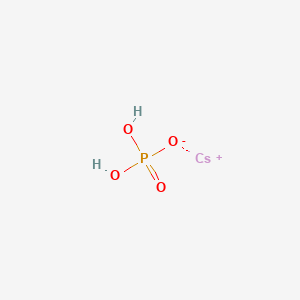
Caesium dihydrogen phosphate
- Klicken Sie auf QUICK INQUIRY, um ein Angebot von unserem Expertenteam zu erhalten.
- Mit qualitativ hochwertigen Produkten zu einem WETTBEWERBSFÄHIGEN Preis können Sie sich mehr auf Ihre Forschung konzentrieren.
Übersicht
Beschreibung
Caesium dihydrogen phosphate, with the chemical formula CsH2PO4, is a compound that consists of cesium, hydrogen, phosphorus, and oxygen. It is a highly insoluble and thermally stable salt, making it suitable for various applications, particularly in the fields of glass, optics, and ceramics. The compound is known for its unique properties, such as its ability to conduct protons, which makes it a candidate for use in solid oxide fuel cells. The molecular weight of this compound is approximately 229.89 g/mol. It is often utilized in research and industrial applications due to its stability and low solubility in water. The compound can be synthesized through the reaction of phosphoric acid with cesium hydroxide or cesium carbonate. In addition to its practical applications, this compound is also studied for its potential in advanced materials science, particularly in the development of new ionic conductors and in the field of nanotechnology.
Vorbereitungsmethoden
Common Preparation methods
Aqueous Solution Method
The aqueous solution method is one of the most straightforward techniques for preparing CsH2PO4. This process typically involves:
Dissolving caesium carbonate (CsCO3) and phosphoric acid (H3PO4) in water.
Mixing the reactants in a 1:1 molar ratio.
Adding methanol to precipitate CsH2PO4.
Filtering and drying the precipitate at 80°C for 12 hours[“].
This method is favored for its simplicity and ability to produce high-purity CsH2PO4.
Precipitation Method
The precipitation method is another common approach, which may involve:
Preparing a solution of caesium salt and phosphate ions.
Adjusting the pH to induce precipitation.
Collecting and purifying the precipitate.
This method can be effective for producing CsH2PO4 with controlled particle size.
Sol-Gel Method
The sol-gel method offers advantages in terms of morphology control and can involve:
Preparing a sol containing caesium and phosphate precursors.
Allowing the sol to gel under controlled conditions.
Aging and drying the gel to form CsH2PO4.
This technique can result in high surface area materials, which may be beneficial for certain applications.
Advanced Preparation Techniques
Electrospinning Method
Electrospinning is an advanced technique that can be used to produce CsH2PO4 nanofibers[“]. The process typically involves:
Preparing a solution of CsH2PO4 precursors in a suitable solvent.
Using an electric field to draw the solution into fibers.
Collecting and processing the fibers to obtain CsH2PO4 nanostructures.
This method can create materials with high surface area and unique properties.
Hydrothermal Synthesis
Hydrothermal synthesis is a powerful technique for preparing CsH2PO4 under controlled temperature and pressure conditions2. The process may include:
Mixing reactants in an aqueous solution.
Sealing the mixture in a pressure vessel.
Heating to temperatures above 100°C under autogenous pressure.
Cooling and recovering the CsH2PO4 product.
This method can produce well-crystallized materials with specific morphologies.
Optimization of Preparation methods
Temperature Effects
Temperature plays a crucial role in the formation and crystallization of CsH2PO4. Optimal temperatures typically range from 80°C to 250°C, depending on the specific preparation method[“].
pH Control
Maintaining the correct pH is essential for the successful synthesis of CsH2PO4. The optimal pH range can vary depending on the preparation method but is often near neutral or slightly acidic.
Reaction Time Optimization
The duration of the reaction can significantly affect the yield and quality of CsH2PO4. Reaction times can range from a few hours to several days, depending on the method and desired properties of the final product[“].
Purification Techniques
Purification is crucial for obtaining high-quality CsH2PO4. Common techniques include:
Recrystallization
Washing with appropriate solvents
Centrifugation or filtration to remove impurities
Characterization of Prepared Caesium dihydrogen phosphate
Analyse Chemischer Reaktionen
Thermal Analysis of CsH2PO4
Thermogravimetric Analysis (TGA)
Thermogravimetric analysis is a crucial technique for studying the thermal behavior of CsH2PO4. TGA reveals the mass changes of the compound as it undergoes various thermal processes[“].
Differential Scanning Calorimetry (DSC)
DSC provides insights into the phase transitions and thermal events occurring in CsH2PO4. It is particularly useful for identifying the superprotonic phase transition, which occurs at approximately 230°C[“].
