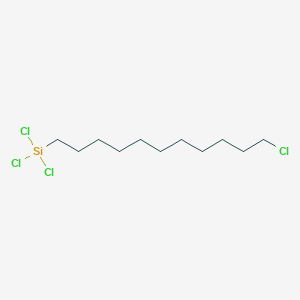
11-Chloroundecyltrichlorosilane
Übersicht
Beschreibung
11-Chloroundecyltrichlorosilane is a chemical compound with the molecular formula C11H22Cl4Si . It contains a total of 38 atoms, including 22 Hydrogen atoms, 11 Carbon atoms, and 4 Chlorine atoms .
Molecular Structure Analysis
The 11-chloroundecyltrichlorosilane molecule contains a total of 37 bond(s) including 15 non-H bond(s) and 9 rotatable bond(s) . More detailed structural analysis might require advanced computational methods or experimental techniques .Physical And Chemical Properties Analysis
11-Chloroundecyltrichlorosilane has a boiling point of 188°C at 15mmHg, a density of 1.1204 g/mL, and a refractive index of 1.4688 at 20°C .Wissenschaftliche Forschungsanwendungen
Surface Control in Liquid-Crystal Displays
Chemisorbed monolayers of 11-Chloroundecyltrichlorosilane and similar compounds have been investigated for their potential in controlling liquid-crystal orientation. The high degree of registry in these monolayers suggests promising applications in display technologies (Geer, Yang, & Frank, 1997).
Model Studies for Silica Surfaces
Silsesquioxanes derived from chlorosilanes, like 11-Chloroundecyltrichlorosilane, offer models for understanding silica surfaces. These compounds have structural similarities to various forms of silica, aiding in the study of surface chemistry (Feher, Newman, & Walzer, 1989).
Bacteria/Material Biointerface Studies
11-Chloroundecyltrichlorosilane-based self-assembled monolayers (SAMs) on silicon substrates have been used to study bacteria/material biointerfaces. These SAMs provide highly controlled surfaces for examining bacterial adhesion, crucial for biomaterials research (Böhmler, Ponche, Anselme, & Ploux, 2013).
Protective Films in Electrochemistry
Alkanethiol monolayers modified with chlorosilanes, including 11-Chloroundecyltrichlorosilane, have been developed to create protective films on metal surfaces. These films demonstrate high protective efficiency, which is essential in corrosion prevention and electrochemistry (Haneda & Aramaki, 1998).
Environmental and Analytical Chemistry
Chlorosilane-based nanoparticles, modified with compounds like 11-Chloroundecyltrichlorosilane, have been synthesized for sensitive detection of latent fingerprints, offering practical applications in forensic science (Huang et al., 2015).
Safety and Hazards
Eigenschaften
IUPAC Name |
trichloro(11-chloroundecyl)silane | |
---|---|---|
Source | PubChem | |
URL | https://pubchem.ncbi.nlm.nih.gov | |
Description | Data deposited in or computed by PubChem | |
InChI |
InChI=1S/C11H22Cl4Si/c12-10-8-6-4-2-1-3-5-7-9-11-16(13,14)15/h1-11H2 | |
Source | PubChem | |
URL | https://pubchem.ncbi.nlm.nih.gov | |
Description | Data deposited in or computed by PubChem | |
InChI Key |
AISIWSXYGRYXLI-UHFFFAOYSA-N | |
Source | PubChem | |
URL | https://pubchem.ncbi.nlm.nih.gov | |
Description | Data deposited in or computed by PubChem | |
Canonical SMILES |
C(CCCCC[Si](Cl)(Cl)Cl)CCCCCCl | |
Source | PubChem | |
URL | https://pubchem.ncbi.nlm.nih.gov | |
Description | Data deposited in or computed by PubChem | |
Molecular Formula |
C11H22Cl4Si | |
Source | PubChem | |
URL | https://pubchem.ncbi.nlm.nih.gov | |
Description | Data deposited in or computed by PubChem | |
Molecular Weight |
324.2 g/mol | |
Source | PubChem | |
URL | https://pubchem.ncbi.nlm.nih.gov | |
Description | Data deposited in or computed by PubChem | |
Product Name |
11-Chloroundecyltrichlorosilane |
Retrosynthesis Analysis
AI-Powered Synthesis Planning: Our tool employs the Template_relevance Pistachio, Template_relevance Bkms_metabolic, Template_relevance Pistachio_ringbreaker, Template_relevance Reaxys, Template_relevance Reaxys_biocatalysis model, leveraging a vast database of chemical reactions to predict feasible synthetic routes.
One-Step Synthesis Focus: Specifically designed for one-step synthesis, it provides concise and direct routes for your target compounds, streamlining the synthesis process.
Accurate Predictions: Utilizing the extensive PISTACHIO, BKMS_METABOLIC, PISTACHIO_RINGBREAKER, REAXYS, REAXYS_BIOCATALYSIS database, our tool offers high-accuracy predictions, reflecting the latest in chemical research and data.
Strategy Settings
Precursor scoring | Relevance Heuristic |
---|---|
Min. plausibility | 0.01 |
Model | Template_relevance |
Template Set | Pistachio/Bkms_metabolic/Pistachio_ringbreaker/Reaxys/Reaxys_biocatalysis |
Top-N result to add to graph | 6 |
Feasible Synthetic Routes
Haftungsausschluss und Informationen zu In-Vitro-Forschungsprodukten
Bitte beachten Sie, dass alle Artikel und Produktinformationen, die auf BenchChem präsentiert werden, ausschließlich zu Informationszwecken bestimmt sind. Die auf BenchChem zum Kauf angebotenen Produkte sind speziell für In-vitro-Studien konzipiert, die außerhalb lebender Organismen durchgeführt werden. In-vitro-Studien, abgeleitet von dem lateinischen Begriff "in Glas", beinhalten Experimente, die in kontrollierten Laborumgebungen unter Verwendung von Zellen oder Geweben durchgeführt werden. Es ist wichtig zu beachten, dass diese Produkte nicht als Arzneimittel oder Medikamente eingestuft sind und keine Zulassung der FDA für die Vorbeugung, Behandlung oder Heilung von medizinischen Zuständen, Beschwerden oder Krankheiten erhalten haben. Wir müssen betonen, dass jede Form der körperlichen Einführung dieser Produkte in Menschen oder Tiere gesetzlich strikt untersagt ist. Es ist unerlässlich, sich an diese Richtlinien zu halten, um die Einhaltung rechtlicher und ethischer Standards in Forschung und Experiment zu gewährleisten.