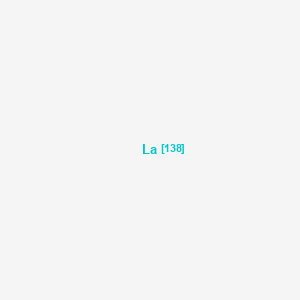
Lanthanum-138
Übersicht
Beschreibung
Lanthanum-138 is an isotope of Lanthanum . Lanthanum is a chemical element with the symbol “La” and atomic number 57 . It belongs to the lanthanide series and is classified as a rare-earth element . Lanthanum-138 has an atomic number of 57, which means it has 57 protons, and a mass number of 138, indicating the number of protons and neutrons combined . Therefore, Lanthanum-138 has 81 neutrons .
Molecular Structure Analysis
The molecular structure of Lanthanum-138 can be represented by the symbol 138 La or 13857 La . The isotopic mass of Lanthanum-138 is 137.907118 (3) u . The nuclide mass, which is the calculated nuclear mass without electrons, is 137.8758511 u .
Chemical Reactions Analysis
Lanthanum reacts with atmospheric oxygen to form lanthanum oxide (La₂O₃). This is generally a slow oxidation process that occurs at room temperature . Lanthanum also reacts slowly with cold water, and quickly with hot water, forming lanthanum(III) hydroxide, La(OH) 3, and hydrogen gas (H 2) .
Physical And Chemical Properties Analysis
Lanthanum is a soft, ductile, silvery-white metal . It has a melting point of 920°C, a boiling point of 3464°C, and a density of 6.15 g/cm³ at 20°C . Lanthanum-138 has a mass excess of -86.51903 MeV and a nuclear binding energy of 1155.76703453 MeV per nucleus .
Wissenschaftliche Forschungsanwendungen
Isotopic Composition and Atomic Weight
Lanthanum's isotopic composition, specifically Lanthanum-138, has been a subject of detailed study. Laeter and Bukilic (2005) conducted high-precision measurements using a thermal ionization mass spectrometer to determine the isotopic composition of lanthanum, essential for calculating its atomic weight. This research is significant as Lanthanum-138 is the parent nuclide of geochronometers 138La-138Ba and 138La-138Ce, crucial for p-process nucleosynthetic calculations (Laeter & Bukilic, 2005).
Quadrupole Moment Determination
Fischer et al. (1972) enriched Lanthanum-138 and used optical interference spectroscopy to determine its nuclear electric quadrupole moment. This kind of research provides fundamental insights into the nuclear properties of rare isotopes like Lanthanum-138 (Fischer, Hühnermann, Mandrek, & Ihle, 1972).
Geochronology Applications
Tanaka and Masuda (1982) utilized Lanthanum-138 in a new dating method, the La-Ce geochronometer. They demonstrated the first age determination using La-Ce dating, showcasing Lanthanum-138's application in geochronology and petrogenesis (Tanaka & Masuda, 1982).
Hyperfine Structure Analysis
Benton, Cooke, and Griffith (1994) performed laser spectroscopy on a thermal atomic beam of natural lanthanum, revealing the hyperfine structure associated with Lanthanum-138. Their findings contribute to the understanding of atomic and nuclear physics (Benton, Cooke, & Griffith, 1994).
Fission Product Monitoring
Knight et al. (1984) explored the use of Lanthanum-138 as a fission monitor for determining fissions and burnup in thorium-uranium dioxide fuels. This application is critical for nuclear fuel cycle management and reactor operation (Knight, Cassidy, Recoskie, & Green, 1984).
La–Ce Dating in Geological Studies
Dickin (1987) conducted La-Ce isotope scheme studies, using Lanthanum-138, for petrogenetic analysis. This research aids in understanding geological processes and the age of rock formations (Dickin, 1987).
Nuclear Magnetic Resonance Spectroscopy
Krüger, Lutz, and Oehler (1977) provided data on nuclear magnetic moments and ratios of quadrupole moments for Lanthanum-138 using NMR spectroscopy. This research contributes to a better understanding of nuclear properties (Krüger, Lutz, & Oehler, 1977).
Solid-State NMR Spectroscopy of Lanthanum Compounds
Willans et al. (2005) investigated lanthanum coordination compounds using solid-state NMR spectroscopy, providing insight into the molecular structure of these compounds (Willans, Feindel, Ooms, & Wasylishen, 2005).
