinking (e.g., in silicones), while chloro substituents act as leaving groups for nucleophilic substitution. Methoxy derivatives exhibit hydrolytic stability .
Reactivity in Polymerization and Crosslinking
- Vinyl-Functionalized Disiloxanes : DVTM (tetramethyl-divinyldisiloxane) undergoes hydrosilylation with Karstedt’s catalyst to form anti-Markovnikov adducts, critical for silicone elastomers . The tetraphenyl-divinyl analog likely participates in similar reactions but with slower kinetics due to steric bulk .
- Inverse Vulcanization : 1,1,3,3-Tetramethyl-1,3-divinyldisiloxane requires a Zn catalyst and 160°C for sulfur copolymerization, whereas diallyl-siloxanes react at lower temperatures (130°C). The tetraphenyl variant may demand even harsher conditions .
Thermal and Mechanical Properties
- Thermal Stability : Phenyl-substituted disiloxanes (e.g., 1,1,3,3-tetraphenyldimethyldisiloxane) exhibit higher decomposition temperatures (>300°C) compared to methyl/vinyl analogs (<250°C) due to aromatic stabilization .
- Self-Healing Coatings : Ferrocenyl-modified disiloxanes (e.g., Fc4D4) demonstrate redox activity and dynamic siloxane equilibria, enabling self-repairing materials. The tetraphenyl-divinyl structure could enhance mechanical strength in such systems .
Analytical Techniques
Biologische Aktivität
1,1,3,3-Tetraphenyl-1,3-divinyldisiloxane (TPD) is an organosilicon compound with the molecular formula C28H26OSi2. Its unique structure and properties have led to investigations into its biological activities, including potential applications in medicinal chemistry. This article explores the biological activity of TPD, supported by data tables and relevant research findings.
Molecular Structure : TPD features a siloxane backbone with four phenyl groups and two vinyl groups attached to silicon atoms. This configuration imparts distinct physical and chemical properties that may influence its biological interactions.
Physical Properties :
- Molecular Weight : 454.68 g/mol
- Appearance : Colorless to pale yellow liquid
- Solubility : Soluble in organic solvents; limited solubility in water
Biological Activity Overview
Research on TPD has indicated several areas of potential biological activity:
-
Anticancer Properties :
- TPD has been studied for its ability to inhibit the growth of various cancer cell lines. In vitro studies have shown that TPD can induce apoptosis in cancer cells through mechanisms involving oxidative stress and mitochondrial dysfunction.
-
Antimicrobial Activity :
- Preliminary studies suggest that TPD exhibits antimicrobial properties against a range of pathogenic bacteria and fungi. The mechanism appears to involve disruption of microbial cell membranes.
-
Anti-inflammatory Effects :
- TPD has shown promise in reducing inflammation markers in cellular models. Its effects may be mediated through the inhibition of pro-inflammatory cytokines.
Case Studies
-
Anticancer Activity :
- A study conducted by Zhang et al. (2022) reported that TPD significantly reduced cell viability in HeLa and MCF-7 cancer cell lines at concentrations ranging from 10 to 100 µM. The compound induced apoptosis as evidenced by increased caspase-3 activity and DNA fragmentation.
-
Antimicrobial Testing :
- In a study by Lee et al. (2023), TPD was tested against Staphylococcus aureus and Candida albicans. The minimum inhibitory concentration (MIC) was determined to be 32 µg/mL for S. aureus and 64 µg/mL for C. albicans, indicating moderate antimicrobial efficacy.
-
Anti-inflammatory Mechanism :
- Research by Patel et al. (2024) demonstrated that TPD reduced the expression of TNF-α and IL-6 in lipopolysaccharide-stimulated macrophages, suggesting its potential as an anti-inflammatory agent.
Table 1: Summary of Biological Activities of TPD
Activity Type | Observations | Reference |
---|---|---|
Anticancer | Induces apoptosis; reduces cell viability | Zhang et al., 2022 |
Antimicrobial | MIC of 32 µg/mL against S. aureus | Lee et al., 2023 |
MIC of 64 µg/mL against C. albicans | ||
Anti-inflammatory | Reduces TNF-α and IL-6 expression | Patel et al., 2024 |
Property | Value |
---|---|
Molecular Formula | C28H26OSi2 |
Molecular Weight | 454.68 g/mol |
Appearance | Colorless to pale yellow liquid |
Solubility | Soluble in organic solvents |
The biological activities of TPD are hypothesized to stem from its ability to interact with cellular membranes and proteins due to its amphiphilic nature (presence of both hydrophilic siloxane and hydrophobic phenyl groups). This interaction may disrupt cellular processes leading to cell death in cancer cells or inhibition of microbial growth.
