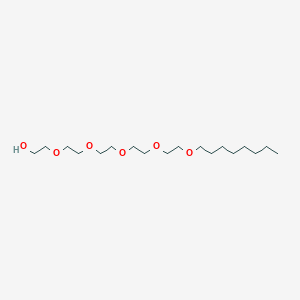
3,6,9,12,15-Pentaoxatricosan-1-ol
Übersicht
Beschreibung
“3,6,9,12,15-Pentaoxatricosan-1-ol” is a small molecule with the molecular formula C18H38O6 . It is also known by other names such as “n-Octylpentaoxyethylene”, “Pentaethylene Glycol Monooctyl Ether”, and "Pentaoxyethylene Monooctyl Ether" . It belongs to the class of organic compounds known as polyethylene glycols, which are oligomers or polymers of ethylene oxide, with the general formula (C2H4O)n (with n>=3) .
Molecular Structure Analysis
The molecular weight of “3,6,9,12,15-Pentaoxatricosan-1-ol” is 350.5 g/mol . The InChI representation of the molecule isInChI=1S/C18H38O6/c1-2-3-4-5-6-7-9-20-11-13-22-15-17-24-18-16-23-14-12-21-10-8-19/h19H,2-18H2,1H3
. The Canonical SMILES representation is CCCCCCCCOCCOCCOCCOCCOCCO
. Physical And Chemical Properties Analysis
The molecule has a Hydrogen Bond Donor Count of 1 and a Hydrogen Bond Acceptor Count of 6 . It has a Rotatable Bond Count of 21 . The Exact Mass of the molecule is 350.26683893 g/mol . The XLogP3 of the molecule is 2.3 .Wissenschaftliche Forschungsanwendungen
Drug Delivery Systems
n-Octylpentaoxyethylene: has properties that make it suitable for use in drug delivery systems. Its ability to form micelles and encapsulate drugs can be utilized to improve the solubility and bioavailability of hydrophobic drugs . This application is particularly valuable in the development of new pharmaceutical formulations where drug solubility poses a significant challenge.
Surface Active Agent
Due to its surfactant nature, n-Octylpentaoxyethylene can be used as a surface active agent in scientific research. It can reduce surface tension, which is beneficial in processes like emulsification, wetting, and dispersion of materials . This property is essential in the formulation of coatings, paints, and inks.
Biochemical Research
In biochemical research, n-Octylpentaoxyethylene can be used to solubilize proteins and other biomolecules, making it easier to study their structure and function. It can also be used in the purification process of proteins by acting as a mild detergent that preserves protein integrity .
Nanotechnology
n-Octylpentaoxyethylene: can be used in the synthesis of nanoparticles. Its surfactant properties help in stabilizing nanoparticles, preventing aggregation, and controlling particle size during synthesis. This is crucial for the production of uniform nanoparticles with specific properties for various applications .
Cosmetics and Personal Care
In the cosmetics industry, n-Octylpentaoxyethylene can be used as an emulsifier to stabilize products like creams and lotions. Its ability to mix oil and water phases makes it an important ingredient in personal care products .
Environmental Research
n-Octylpentaoxyethylene: can be applied in environmental research, particularly in the study of soil and water pollution. It can act as a tracer or carrier for pollutants, helping researchers understand the movement and distribution of contaminants in the environment .
Food Industry
This compound can be used in the food industry as an additive to modify the texture and stability of food products. Its surfactant properties can improve the shelf-life and consistency of processed foods .
Lubricants and Hydraulic Fluids
In mechanical and industrial applications, n-Octylpentaoxyethylene can serve as a component in lubricants and hydraulic fluids. Its properties can enhance the performance and longevity of machinery by reducing friction and wear .
Wirkmechanismus
Target of Action
3,6,9,12,15-Pentaoxatricosan-1-ol, also known as n-Octylpentaoxyethylene, interacts with several targets. The primary targets include Dihydroorotate dehydrogenase (quinone), mitochondrial in humans , Fatty acid oxidation complex subunit alpha and 3-ketoacyl-CoA thiolase in Pseudomonas fragi , and Ferripyoverdine receptor in Pseudomonas aeruginosa .
