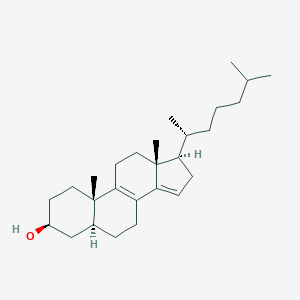
5alpha-Cholesta-8,14-dien-3beta-ol
Übersicht
Beschreibung
Wissenschaftliche Forschungsanwendungen
14-Dehydrozymostenol hat eine breite Palette von Anwendungen in der wissenschaftlichen Forschung:
Chemie: Als Vorläufer bei der Synthese komplexer Sterolverbindungen verwendet.
Biologie: Spielt eine Rolle bei der Untersuchung der Cholesterinbiosynthese und des Cholesterinstoffwechsels.
Medizin: Untersucht auf seine potenziellen therapeutischen Wirkungen bei der Behandlung von Cholesterin-bedingten Erkrankungen.
Industrie: Wird bei der Herstellung von sterolbasierten Produkten und Nahrungsergänzungsmitteln eingesetzt
5. Wirkmechanismus
Der Wirkmechanismus von 14-Dehydrozymostenol beinhaltet seine Umwandlung in Cholesterin durch eine Reihe von enzymatischen Reaktionen. Es zielt auf bestimmte Enzyme im Cholesterinbiosyntheseweg ab und erleichtert die Bildung von Cholesterin aus Sterolzwischenprodukten . Dieser Prozess ist entscheidend für die Aufrechterhaltung der Zellfunktionen und der allgemeinen Gesundheit.
Ähnliche Verbindungen:
- Zymosterol
- Lathosterol
- Desmosterol
- 7-Dehydrocholesterin
Vergleich: 14-Dehydrozymostenol ist aufgrund seiner spezifischen Rolle im Cholesterinbiosyntheseweg einzigartig. Im Gegensatz zu anderen Sterolzwischenprodukten unterliegt es unterschiedlichen enzymatischen Reaktionen, die für die Bildung von Cholesterin entscheidend sind . Seine Strukturmerkmale und Reaktivität unterscheiden es auch von ähnlichen Verbindungen .
Wirkmechanismus
Target of Action
5alpha-Cholesta-8,14-dien-3beta-ol is a 3β-sterol and an efficient precursor of cholesterol . It primarily targets the enzyme Delta(14)-sterol reductase , which belongs to the family of oxidoreductases . These enzymes act on the CH-CH group of donors with NAD+ or NADP+ as acceptors .
Mode of Action
The compound interacts with its target enzyme, Delta(14)-sterol reductase, to facilitate the conversion of 4,4-dimethyl-5alpha-cholesta-8,24-dien-3beta-ol to 4,4-dimethyl-5alpha-cholesta-8,14,24-trien-3beta-ol . This reaction involves the reduction of a double bond in the sterol molecule, aided by the transfer of hydrogen ions from NADPH .
Biochemical Pathways
This compound participates in the biosynthesis of steroids . The conversion of this compound to cholesterol under aerobic conditions is a crucial step in the steroid biosynthesis pathway. The resulting cholesterol is a vital component of cell membranes and a precursor for the synthesis of other important steroids.
Pharmacokinetics
Its conversion to cholesterol under aerobic conditions suggests that oxygen availability may impact its bioavailability.
Result of Action
The primary result of the action of this compound is the production of cholesterol . Cholesterol is a fundamental component of cell membranes, contributing to membrane fluidity and integrity. It is also a precursor for the synthesis of vital biomolecules, including steroid hormones, bile acids, and vitamin D.
Action Environment
The action of this compound is influenced by environmental factors such as oxygen availability, as its conversion to cholesterol occurs under aerobic conditions . Additionally, factors that affect the function of the liver, where cholesterol synthesis and metabolism primarily occur, could also influence the action, efficacy, and stability of this compound.
