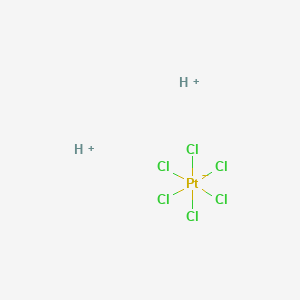
Hexachloroplatinsäure
Übersicht
Beschreibung
Hexachloroplatinic acid, also known as chloroplatinic acid, is an inorganic compound with the chemical formula [H₃O]₂PtCl₆ₓ (0 ≤ x ≤ 6). It is a red solid and serves as an important commercial source of platinum, typically available as an aqueous solution. This compound is highly hygroscopic and is often used in various industrial and scientific applications .
Wissenschaftliche Forschungsanwendungen
Hexachloroplatinic acid has numerous applications in scientific research:
-
Catalysis: : It is used as a precursor for synthesizing platinum catalysts, which are employed in various chemical reactions, including hydrogenation and oxidation .
-
Nanoparticle Synthesis: : It serves as a platinum precursor in the preparation of platinum nanoparticles, which are used in catalysis, fuel cells, and environmental applications .
-
Electroplating: : Hexachloroplatinic acid is used in platinum-plating baths for electroplating applications .
-
Analytical Chemistry: : It is used for the quantitative analysis of potassium by selective precipitation as potassium chloroplatinate .
Wirkmechanismus
Target of Action
Hexachloroplatinic acid is primarily used as a precursor for the synthesis of various platinum compounds . It is also used in platinum-plating baths .
Mode of Action
Hexachloroplatinic acid is a very effective homogeneous transition metal catalyst . It is often used in the hydrosilylation of olefins, an important reaction for the production of various organosilicon compounds . The compound is also used in the synthesis of Pt/C catalyst for polymer electrolyte membrane fuel cell applications and Pt/TiO2 catalyst to study the photocatalytic degradation pathway of methylene blue .
Biochemical Pathways
The compound is involved in the hydrosilylation reaction, which enables the addition of silicon hydrides across CC multiple bonds . This reaction is an efficient method for the formation of organosilicon compounds and represents one of the most important reactions in silicon chemistry .
Pharmacokinetics
Hexachloroplatinic acid is highly soluble in water , which can impact its bioavailability.
Result of Action
The result of hexachloroplatinic acid’s action is the formation of various platinum compounds and organosilicon compounds . These compounds have wide-ranging applications in industries such as electronics, catalysis, and medicine.
Action Environment
Environmental factors can influence the action, efficacy, and stability of hexachloroplatinic acid. For instance, it is highly hygroscopic , meaning it readily absorbs moisture from the environment. This property can affect its stability and efficacy in certain applications. Furthermore, its release into the environment at elevated concentrations due to its widespread use in many industrial applications could potentially have adverse effects on human and environmental health .
Biochemische Analyse
Biochemical Properties
It is known that Hexachloroplatinic acid can interact with various enzymes and proteins, potentially influencing their function
Cellular Effects
Some studies suggest that it may have cytotoxic effects on certain cell lines . It is also suggested that Hexachloroplatinic acid may influence cell function, potentially impacting cell signaling pathways, gene expression, and cellular metabolism .
Molecular Mechanism
It is thought to exert its effects at the molecular level through binding interactions with biomolecules, potentially leading to enzyme inhibition or activation, and changes in gene expression .
Temporal Effects in Laboratory Settings
It is known that Hexachloroplatinic acid is highly soluble in water and decomposes upon boiling
Vorbereitungsmethoden
Hexachloroplatinic acid can be synthesized through several methods:
-
Dissolution in Aqua Regia: : The most common method involves dissolving platinum metal in aqua regia, a mixture of nitric acid and hydrochloric acid. The reaction can be represented as: [ \text{Pt} + 4 \text{HNO}_3 + 6 \text{HCl} \rightarrow \text{H}_2\text{PtCl}_6 + 4 \text{NO}_2 + 4 \text{H}_2\text{O} ] The resulting orange/red solution can be evaporated to produce brownish-red crystals .