Dehydration Behavior
The dehydration of CsH2PO4 occurs in two distinct temperature ranges:
First dehydration: 237-239°C
Second dehydration: 290-295°C
These dehydration events are accompanied by significant mass loss, typically in the range of 6.62-6.97 wt%[“].
Dehydration Reactions of CsH2PO4
CsH2PO4 to Cs2H2P2O7
The first step in the dehydration process involves the transformation of CsH2PO4 to caesium hydrogen pyrophosphate (Cs2H2P2O7)[“].
Cs2H2P2O7 to CsPO3
The second step of dehydration results in the formation of caesium metaphosphate (CsPO3).
Mass Loss Analysis
The observed mass loss during dehydration is slightly less than the theoretical value of 7.8 wt%, indicating that the reaction from CsH2PO4 to Cs2H2P2O7 is more prevalent than the complete dehydration to CsPO3.
Activation Energy Calculations
The activation energy for the two-step dehydration process can be calculated using the Kissinger equation. The first step (CsH2PO4 → Cs2H2P2O7) typically has a lower activation energy and proceeds faster than the second step (Cs2H2P2O7 → CsPO3).
Surfactant Effects on CsH2PO4 Reactions
Influence of CTAB
Cetyltrimethylammonium bromide (CTAB) has been shown to affect the dehydration behavior and conductivity of CsH2PO4.
Influence of F-68
Polyoxyethylene-polyoxypropylene (F-68) also impacts the thermal properties and conductivity of CsH2PO4[“].
Influence of F-68:CTAB Mixtures
Mixtures of F-68 and CTAB in specific molar ratios can further modify the behavior of CsH2PO4, affecting its dehydration characteristics and conductivity.
Proton Conductivity and Phase Transitions
Superprotonic Phase Transition
CsH2PO4 undergoes a superprotonic phase transition at approximately 230°C, resulting in a significant increase in proton conductivity[“].
Temperature-dependent Conductivity
The conductivity of CsH2PO4 shows a strong dependence on temperature, with a dramatic increase observed at the superprotonic phase transition[“].
Arrhenius Relation Analysis
The conductivity of CsH2PO4 and its doped variants typically follows the Arrhenius relation, allowing for the calculation of activation energies for proton conduction.
Chemical Reactions in Composite Materials
CsH2PO4/SiP2O7 Composites
Composites of CsH2PO4 and silicon pyrophosphate (SiP2O7) have been investigated for their potential use in intermediate-temperature fuel cells[“].
Interaction with Silicon Phosphates
The interaction between CsH2PO4 and SiP2O7 can lead to the formation of new phases, such as CsH5(PO4)2, which can affect the overall properties of the composite[“].
Humidity Effects on Reactions
The presence of humidity plays a crucial role in the behavior of CsH2PO4 and its composites, particularly in maintaining the high-conductivity phase and preventing dehydration.
Wissenschaftliche Forschungsanwendungen
Solid Acid Fuel Cells (SAFC)
CsH2PO4 has gained considerable attention as an electrolyte material for solid acid fuel cells (SAFCs). These fuel cells operate at intermediate temperatures, typically around 250°C, which offers several advantages:
Higher tolerance to catalyst poisoning, especially by carbon monoxide
Improved catalyst kinetics
Potential for reduced precious metal loading in electrodes
SAFCs using CsH2PO4 as the electrolyte have demonstrated impressive performance, with peak power densities exceeding 400 mW/cm2 at 250°C[“]. The high proton conductivity of CsH2PO4 in its superprotonic phase, which occurs above 228°C, is key to this performance.
Proton Exchange Membrane Water Electrolysis (PEMWE)
CsH2PO4 has shown potential as an electrolyte for Proton Exchange Membrane Water Electrolysis (PEMWE) at intermediate temperatures3. This application leverages the high proton conductivity of CsH2PO4 to potentially improve the efficiency of hydrogen production through water electrolysis.
Composite Electrolytes with CsH2PO4
Research has explored the development of composite electrolytes incorporating CsH2PO4 to enhance performance and stability:
CsH2PO4-Polyvinyl Butyral (PVB) Composites
A composite electrolyte membrane composed of polyvinyl butyral (PVB) and CsH2PO4 has been developed for solid acid fuel cells. With just 3 wt% PVB, the composite offers improved mechanical integrity while maintaining high conductivity (~28 mS cm-1 at 260°C)[“].
CsH2PO4-UiO-66 Nanocomposites
New (1−x)CsH2PO4–xUiO-66 electrolytes have been developed, showing high proton conductivity and thermal stability at 230–250°C. These nanocomposites exhibit increased proton conductivity by 1-2 orders of magnitude at lower temperatures compared to pure CsH2PO4[“].