Biochemische Analyse
Biochemical Properties
5alpha-Cholesta-8,14-dien-3beta-ol plays a significant role in biochemical reactions. It interacts with various enzymes, proteins, and other biomolecules. For instance, it is involved in the enzymatic conversion process catalyzed by Delta14-sterol reductase . This enzyme catalyzes the three-step oxidative removal of the 14alpha-methyl group of sterols such as lanosterol and 24,25-dihydrolanosterol .
Molecular Mechanism
The molecular mechanism of action of this compound involves its conversion to cholesterol under aerobic conditions . This conversion is facilitated by the enzyme Delta14-sterol reductase . The enzyme catalyzes the three-step oxidative removal of the 14alpha-methyl group of sterols, converting them to intermediates of cholesterol biosynthesis .
Metabolic Pathways
This compound is involved in the cholesterol biosynthesis pathway . It is converted to cholesterol under aerobic conditions, a process facilitated by the enzyme Delta14-sterol reductase .
Vorbereitungsmethoden
Synthesewege und Reaktionsbedingungen: 14-Dehydrozymostenol kann durch verschiedene chemische Reaktionen synthetisiert werden, an denen Sterolzwischenprodukte beteiligt sind. Eine übliche Methode beinhaltet die enzymatische Umwandlung von 5α-Cholesta-8,14-dien-3β-ol zu Cholesterin . Der Prozess umfasst typischerweise Schritte wie Carboxylierung, Dehydrierung und Isomerisierung .
Industrielle Produktionsmethoden: Die industrielle Produktion von 14-Dehydrozymostenol beinhaltet den Einsatz fortschrittlicher biochemischer Techniken, um eine hohe Reinheit und Ausbeute zu gewährleisten. Die Verbindung wird oft in Pulverform hergestellt und bei niedrigen Temperaturen gelagert, um die Stabilität zu erhalten .
Analyse Chemischer Reaktionen
Reaktionstypen: 14-Dehydrozymostenol unterliegt verschiedenen chemischen Reaktionen, darunter:
Oxidation: Umwandlung in andere Sterolzwischenprodukte.
Reduktion: Bildung verschiedener Sterolderivate.
Substitution: Einführung von funktionellen Gruppen zur Modifizierung seiner Struktur.
Häufige Reagenzien und Bedingungen:
Oxidation: Reagenzien wie Kaliumpermanganat oder Chromtrioxid.
Reduktion: Reagenzien wie Natriumborhydrid oder Lithiumaluminiumhydrid.
Substitution: Bedingungen unter Verwendung von Katalysatoren wie Palladium oder Platin.
Hauptprodukte, die gebildet werden: Die Hauptprodukte, die aus diesen Reaktionen gebildet werden, umfassen verschiedene Sterolzwischenprodukte und -derivate, die für weitere biochemische Prozesse von entscheidender Bedeutung sind .
Vergleich Mit ähnlichen Verbindungen
- Zymosterol
- Lathosterol
- Desmosterol
- 7-dehydrocholesterol
Comparison: 14-dehydro Zymostenol is unique due to its specific role in the cholesterol biosynthesis pathway. Unlike other sterol intermediates, it undergoes distinct enzymatic reactions that are critical for the formation of cholesterol . Its structural features and reactivity also set it apart from similar compounds .