Mode of Action
It is known to interact with its targets, leading to changes in their function
Biochemical Pathways
Given its interaction with the fatty acid oxidation complex and 3-ketoacyl-CoA thiolase, it may influence fatty acid metabolism in Pseudomonas fragi
Result of Action
Given its interaction with various targets, it is likely to have multiple effects at the molecular and cellular levels .
Eigenschaften
IUPAC Name |
2-[2-[2-[2-(2-octoxyethoxy)ethoxy]ethoxy]ethoxy]ethanol | |
---|---|---|
Source | PubChem | |
URL | https://pubchem.ncbi.nlm.nih.gov | |
Description | Data deposited in or computed by PubChem | |
InChI |
InChI=1S/C18H38O6/c1-2-3-4-5-6-7-9-20-11-13-22-15-17-24-18-16-23-14-12-21-10-8-19/h19H,2-18H2,1H3 | |
Source | PubChem | |
URL | https://pubchem.ncbi.nlm.nih.gov | |
Description | Data deposited in or computed by PubChem | |
InChI Key |
MJELOWOAIAAUJT-UHFFFAOYSA-N | |
Source | PubChem | |
URL | https://pubchem.ncbi.nlm.nih.gov | |
Description | Data deposited in or computed by PubChem | |
Canonical SMILES |
CCCCCCCCOCCOCCOCCOCCOCCO | |
Source | PubChem | |
URL | https://pubchem.ncbi.nlm.nih.gov | |
Description | Data deposited in or computed by PubChem | |
Molecular Formula |
C18H38O6 | |
Source | PubChem | |
URL | https://pubchem.ncbi.nlm.nih.gov | |
Description | Data deposited in or computed by PubChem | |
DSSTOX Substance ID |
DTXSID6075192 | |
Record name | 3,6,9,12,15-Pentaoxatricosan-1-ol | |
Source | EPA DSSTox | |
URL | https://comptox.epa.gov/dashboard/DTXSID6075192 | |
Description | DSSTox provides a high quality public chemistry resource for supporting improved predictive toxicology. | |
Molecular Weight |
350.5 g/mol | |
Source | PubChem | |
URL | https://pubchem.ncbi.nlm.nih.gov | |
Description | Data deposited in or computed by PubChem | |
Product Name |
3,6,9,12,15-Pentaoxatricosan-1-ol | |
CAS RN |
19327-40-3 | |
Record name | C8E5 | |
Source | CAS Common Chemistry | |
URL | https://commonchemistry.cas.org/detail?cas_rn=19327-40-3 | |
Description | CAS Common Chemistry is an open community resource for accessing chemical information. Nearly 500,000 chemical substances from CAS REGISTRY cover areas of community interest, including common and frequently regulated chemicals, and those relevant to high school and undergraduate chemistry classes. This chemical information, curated by our expert scientists, is provided in alignment with our mission as a division of the American Chemical Society. | |
Explanation | The data from CAS Common Chemistry is provided under a CC-BY-NC 4.0 license, unless otherwise stated. | |
Record name | n-Octylpentaoxyethylene | |
Source | ChemIDplus | |
URL | https://pubchem.ncbi.nlm.nih.gov/substance/?source=chemidplus&sourceid=0019327403 | |
Description | ChemIDplus is a free, web search system that provides access to the structure and nomenclature authority files used for the identification of chemical substances cited in National Library of Medicine (NLM) databases, including the TOXNET system. | |
Record name | 3,6,9,12,15-Pentaoxatricosan-1-ol | |
Source | EPA DSSTox | |
URL | https://comptox.epa.gov/dashboard/DTXSID6075192 | |
Description | DSSTox provides a high quality public chemistry resource for supporting improved predictive toxicology. | |
Record name | 2-[2-[2-[2-(2-octoxyethoxy)ethoxy]ethoxy]ethoxy]ethanol | |
Source | European Chemicals Agency (ECHA) | |
URL | https://echa.europa.eu/information-on-chemicals | |
Description | The European Chemicals Agency (ECHA) is an agency of the European Union which is the driving force among regulatory authorities in implementing the EU's groundbreaking chemicals legislation for the benefit of human health and the environment as well as for innovation and competitiveness. | |
Explanation | Use of the information, documents and data from the ECHA website is subject to the terms and conditions of this Legal Notice, and subject to other binding limitations provided for under applicable law, the information, documents and data made available on the ECHA website may be reproduced, distributed and/or used, totally or in part, for non-commercial purposes provided that ECHA is acknowledged as the source: "Source: European Chemicals Agency, http://echa.europa.eu/". Such acknowledgement must be included in each copy of the material. ECHA permits and encourages organisations and individuals to create links to the ECHA website under the following cumulative conditions: Links can only be made to webpages that provide a link to the Legal Notice page. | |
Retrosynthesis Analysis
AI-Powered Synthesis Planning: Our tool employs the Template_relevance Pistachio, Template_relevance Bkms_metabolic, Template_relevance Pistachio_ringbreaker, Template_relevance Reaxys, Template_relevance Reaxys_biocatalysis model, leveraging a vast database of chemical reactions to predict feasible synthetic routes.