Wirkmechanismus
Lanthanum-138, like other isotopes of Lanthanum, can form insoluble lanthanum phosphate complexes that pass through the gastrointestinal (GI) tract unabsorbed . This property is used in medical applications where Lanthanum is used as a phosphate binder to reduce serum phosphate in patients with end-stage renal disease .
Safety and Hazards
Lanthanum will burn under fire conditions and may react with water under fire conditions liberating flammable hydrogen gas . It may emit fumes of lanthanum oxide under fire conditions . Special protective equipment and precautions for firefighters include full face, self-contained breathing apparatus and full protective clothing to prevent contact with skin and eyes .
Eigenschaften
IUPAC Name |
lanthanum-138 | |
---|---|---|
Source | PubChem | |
URL | https://pubchem.ncbi.nlm.nih.gov | |
Description | Data deposited in or computed by PubChem | |
InChI |
InChI=1S/La/i1-1 | |
Source | PubChem | |
URL | https://pubchem.ncbi.nlm.nih.gov | |
Description | Data deposited in or computed by PubChem | |
InChI Key |
FZLIPJUXYLNCLC-BJUDXGSMSA-N | |
Source | PubChem | |
URL | https://pubchem.ncbi.nlm.nih.gov | |
Description | Data deposited in or computed by PubChem | |
Canonical SMILES |
[La] | |
Source | PubChem | |
URL | https://pubchem.ncbi.nlm.nih.gov | |
Description | Data deposited in or computed by PubChem | |
Isomeric SMILES |
[138La] | |
Source | PubChem | |
URL | https://pubchem.ncbi.nlm.nih.gov | |
Description | Data deposited in or computed by PubChem | |
Molecular Formula |
La | |
Source | PubChem | |
URL | https://pubchem.ncbi.nlm.nih.gov | |
Description | Data deposited in or computed by PubChem | |
DSSTOX Substance ID |
DTXSID40935867 | |
Record name | (~138~La)Lanthanum | |
Source | EPA DSSTox | |
URL | https://comptox.epa.gov/dashboard/DTXSID40935867 | |
Description | DSSTox provides a high quality public chemistry resource for supporting improved predictive toxicology. | |
Molecular Weight |
137.907124 g/mol | |
Source | PubChem | |
URL | https://pubchem.ncbi.nlm.nih.gov | |
Description | Data deposited in or computed by PubChem | |
Product Name |
Lanthanum-138 | |
CAS RN |
15816-87-2 | |
Record name | Lanthanum, isotope of mass 138 | |
Source | ChemIDplus | |
URL | https://pubchem.ncbi.nlm.nih.gov/substance/?source=chemidplus&sourceid=0015816872 | |
Description | ChemIDplus is a free, web search system that provides access to the structure and nomenclature authority files used for the identification of chemical substances cited in National Library of Medicine (NLM) databases, including the TOXNET system. | |
Record name | (~138~La)Lanthanum | |
Source | EPA DSSTox | |
URL | https://comptox.epa.gov/dashboard/DTXSID40935867 | |
Description | DSSTox provides a high quality public chemistry resource for supporting improved predictive toxicology. | |
Synthesis routes and methods I
Procedure details
Synthesis routes and methods II
Procedure details
Haftungsausschluss und Informationen zu In-Vitro-Forschungsprodukten
Bitte beachten Sie, dass alle Artikel und Produktinformationen, die auf BenchChem präsentiert werden, ausschließlich zu Informationszwecken bestimmt sind. Die auf BenchChem zum Kauf angebotenen Produkte sind speziell für In-vitro-Studien konzipiert, die außerhalb lebender Organismen durchgeführt werden. In-vitro-Studien, abgeleitet von dem lateinischen Begriff "in Glas", beinhalten Experimente, die in kontrollierten Laborumgebungen unter Verwendung von Zellen oder Geweben durchgeführt werden. Es ist wichtig zu beachten, dass diese Produkte nicht als Arzneimittel oder Medikamente eingestuft sind und keine Zulassung der FDA für die Vorbeugung, Behandlung oder Heilung von medizinischen Zuständen, Beschwerden oder Krankheiten erhalten haben. Wir müssen betonen, dass jede Form der körperlichen Einführung dieser Produkte in Menschen oder Tiere gesetzlich strikt untersagt ist. Es ist unerlässlich, sich an diese Richtlinien zu halten, um die Einhaltung rechtlicher und ethischer Standards in Forschung und Experiment zu gewährleisten.