Eigenschaften
IUPAC Name |
(3S,5S,10S,13R,17R)-10,13-dimethyl-17-[(2R)-6-methylheptan-2-yl]-2,3,4,5,6,7,11,12,16,17-decahydro-1H-cyclopenta[a]phenanthren-3-ol | |
---|---|---|
Source | PubChem | |
URL | https://pubchem.ncbi.nlm.nih.gov | |
Description | Data deposited in or computed by PubChem | |
InChI |
InChI=1S/C27H44O/c1-18(2)7-6-8-19(3)23-11-12-24-22-10-9-20-17-21(28)13-15-26(20,4)25(22)14-16-27(23,24)5/h12,18-21,23,28H,6-11,13-17H2,1-5H3/t19-,20+,21+,23-,26+,27-/m1/s1 | |
Source | PubChem | |
URL | https://pubchem.ncbi.nlm.nih.gov | |
Description | Data deposited in or computed by PubChem | |
InChI Key |
AWBZPJQUWZBRII-CXDHQSPESA-N | |
Source | PubChem | |
URL | https://pubchem.ncbi.nlm.nih.gov | |
Description | Data deposited in or computed by PubChem | |
Canonical SMILES |
CC(C)CCCC(C)C1CC=C2C1(CCC3=C2CCC4C3(CCC(C4)O)C)C | |
Source | PubChem | |
URL | https://pubchem.ncbi.nlm.nih.gov | |
Description | Data deposited in or computed by PubChem | |
Isomeric SMILES |
C[C@H](CCCC(C)C)[C@H]1CC=C2[C@@]1(CCC3=C2CC[C@@H]4[C@@]3(CC[C@@H](C4)O)C)C | |
Source | PubChem | |
URL | https://pubchem.ncbi.nlm.nih.gov | |
Description | Data deposited in or computed by PubChem | |
Molecular Formula |
C27H44O | |
Source | PubChem | |
URL | https://pubchem.ncbi.nlm.nih.gov | |
Description | Data deposited in or computed by PubChem | |
Molecular Weight |
384.6 g/mol | |
Source | PubChem | |
URL | https://pubchem.ncbi.nlm.nih.gov | |
Description | Data deposited in or computed by PubChem | |
Retrosynthesis Analysis
AI-Powered Synthesis Planning: Our tool employs the Template_relevance Pistachio, Template_relevance Bkms_metabolic, Template_relevance Pistachio_ringbreaker, Template_relevance Reaxys, Template_relevance Reaxys_biocatalysis model, leveraging a vast database of chemical reactions to predict feasible synthetic routes.
One-Step Synthesis Focus: Specifically designed for one-step synthesis, it provides concise and direct routes for your target compounds, streamlining the synthesis process.
Accurate Predictions: Utilizing the extensive PISTACHIO, BKMS_METABOLIC, PISTACHIO_RINGBREAKER, REAXYS, REAXYS_BIOCATALYSIS database, our tool offers high-accuracy predictions, reflecting the latest in chemical research and data.
Strategy Settings
Precursor scoring | Relevance Heuristic |
---|---|
Min. plausibility | 0.01 |
Model | Template_relevance |
Template Set | Pistachio/Bkms_metabolic/Pistachio_ringbreaker/Reaxys/Reaxys_biocatalysis |
Top-N result to add to graph | 6 |
Feasible Synthetic Routes
Haftungsausschluss und Informationen zu In-Vitro-Forschungsprodukten
Bitte beachten Sie, dass alle Artikel und Produktinformationen, die auf BenchChem präsentiert werden, ausschließlich zu Informationszwecken bestimmt sind. Die auf BenchChem zum Kauf angebotenen Produkte sind speziell für In-vitro-Studien konzipiert, die außerhalb lebender Organismen durchgeführt werden. In-vitro-Studien, abgeleitet von dem lateinischen Begriff "in Glas", beinhalten Experimente, die in kontrollierten Laborumgebungen unter Verwendung von Zellen oder Geweben durchgeführt werden. Es ist wichtig zu beachten, dass diese Produkte nicht als Arzneimittel oder Medikamente eingestuft sind und keine Zulassung der FDA für die Vorbeugung, Behandlung oder Heilung von medizinischen Zuständen, Beschwerden oder Krankheiten erhalten haben. Wir müssen betonen, dass jede Form der körperlichen Einführung dieser Produkte in Menschen oder Tiere gesetzlich strikt untersagt ist. Es ist unerlässlich, sich an diese Richtlinien zu halten, um die Einhaltung rechtlicher und ethischer Standards in Forschung und Experiment zu gewährleisten.