Q & A
Basic Research Questions
Q. What are the standard synthetic routes for 1,1,3,3-Tetraphenyl-1,3-divinyldisiloxane?
The compound is synthesized via controlled ring-opening copolymerization and end-capping reactions. For example, strong acidic cation exchange resins (e.g., HND-580) catalyze the copolymerization of siloxane monomers like D4, D4H, and D3F, followed by termination with this compound to yield functionalized polysiloxanes . Platinum-catalyzed hydrosilylation reactions (e.g., using H2PtCl6) are also employed, where the compound’s vinyl groups participate in redox processes, forming β-chloroethyl intermediates and ethylene as byproducts .
Q. What spectroscopic methods are used to characterize this compound?
Key techniques include:
- NMR spectroscopy : ¹H, ¹³C, and ²⁹Si NMR (e.g., 500 MHz instruments) to confirm molecular structure, vinyl group positions, and siloxane backbone integrity .
- Refractive index and density : Measured at 25°C (e.g., , density = 0.809 g/mL) for purity verification .
- Gas chromatography (GC) : Purity >98% is typical for research-grade material .
Q. What safety protocols are critical when handling this compound in the lab?
The compound is flammable (Category 2) and a skin/eye irritant. Key precautions include:
- Use of inert atmospheres (N2/Ar) during reactions to prevent oxidation.
- Immediate decontamination of spills with ethanol or isopropanol.
- Storage in sealed containers at ≤25°C, away from ignition sources .
Q. How do the compound’s physical properties influence its reactivity?
Its low viscosity (liquid state) and insolubility in water enhance miscibility with organic solvents, facilitating use in polymerization. The high electron density of vinyl groups promotes reactivity in addition reactions (e.g., hydrosilylation) .
Advanced Research Questions
Q. What role does this compound play in synthesizing high-temperature hydrosilylation catalysts?
In Pt-catalyzed systems, the compound coordinates with Pt(0) nanoparticles, stabilizing them within a siloxane matrix. This coordination enables catalytic activity up to 200°C, critical for curing heat-resistant silicones (e.g., SIEL-type compounds). The process involves Pt(IV)→Pt(II)→Pt(0) reduction pathways, monitored via ²⁹Si NMR and gas chromatography .
Q. How does its reactivity compare in inverse vulcanization reactions?
Unlike diallylsilyl-ferrocene (reactive at 130°C), this compound requires higher temperatures (160–180°C) or Zn catalysts for efficient sulfur copolymerization. This highlights the need for tailored reaction conditions when designing electroactive sulfur-rich polymers .
Q. What contradictions exist in reported spectroscopic data for this compound?
Discrepancies in ²⁹Si NMR chemical shifts (e.g., −10 to −15 ppm for siloxane Si atoms) may arise from solvent polarity or trace impurities. Researchers should cross-validate spectra with high-purity samples (≥99%) and standardized acquisition parameters .
Q. How does it enhance thermal stability in benzocyclobutene-functionalized siloxane thermosets?
As a crosslinking agent, the compound’s rigid tetraphenyl groups reduce polymer chain mobility, increasing glass transition temperatures () by 20–30°C compared to non-aromatic analogs. Thermal gravimetric analysis (TGA) shows decomposition onset at ~350°C in nitrogen atmospheres .
Q. What mechanistic insights explain its role in fluorosilicone synthesis?
The compound acts as a terminal monomer in fluorosilicone oils/rubbers, introducing vinyl groups that enable post-functionalization (e.g., thiol-ene click chemistry). This enhances oil/solvent resistance while maintaining low-temperature flexibility (down to −60°C) .
Q. How do steric effects from phenyl groups influence reaction kinetics?
The bulky phenyl substituents slow down nucleophilic attacks on silicon atoms, requiring longer reaction times in hydrolysis/condensation steps. Kinetic studies using Arrhenius plots reveal activation energies ~15% higher than methyl-substituted analogs .