-
Chlorine Gas Exposure: : Another method involves exposing an aqueous suspension of platinum particles to chlorine gas .
-
Electrolysis: : Hexachloroplatinic acid can also be produced via electrolysis .
Analyse Chemischer Reaktionen
Hexachloroplatinic acid undergoes various chemical reactions, including:
-
Decomposition: : When heated, hexachloroplatinic acid decomposes to platinum(IV) chloride: [ (\text{H}_3\text{O})_2\text{PtCl}_6 \cdot n \text{H}_2\text{O} \rightarrow \text{PtCl}_4 + 2 \text{HCl} + (n + 2) \text{H}_2\text{O} ] This reaction occurs at elevated temperatures .
-
Reduction: : Hexachloroplatinic acid can be reduced by hydrogen to form platinum metal. The kinetics of this reduction have been studied extensively .
-
Substitution: : It reacts with various reagents to form different platinum complexes. For example, it reacts with tetraorganylphosphonium and trimethylammonium chlorides to form corresponding hexachloroplatinate complexes .
Vergleich Mit ähnlichen Verbindungen
Hexachloroplatinic acid can be compared with other hexachloroplatinate compounds, such as:
Hexachloropalladic Acid: Similar to hexachloroplatinic acid but contains palladium instead of platinum.
Potassium Hexachloroplatinate: A potassium salt of hexachloroplatinic acid, used in different applications.
Ammonium Hexachloroplatinate: An ammonium salt of hexachloroplatinic acid, also used in various industrial processes.
Hexachloroplatinic acid is unique due to its high hygroscopicity and its role as a primary commercial source of platinum.
Biologische Aktivität
Hexachloroplatinic acid (H₂PtCl₆), also known as chloroplatinic acid, is an inorganic compound with significant biological activity, particularly in the fields of cancer treatment and nanotechnology. This article explores its biological properties, mechanisms of action, and relevant research findings, including data tables and case studies.
Hexachloroplatinic acid is a strong acid that undergoes hydrolysis in aqueous solutions, leading to the formation of various platinum complexes. The coordination chemistry of hexachloroplatinic acid involves interactions with chloride ions and water molecules, affecting its biological activity. The compound can be synthesized from platinum metal through dissolution in aqua regia, resulting in a solution that is highly hygroscopic and soluble in water .
The biological activity of hexachloroplatinic acid primarily stems from its ability to form reactive platinum species that can interact with cellular macromolecules, leading to cytotoxic effects. The mechanism often involves the formation of DNA cross-links, which interfere with replication and transcription processes in cancer cells .
Antitumor Activity
Hexachloroplatinic acid has been studied for its antitumor properties, particularly as a precursor to cisplatin, a widely used chemotherapeutic agent. Research indicates that hexachloroplatinic acid exhibits cytotoxic effects against various cancer cell lines:
- Cytotoxicity Studies : In vitro studies have shown that hexachloroplatinic acid induces cell death in HeLa cells and other cancer cell lines. For instance, a study demonstrated that treatment with hexachloroplatinic acid resulted in a significant reduction in cell viability compared to control groups .
- Comparative Analysis : When comparing the cytotoxic effects of hexachloroplatinic acid and platinum nanoparticles (Pt NPs), it was found that Pt NPs exhibited lower cytotoxicity than hexachloroplatinic acid at equivalent concentrations. For example, at 640 µg/mL concentration, the cell viability for A549 cells treated with hexachloroplatinic acid was markedly lower than those treated with Pt NPs .
Antioxidant Activity
Recent studies have explored the antioxidant properties of hexachloroplatinic acid. It has been shown to possess radical scavenging activity, although this activity is generally lower than that observed for platinum nanoparticles synthesized from it:
- DPPH Scavenging Activity : In tests measuring DPPH radical scavenging ability, hexachloroplatinic acid exhibited a scavenging percentage of 3.36% at 640 µg/mL compared to 52.13% for platinum nanoparticles at the same concentration .
