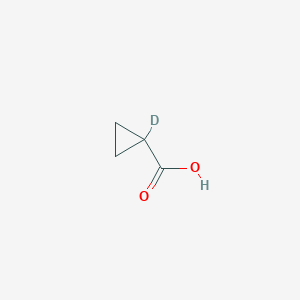
1-Deuteriocyclopropan-1-carbonsäure
Übersicht
Beschreibung
Cyclopropane-1-D1-carboxylic acid is a deuterated analog of cyclopropane-1-carboxylic acid Deuterium, a stable isotope of hydrogen, replaces one hydrogen atom in the cyclopropane ring
Wissenschaftliche Forschungsanwendungen
Cyclopropane-1-D1-carboxylic acid has several scientific research applications:
Organic Synthesis: It is used as a building block in the synthesis of more complex organic molecules.
Medicinal Chemistry: Deuterated compounds often exhibit altered metabolic profiles, making them useful in drug development.
Isotope Labeling: Deuterium labeling is used in mechanistic studies to trace reaction pathways and understand reaction mechanisms.
Wirkmechanismus
Target of Action
1-Deuteriocyclopropane-1-carboxylic acid (also known as Cyclopropane-1-carboxylic Acid-d1) is a derivative of 1-Aminocyclopropane-1-carboxylic acid (ACC), which is the direct precursor of the plant hormone ethylene . The primary targets of this compound are ACC synthases (ACSs) and ACC oxidases (ACOs), which are involved in the synthesis and oxidation of ACC, respectively .
Mode of Action
The compound interacts with its targets, ACSs and ACOs, to influence the biosynthesis of ethylene. ACC is synthesized from S-adenosyl-L-methionine (SAM) by ACSs and subsequently oxidized to ethylene by ACOs . This interaction results in changes in the levels of ethylene, a plant hormone that regulates a wide range of developmental processes and responses to biotic and abiotic stresses .
Biochemical Pathways
The affected biochemical pathway is the ethylene biosynthesis pathway. The biosynthesis of ethylene starts with the conversion of the amino acid methionine to S-adenosyl L-methionine (SAM) by SAM synthetase. SAM is then converted to ACC by ACS, and ACC is oxidized to ethylene by ACO . This pathway is regulated by developmental, hormonal, and environmental cues .
Result of Action
The action of 1-Deuteriocyclopropane-1-carboxylic acid results in changes in the levels of ethylene in plants. Ethylene regulates a wide range of developmental processes and responses to biotic and abiotic stresses . These include germination, leaf and flower senescence and abscission, fruit ripening, and responses to various stresses .
Action Environment
The action of 1-Deuteriocyclopropane-1-carboxylic acid is influenced by various environmental factors. The rate of ACC formation, and thus ethylene production, differs in response to developmental, hormonal, and environmental cues
Biochemische Analyse
Biochemical Properties
1-Deuteriocyclopropane-1-carboxylic acid interacts with several enzymes and proteins. It is converted from SAM by ACC synthases, a member of the pyridoxal-5′-phosphate (PLP) dependent aminotransferases . This conversion is the first committed and generally rate-limiting step in ethylene biosynthesis . The interaction between 1-Deuteriocyclopropane-1-carboxylic acid and these enzymes is crucial for the production of ethylene .
Cellular Effects
1-Deuteriocyclopropane-1-carboxylic acid has significant effects on various types of cells and cellular processes. It influences cell function by acting as a precursor to the plant hormone ethylene . Ethylene regulates a wide range of developmental processes and responses to biotic and abiotic stresses . Therefore, the presence of 1-Deuteriocyclopropane-1-carboxylic acid indirectly impacts cell signaling pathways, gene expression, and cellular metabolism .
Molecular Mechanism
The molecular mechanism of 1-Deuteriocyclopropane-1-carboxylic acid involves its conversion to ethylene. This process starts with the conversion of the amino acid methionine to SAM by SAM synthetase. SAM is then converted to 1-Deuteriocyclopropane-1-carboxylic acid by ACC synthase . The by-product of this reaction, 5’-methylthioadenosine (MTA), is recycled back into the Yang cycle while 1-Deuteriocyclopropane-1-carboxylic acid is oxidized to ethylene by ACC oxidase .
Temporal Effects in Laboratory Settings
The effects of 1-Deuteriocyclopropane-1-carboxylic acid over time in laboratory settings are primarily related to its role in ethylene production. The rate of 1-Deuteriocyclopropane-1-carboxylic acid formation differs in response to developmental, hormonal, and environmental cues
Metabolic Pathways
1-Deuteriocyclopropane-1-carboxylic acid is involved in the ethylene biosynthesis pathway . It is converted from SAM, which is derived from the amino acid methionine . This conversion is catalyzed by ACC synthase . The resulting 1-Deuteriocyclopropane-1-carboxylic acid is then oxidized to ethylene by ACC oxidase .
Transport and Distribution
1-Deuteriocyclopropane-1-carboxylic acid is transported within cells and throughout the plant. Some evidence suggests the existence of ACC transporters
Subcellular Localization
The enzymes that convert it into ethylene, ACC synthase and ACC oxidase, are known to be localized in the cytosol
Vorbereitungsmethoden
Synthetic Routes and Reaction Conditions: Cyclopropane-1-D1-carboxylic acid can be synthesized through several methods:
Alkylation of Glycine Equivalents: This involves the alkylation of glycine equivalents with 1,2-electrophiles, followed by cyclization to form the cyclopropane ring.