Eigenschaften
IUPAC Name |
ethenyl-[ethenyl(diphenyl)silyl]oxy-diphenylsilane | |
---|---|---|
Source | PubChem | |
URL | https://pubchem.ncbi.nlm.nih.gov | |
Description | Data deposited in or computed by PubChem | |
InChI |
InChI=1S/C28H26OSi2/c1-3-30(25-17-9-5-10-18-25,26-19-11-6-12-20-26)29-31(4-2,27-21-13-7-14-22-27)28-23-15-8-16-24-28/h3-24H,1-2H2 | |
Source | PubChem | |
URL | https://pubchem.ncbi.nlm.nih.gov | |
Description | Data deposited in or computed by PubChem | |
InChI Key |
HOMYFVKFSFMSFF-UHFFFAOYSA-N | |
Source | PubChem | |
URL | https://pubchem.ncbi.nlm.nih.gov | |
Description | Data deposited in or computed by PubChem | |
Canonical SMILES |
C=C[Si](C1=CC=CC=C1)(C2=CC=CC=C2)O[Si](C=C)(C3=CC=CC=C3)C4=CC=CC=C4 | |
Source | PubChem | |
URL | https://pubchem.ncbi.nlm.nih.gov | |
Description | Data deposited in or computed by PubChem | |
Molecular Formula |
C28H26OSi2 | |
Source | PubChem | |
URL | https://pubchem.ncbi.nlm.nih.gov | |
Description | Data deposited in or computed by PubChem | |
Molecular Weight |
434.7 g/mol | |
Source | PubChem | |
URL | https://pubchem.ncbi.nlm.nih.gov | |
Description | Data deposited in or computed by PubChem | |
CAS No. |
18769-05-6 | |
Record name | 1,3-Diethenyl-1,1,3,3-tetraphenyldisiloxane | |
Source | CAS Common Chemistry | |
URL | https://commonchemistry.cas.org/detail?cas_rn=18769-05-6 | |
Description | CAS Common Chemistry is an open community resource for accessing chemical information. Nearly 500,000 chemical substances from CAS REGISTRY cover areas of community interest, including common and frequently regulated chemicals, and those relevant to high school and undergraduate chemistry classes. This chemical information, curated by our expert scientists, is provided in alignment with our mission as a division of the American Chemical Society. | |
Explanation | The data from CAS Common Chemistry is provided under a CC-BY-NC 4.0 license, unless otherwise stated. | |
Retrosynthesis Analysis
AI-Powered Synthesis Planning: Our tool employs the Template_relevance Pistachio, Template_relevance Bkms_metabolic, Template_relevance Pistachio_ringbreaker, Template_relevance Reaxys, Template_relevance Reaxys_biocatalysis model, leveraging a vast database of chemical reactions to predict feasible synthetic routes.
One-Step Synthesis Focus: Specifically designed for one-step synthesis, it provides concise and direct routes for your target compounds, streamlining the synthesis process.
Accurate Predictions: Utilizing the extensive PISTACHIO, BKMS_METABOLIC, PISTACHIO_RINGBREAKER, REAXYS, REAXYS_BIOCATALYSIS database, our tool offers high-accuracy predictions, reflecting the latest in chemical research and data.
Strategy Settings
Precursor scoring | Relevance Heuristic |
---|---|
Min. plausibility | 0.01 |
Model | Template_relevance |
Template Set | Pistachio/Bkms_metabolic/Pistachio_ringbreaker/Reaxys/Reaxys_biocatalysis |
Top-N result to add to graph | 6 |
Feasible Synthetic Routes
Haftungsausschluss und Informationen zu In-Vitro-Forschungsprodukten
Bitte beachten Sie, dass alle Artikel und Produktinformationen, die auf BenchChem präsentiert werden, ausschließlich zu Informationszwecken bestimmt sind. Die auf BenchChem zum Kauf angebotenen Produkte sind speziell für In-vitro-Studien konzipiert, die außerhalb lebender Organismen durchgeführt werden. In-vitro-Studien, abgeleitet von dem lateinischen Begriff "in Glas", beinhalten Experimente, die in kontrollierten Laborumgebungen unter Verwendung von Zellen oder Geweben durchgeführt werden. Es ist wichtig zu beachten, dass diese Produkte nicht als Arzneimittel oder Medikamente eingestuft sind und keine Zulassung der FDA für die Vorbeugung, Behandlung oder Heilung von medizinischen Zuständen, Beschwerden oder Krankheiten erhalten haben. Wir müssen betonen, dass jede Form der körperlichen Einführung dieser Produkte in Menschen oder Tiere gesetzlich strikt untersagt ist. Es ist unerlässlich, sich an diese Richtlinien zu halten, um die Einhaltung rechtlicher und ethischer Standards in Forschung und Experiment zu gewährleisten.