Hemocompatibility
The hemocompatibility of hexachloroplatinic acid has also been evaluated, particularly regarding its potential use in biomedical applications:
- Hemolytic Assays : Studies indicate that while hexachloroplatinic acid can induce hemolysis at certain concentrations, the synthesized platinum nanoparticles derived from it show improved hemocompatibility, making them more suitable for medical applications .
Case Studies and Research Findings
Several studies provide insights into the biological effects of hexachloroplatinic acid:
Study | Cell Line | Treatment Concentration | Cell Viability (%) | Effect Observed |
---|---|---|---|---|
Gao et al. (2007) | HeLa | 100 µg/mL | 45% | Significant cytotoxicity |
Alshatwi et al. (2015) | MCF-7 | 640 µg/mL | 60% | Inhibition of proliferation |
Şahin et al. (2018) | MCF-7 | Various concentrations | <50% at high doses | Induction of apoptosis |
Eigenschaften
IUPAC Name |
hexachloroplatinum(2-);hydron | |
---|---|---|
Source | PubChem | |
URL | https://pubchem.ncbi.nlm.nih.gov | |
Description | Data deposited in or computed by PubChem | |
InChI |
InChI=1S/6ClH.Pt/h6*1H;/q;;;;;;+4/p-4 | |
Source | PubChem | |
URL | https://pubchem.ncbi.nlm.nih.gov | |
Description | Data deposited in or computed by PubChem | |
InChI Key |
GBFHNZZOZWQQPA-UHFFFAOYSA-J | |
Source | PubChem | |
URL | https://pubchem.ncbi.nlm.nih.gov | |
Description | Data deposited in or computed by PubChem | |
Canonical SMILES |
[H+].[H+].Cl[Pt-2](Cl)(Cl)(Cl)(Cl)Cl | |
Source | PubChem | |
URL | https://pubchem.ncbi.nlm.nih.gov | |
Description | Data deposited in or computed by PubChem | |
Molecular Formula |
H2PtCl6, Cl6H2Pt | |
Record name | CHLOROPLATINIC ACID, SOLID | |
Source | CAMEO Chemicals | |
URL | https://cameochemicals.noaa.gov/chemical/390 | |
Description | CAMEO Chemicals is a chemical database designed for people who are involved in hazardous material incident response and planning. CAMEO Chemicals contains a library with thousands of datasheets containing response-related information and recommendations for hazardous materials that are commonly transported, used, or stored in the United States. CAMEO Chemicals was developed by the National Oceanic and Atmospheric Administration's Office of Response and Restoration in partnership with the Environmental Protection Agency's Office of Emergency Management. | |
Explanation | CAMEO Chemicals and all other CAMEO products are available at no charge to those organizations and individuals (recipients) responsible for the safe handling of chemicals. However, some of the chemical data itself is subject to the copyright restrictions of the companies or organizations that provided the data. | |
Source | PubChem | |
URL | https://pubchem.ncbi.nlm.nih.gov | |
Description | Data deposited in or computed by PubChem | |
Molecular Weight |
409.8 g/mol | |
Source | PubChem | |
URL | https://pubchem.ncbi.nlm.nih.gov | |
Description | Data deposited in or computed by PubChem | |
Physical Description |
Chloroplatinic acid, is a reddish-brown solid. It is soluble in water and will yield a mildly acidic solution. It may cause illness from inhalation of the dust and it is irritating to skin and eyes. When heated to high temperatures it may decompose to toxic chloride fumes. It may burn, but may be difficult to ignite. It is used for manufacturing indelible ink and in electroplating processes. | |
Record name | CHLOROPLATINIC ACID, SOLID | |
Source | CAMEO Chemicals | |
URL | https://cameochemicals.noaa.gov/chemical/390 | |
Description | CAMEO Chemicals is a chemical database designed for people who are involved in hazardous material incident response and planning. CAMEO Chemicals contains a library with thousands of datasheets containing response-related information and recommendations for hazardous materials that are commonly transported, used, or stored in the United States. CAMEO Chemicals was developed by the National Oceanic and Atmospheric Administration's Office of Response and Restoration in partnership with the Environmental Protection Agency's Office of Emergency Management. | |