One-Step Synthesis Focus: Specifically designed for one-step synthesis, it provides concise and direct routes for your target compounds, streamlining the synthesis process.
Accurate Predictions: Utilizing the extensive PISTACHIO, BKMS_METABOLIC, PISTACHIO_RINGBREAKER, REAXYS, REAXYS_BIOCATALYSIS database, our tool offers high-accuracy predictions, reflecting the latest in chemical research and data.
Strategy Settings
Precursor scoring | Relevance Heuristic |
---|---|
Min. plausibility | 0.01 |
Model | Template_relevance |
Template Set | Pistachio/Bkms_metabolic/Pistachio_ringbreaker/Reaxys/Reaxys_biocatalysis |
Top-N result to add to graph | 6 |
Feasible Synthetic Routes
Q & A
Q1: How does n-Octylpentaoxyethylene behave in aqueous solutions, and how does temperature impact this behavior?
A1: n-Octylpentaoxyethylene forms micelles in aqueous solutions above a critical concentration (CMC). Interestingly, the microstructure of these micelles is sensitive to temperature. At room temperature, C8E5 forms globular micelles, maintaining this shape even with increasing urea concentration. [] This behavior contrasts with structurally similar surfactants like C8E4 and C12E6, which transition from elongated to globular micelles with increased urea or decreased temperature. [] Additionally, C8E5 solutions exhibit a cloud point phenomenon, where the solution transitions from clear to cloudy upon heating past a specific temperature (cloud point temperature). This clouding arises from the temperature-dependent interactions between micelles, influenced by the hydration of the polyethylene oxide headgroup. []
Q2: How does the Baxter model help understand the phase behavior of n-Octylpentaoxyethylene solutions?
A2: The Baxter model, which describes the statistical mechanics of particles interacting via a hard-sphere repulsion with surface adhesion, offers valuable insights into the phase behavior of C8E5 solutions. [] This model proves particularly relevant for colloidal systems like nonionic surfactant solutions, where Coulombic interactions are negligible. [] The Baxter model accurately predicts the low critical volume fraction (φc = 0.12) observed in these solutions. [] Moreover, it demonstrates that the interaction parameter (U/kT) is a slowly varying function, aligning with experimental observations and contrasting with the unphysically high values predicted by other models like the Mean Spherical Approximation (MSA). []
Haftungsausschluss und Informationen zu In-Vitro-Forschungsprodukten
Bitte beachten Sie, dass alle Artikel und Produktinformationen, die auf BenchChem präsentiert werden, ausschließlich zu Informationszwecken bestimmt sind. Die auf BenchChem zum Kauf angebotenen Produkte sind speziell für In-vitro-Studien konzipiert, die außerhalb lebender Organismen durchgeführt werden. In-vitro-Studien, abgeleitet von dem lateinischen Begriff "in Glas", beinhalten Experimente, die in kontrollierten Laborumgebungen unter Verwendung von Zellen oder Geweben durchgeführt werden. Es ist wichtig zu beachten, dass diese Produkte nicht als Arzneimittel oder Medikamente eingestuft sind und keine Zulassung der FDA für die Vorbeugung, Behandlung oder Heilung von medizinischen Zuständen, Beschwerden oder Krankheiten erhalten haben. Wir müssen betonen, dass jede Form der körperlichen Einführung dieser Produkte in Menschen oder Tiere gesetzlich strikt untersagt ist. Es ist unerlässlich, sich an diese Richtlinien zu halten, um die Einhaltung rechtlicher und ethischer Standards in Forschung und Experiment zu gewährleisten.