Intramolecular Cyclization: γ-Substituted amino acid derivatives undergo intramolecular cyclization to form the cyclopropane ring.
Cyclopropanation of Alkenes: Alkenes can be cyclopropanated using diazo compounds, ylides, or carbene intermediates.
Industrial Production Methods: Industrial production methods for 1-deuteriocyclopropane-1-carboxylic acid are similar to those used for other cyclopropane derivatives. These methods typically involve large-scale cyclopropanation reactions using diazo compounds or other carbene sources under controlled conditions to ensure high yield and purity .
Analyse Chemischer Reaktionen
Cyclopropane-1-D1-carboxylic acid undergoes various chemical reactions, including:
Oxidation: The carboxylic acid group can be oxidized to form corresponding ketones or aldehydes.
Reduction: The carboxylic acid group can be reduced to form alcohols or alkanes.
Substitution: The hydrogen atoms in the cyclopropane ring can be substituted with other functional groups using appropriate reagents and conditions.
Common Reagents and Conditions:
Oxidation: Common oxidizing agents include potassium permanganate and chromium trioxide.
Reduction: Reducing agents such as lithium aluminum hydride and sodium borohydride are commonly used.
Substitution: Halogenation can be achieved using halogens like chlorine or bromine in the presence of a catalyst.
Major Products:
Oxidation: Ketones, aldehydes.
Reduction: Alcohols, alkanes.
Substitution: Halogenated cyclopropane derivatives.
Vergleich Mit ähnlichen Verbindungen
Cyclopropane-1-D1-carboxylic acid can be compared with other similar compounds such as:
Cyclopropane-1-carboxylic acid: The non-deuterated analog, which has similar chemical properties but different kinetic behaviors.
1-Aminocyclopropane-1-carboxylic acid: A related compound used in plant hormone research.
Cyclopropane derivatives: Other cyclopropane-containing compounds with various functional groups
Uniqueness: The uniqueness of 1-deuteriocyclopropane-1-carboxylic acid lies in its deuterium content, which imparts distinct kinetic and metabolic properties compared to its non-deuterated analogs .
Eigenschaften
IUPAC Name |
1-deuteriocyclopropane-1-carboxylic acid | |
---|---|---|
Source | PubChem | |
URL | https://pubchem.ncbi.nlm.nih.gov | |
Description | Data deposited in or computed by PubChem | |
InChI |
InChI=1S/C4H6O2/c5-4(6)3-1-2-3/h3H,1-2H2,(H,5,6)/i3D | |
Source | PubChem | |
URL | https://pubchem.ncbi.nlm.nih.gov | |
Description | Data deposited in or computed by PubChem | |
InChI Key |
YMGUBTXCNDTFJI-WFVSFCRTSA-N | |
Source | PubChem | |
URL | https://pubchem.ncbi.nlm.nih.gov | |
Description | Data deposited in or computed by PubChem | |
Canonical SMILES |
C1CC1C(=O)O | |
Source | PubChem | |
URL | https://pubchem.ncbi.nlm.nih.gov | |
Description | Data deposited in or computed by PubChem | |
Isomeric SMILES |
[2H]C1(CC1)C(=O)O | |
Source | PubChem | |
URL | https://pubchem.ncbi.nlm.nih.gov | |
Description | Data deposited in or computed by PubChem | |
Molecular Formula |
C4H6O2 | |
Source | PubChem | |
URL | https://pubchem.ncbi.nlm.nih.gov | |
Description | Data deposited in or computed by PubChem | |
Molecular Weight |
87.10 g/mol | |
Source | PubChem | |
URL | https://pubchem.ncbi.nlm.nih.gov | |
Description | Data deposited in or computed by PubChem | |
Synthesis routes and methods I
Procedure details
Synthesis routes and methods II
Procedure details
Haftungsausschluss und Informationen zu In-Vitro-Forschungsprodukten
Bitte beachten Sie, dass alle Artikel und Produktinformationen, die auf BenchChem präsentiert werden, ausschließlich zu Informationszwecken bestimmt sind. Die auf BenchChem zum Kauf angebotenen Produkte sind speziell für In-vitro-Studien konzipiert, die außerhalb lebender Organismen durchgeführt werden. In-vitro-Studien, abgeleitet von dem lateinischen Begriff "in Glas", beinhalten Experimente, die in kontrollierten Laborumgebungen unter Verwendung von Zellen oder Geweben durchgeführt werden. Es ist wichtig zu beachten, dass diese Produkte nicht als Arzneimittel oder Medikamente eingestuft sind und keine Zulassung der FDA für die Vorbeugung, Behandlung oder Heilung von medizinischen Zuständen, Beschwerden oder Krankheiten erhalten haben. Wir müssen betonen, dass jede Form der körperlichen Einführung dieser Produkte in Menschen oder Tiere gesetzlich strikt untersagt ist. Es ist unerlässlich, sich an diese Richtlinien zu halten, um die Einhaltung rechtlicher und ethischer Standards in Forschung und Experiment zu gewährleisten.