Explanation | CAMEO Chemicals and all other CAMEO products are available at no charge to those organizations and individuals (recipients) responsible for the safe handling of chemicals. However, some of the chemical data itself is subject to the copyright restrictions of the companies or organizations that provided the data. | |
CAS No. |
16941-12-1 | |
Record name | CHLOROPLATINIC ACID, SOLID | |
Source | CAMEO Chemicals | |
URL | https://cameochemicals.noaa.gov/chemical/390 | |
Description | CAMEO Chemicals is a chemical database designed for people who are involved in hazardous material incident response and planning. CAMEO Chemicals contains a library with thousands of datasheets containing response-related information and recommendations for hazardous materials that are commonly transported, used, or stored in the United States. CAMEO Chemicals was developed by the National Oceanic and Atmospheric Administration's Office of Response and Restoration in partnership with the Environmental Protection Agency's Office of Emergency Management. | |
Explanation | CAMEO Chemicals and all other CAMEO products are available at no charge to those organizations and individuals (recipients) responsible for the safe handling of chemicals. However, some of the chemical data itself is subject to the copyright restrictions of the companies or organizations that provided the data. | |
Record name | Platinic chloride | |
Source | ChemIDplus | |
URL | https://pubchem.ncbi.nlm.nih.gov/substance/?source=chemidplus&sourceid=0016941121 | |
Description | ChemIDplus is a free, web search system that provides access to the structure and nomenclature authority files used for the identification of chemical substances cited in National Library of Medicine (NLM) databases, including the TOXNET system. | |
Record name | Hexachloroplatinic acid | |
Source | European Chemicals Agency (ECHA) | |
URL | https://echa.europa.eu/substance-information/-/substanceinfo/100.037.267 | |
Description | The European Chemicals Agency (ECHA) is an agency of the European Union which is the driving force among regulatory authorities in implementing the EU's groundbreaking chemicals legislation for the benefit of human health and the environment as well as for innovation and competitiveness. | |
Explanation | Use of the information, documents and data from the ECHA website is subject to the terms and conditions of this Legal Notice, and subject to other binding limitations provided for under applicable law, the information, documents and data made available on the ECHA website may be reproduced, distributed and/or used, totally or in part, for non-commercial purposes provided that ECHA is acknowledged as the source: "Source: European Chemicals Agency, http://echa.europa.eu/". Such acknowledgement must be included in each copy of the material. ECHA permits and encourages organisations and individuals to create links to the ECHA website under the following cumulative conditions: Links can only be made to webpages that provide a link to the Legal Notice page. | |
A: Hexachloroplatinic acid (H2PtCl6) is widely used as a precursor for synthesizing platinum nanoparticles and supported catalysts. These catalysts find applications in diverse fields, including fuel cells, organic synthesis, and environmental remediation. [, , ]
A: Surprisingly, the number of pyridyl groups alone does not directly correlate to higher complexation capacity. Research shows that Zn(II)-5-pyridyl-10,15,20-tris-(3,4-di-methoxy-phenyl)-porphyrin, with only one pyridyl group, demonstrated a significantly higher removal capacity (98%) for hexachloroplatinic acid compared to Zn(II)-meso-tetrakis-pyridylporphyrin (84%), which has four pyridyl groups. This suggests that steric and electronic factors influenced by surrounding groups play a significant role in determining the coordination behavior. []
A: Solution pH significantly impacts both the adsorption rate and the dispersion of platinum on γ-alumina. Lower acidity (pH values below 1.5) favors deposition by pore volume impregnation, while higher acidity leads to a different adsorption mechanism. This difference in adsorption significantly affects the catalytic properties of the resulting Pt-alumina catalyst for carbon monoxide oxidation. []