CsH2PO4-H3PW12O40 Composites
Mechanochemically synthesized CsH2PO4–H3PW12O40 composites have shown improved ionic conductivity under anhydrous conditions. The highest ionic conductivity of 6.58×10−4 Scm−1 was achieved for the 95CDP·5WPA composite electrolyte at 170°C[“].
Catalytic Applications
CsH2PO4 has shown promise in various catalytic applications:
Dehydration reactions
Epoxidation reactions
Acid-base concerted mechanisms in catalysis
These applications leverage the unique properties of CsH2PO4 as a solid acid catalyst[“].
Electrochemical Devices
Beyond fuel cells, CsH2PO4 has potential applications in other electrochemical devices:
Medium-temperature electrochemical devices
Water electrolysis systems
Potential use in methane and methanol synthesis
Nanotechnology and Thin Film Applications
Research has explored the use of CsH2PO4 in nanotechnology:
Development of CsH2PO4 nanoparticles
Creation of thin-film electrolytes based on CsH2PO4
Potential applications in miniaturized devices
Wirkmechanismus
Crystal Structure and Phase Transitions
CsH2PO4 exhibits two distinct crystalline phases, each playing a crucial role in its mechanism of action:
Room temperature monoclinic phase: At ambient conditions, CsH2PO4 adopts a monoclinic structure with space group P21/m.
High-temperature cubic phase: Above 230°C, CsH2PO4 undergoes a phase transition to a cubic structure with space group Pm3m.
The transition between these phases, known as the superprotonic phase transition, is key to understanding the compound's proton conduction mechanism[“].
Proton Conduction Mechanisms
The proton conduction in CsH2PO4 occurs through two primary mechanisms:
Vehicle Transport Mechanism
In this mechanism, protons are transported through the material by "hitching a ride" on larger molecular species, typically water molecules.
Grotthuss Mechanism
This mechanism involves the rapid transfer of protons between adjacent hydrogen-bonded species, allowing for faster proton transport than the vehicle mechanism.
The interplay between these mechanisms, coupled with phosphate ion reorientation, contributes to the high proton conductivity observed in CsH2PO4, especially in its superprotonic phase[“].
Superprotonic Behavior
The superprotonic phase transition in CsH2PO4 occurs at approximately 230°C, resulting in a dramatic increase in proton conductivity. This behavior is characterized by:
A sharp increase in conductivity, often by several orders of magnitude.
A decrease in the activation energy for proton conduction.
A strong dependence on humidity, with water playing a crucial role in maintaining the high-conductivity phase[“].
Local Dynamics and Hydrogen Bonding
Nuclear Magnetic Resonance (NMR) spectroscopy has provided valuable insights into the local dynamics of CsH2PO4. Studies have revealed:
The presence of a complex hydrogen bond network in the crystal structure.
Rapid proton hopping between phosphate groups, contributing to the overall proton conductivity.
These local dynamics are essential for understanding the macroscopic proton transport properties of CsH2PO4[“].
Influence of Doping on Mechanism
Doping CsH2PO4 with various elements can significantly alter its proton conduction mechanism:
Cation substitution (e.g., Rb, Ba) can affect the phase transition temperature and conductivity.
Anion substitution (e.g., S, Mo, W) can modify the hydrogen bonding network and influence proton mobility.
These modifications allow for fine-tuning of CsH2PO4's properties for specific applications[“].
Nanostructure Effects on Proton Conduction
Nanostructuring CsH2PO4 can have profound effects on its proton conduction mechanism:
Formation of nanoparticles on electrospun fibers increases the surface area and number of triple-phase boundaries.
Enhanced surface proton conduction in nanostructured CsH2PO4 can contribute significantly to overall conductivity.
These nanostructure effects offer new avenues for optimizing the performance of CsH2PO4-based devices[“].
Computational Studies on CsH2PO4 Mechanism
Computational methods have provided valuable insights into the atomic-scale mechanisms of proton conduction in CsH2PO4:
Ab initio molecular dynamics simulations have revealed detailed proton transfer pathways.
Density functional theory calculations have helped elucidate the energetics of proton transfer and phosphate reorientation.
These computational studies complement experimental work and offer a deeper understanding of the fundamental processes governing CsH2PO4's behavior[“].
Vergleich Mit ähnlichen Verbindungen
Potassium Dihydrogen Phosphate (KH2PO4)
CsH2PO4 and KH2PO4 share structural similarities as both belong to the family of dihydrogen phosphates. However, their applications differ significantly:
Proton Conductivity: CsH2PO4 exhibits much higher proton conductivity due to its superprotonic phase transition at 230°C, while KH2PO4 lacks this behavior.
Applications: KH2PO4 is primarily used in nonlinear optics, whereas CsH2PO4 is favored for intermediate-temperature fuel cells due to its conductivity properties.
Ammonium Dihydrogen Phosphate (NH4H2PO4)
NH4H2PO4 is another related compound but differs from CsH2PO4 in key aspects:
Thermal Stability: NH4H2PO4 decomposes at lower temperatures compared to CsH2PO4, limiting its use in high-temperature applications.
Conductivity: CsH2PO4 outperforms NH4H2PO4 in humidified environments due to its stable superprotonic phase.
Caesium Hydrogen Sulfate (CsHSO4)
CsHSO4 is a well-known solid acid with a superprotonic phase transition at 140°C, significantly lower than CsH2PO4's transition at 230°C. While both compounds exhibit high proton conductivity in their superprotonic phases, CsHSO4 operates at lower temperatures but has reduced stability at higher ranges compared to CsH2PO4.
Neodymium Phosphate Hydrate
Neodymium phosphate hydrate shows high conductivity in humidified atmospheres but lacks the distinct superprotonic phase transition observed in CsH2PO4. Its stability at intermediate temperatures is also lower.
Cerium Ultraphosphate
Cerium ultraphosphate exhibits unique structural and thermal properties. However, its conductivity mechanisms differ from CsH2PO4 as it relies more on defect-mediated pathways rather than a superprotonic phase transition.
Composite Materials: CsH2PO4 vs Mixed Systems
CsH2PO4-SiP2O7 Composites
Composites of CsH2PO4 with silicon pyrophosphate (SiP2O7) enhance thermal stability and proton conductivity. Under hydrothermal conditions, these composites can form new phases like CsH5(PO4)2, which contribute to improved performance in fuel cells.
Common Problem
How does caesium dihydrogen phosphate conduct protons?
CsH2PO4 conducts protons through a combination of the vehicle transport mechanism and the Grotthuss mechanism, with phosphate ion reorientation playing a crucial role.
What is the superprotonic phase transition in CsH2PO4?
The superprotonic phase transition is a structural change that occurs at approximately 230°C, resulting in a dramatic increase in proton conductivity[“].
How does temperature affect the conductivity of CsH2PO4?
Temperature significantly affects CsH2PO4's conductivity, with a sharp increase observed at the superprotonic phase transition temperature.
Can the mechanism of action of CsH2PO4 be modified?
Yes, the mechanism can be modified through doping with various elements, nanostructuring, and controlling environmental conditions such as humidity[“].
What role does water play in the proton conduction of CsH2PO4?
Water plays a crucial role in maintaining the high-conductivity superprotonic phase and participates in the vehicle transport mechanism of proton conduction[“].
Eigenschaften
CAS-Nummer |
18649-05-3 |
---|---|
Molekularformel |
CsH2PO4 CsH2O4P |
Molekulargewicht |
229.893 g/mol |
IUPAC-Name |
cesium;dihydrogen phosphate |
InChI |
InChI=1S/Cs.H3O4P/c;1-5(2,3)4/h;(H3,1,2,3,4)/q+1;/p-1 |
InChI-Schlüssel |
QPTUMKXXAAHOOE-UHFFFAOYSA-M |
SMILES |
OP(=O)(O)[O-].[Cs+] |
Kanonische SMILES |
[H+].[H+].[O-]P(=O)([O-])[O-].[Cs+] |
Herkunft des Produkts |
United States |
Haftungsausschluss und Informationen zu In-Vitro-Forschungsprodukten
Bitte beachten Sie, dass alle Artikel und Produktinformationen, die auf BenchChem präsentiert werden, ausschließlich zu Informationszwecken bestimmt sind. Die auf BenchChem zum Kauf angebotenen Produkte sind speziell für In-vitro-Studien konzipiert, die außerhalb lebender Organismen durchgeführt werden. In-vitro-Studien, abgeleitet von dem lateinischen Begriff "in Glas", beinhalten Experimente, die in kontrollierten Laborumgebungen unter Verwendung von Zellen oder Geweben durchgeführt werden. Es ist wichtig zu beachten, dass diese Produkte nicht als Arzneimittel oder Medikamente eingestuft sind und keine Zulassung der FDA für die Vorbeugung, Behandlung oder Heilung von medizinischen Zuständen, Beschwerden oder Krankheiten erhalten haben. Wir müssen betonen, dass jede Form der körperlichen Einführung dieser Produkte in Menschen oder Tiere gesetzlich strikt untersagt ist. Es ist unerlässlich, sich an diese Richtlinien zu halten, um die Einhaltung rechtlicher und ethischer Standards in Forschung und Experiment zu gewährleisten.