A: Yes, hexachloroplatinic acid can be used to synthesize carbon nanotube-supported platinum catalysts. Research shows that using hexachloroplatinic acid in polyethylene glycol medium with carbon nanotubes produces an active and thermally stable catalyst for the hydrosilylation of styrene. This catalyst exhibits high conversion rates, improved β-addition selectivity, and excellent recyclability compared to conventional Speier's catalyst. []
A: Studies using hexachloroplatinic acid (H2PtCl6) and [Pt(NH3)4]Cl2 as platinum precursors for n-heptane isomerization revealed that the choice of precursor significantly impacts the reaction selectivity. Using hexachloroplatinic acid led to increased selectivity for the desired di- and trimethyl-substituted heptane isomers, particularly when supported on Y and BEA zeolites. []
A: The molecular formula of hexachloroplatinic acid hexahydrate is H2PtCl6.6H2O. Its molecular weight is 588.09 g/mol. []
ANone: Various techniques are employed to characterize platinum complexes, including:
- XRDA (X-ray Diffraction Analysis): Determines the crystal structure and bond lengths/angles within the complex. []
- IR Spectroscopy: Identifies functional groups and bonding modes within the complex. []
- NMR Spectroscopy: Provides information about the structure, dynamics, and environment of atoms in the complex. [, ]
- XPS (X-ray Photoelectron Spectroscopy): Analyzes the elemental composition and oxidation states of platinum in the complex. [, , ]
- TEM (Transmission Electron Microscopy): Visualizes the size and morphology of platinum nanoparticles formed. [, , , ]
A: Yes, research demonstrates that by controlling reaction parameters such as the type and concentration of reducing agent, stabilizer, temperature, and pH, it is possible to synthesize platinum nanoparticles with a desired size range. For example, using hydrazine as a reducing agent and varying temperature and pH allows for tailoring the size of the resulting platinum particles. []
A: PVP acts as a stabilizing agent during the synthesis of platinum nanoparticles. It helps control the nucleation and growth rates of the particles, preventing their aggregation and influencing their final size distribution. [, , , ]
A: Yes, certain fungal strains, such as Fusarium oxysporum, can reduce hexachloroplatinic acid and synthesize platinum nanoparticles both intracellularly and extracellularly. This biosynthesis approach offers a potentially more environmentally friendly alternative to traditional chemical methods. []
ANone: Carbon xerogels possess several advantages as support materials for platinum catalysts:
- Controllable texture: Their structure can be tailored to optimize catalyst performance. []
- Reproducible synthesis: Allows for consistent material properties. []
- High purity: Minimizes the presence of impurities that could interfere with catalytic activity. []
A: The SEA method is a technique for preparing highly dispersed catalysts. It involves controlling the pH of a metal precursor solution (e.g., hexachloroplatinic acid) to promote electrostatic adsorption onto a support material (e.g., carbon xerogel). Subsequent drying and reduction steps lead to the formation of well-dispersed metal nanoparticles on the support. []
A: Calcination of the catalyst precursor before reduction strengthens the interaction between hexachloroplatinic acid and the support material. This leads to improved electrochemical stability of the final catalyst. []
A: Dechlorination aims to remove residual chlorine species from the catalyst after reduction. While it can increase platinum crystallite size, it doesn't significantly affect the catalyst's surface area or electrochemical performance. []
A: Yes, hexachloroplatinic acid can be used alongside other metal precursors to synthesize bimetallic catalysts. For example, combining it with ruthenium chloride in ethylene glycol solution, followed by reduction, yields platinum-ruthenium nanoparticles with tunable properties. These nanoparticles are explored for their potential application in fuel cells due to their enhanced tolerance towards carbon monoxide poisoning. [, ]
ANone: These catalysts are particularly promising as anode catalysts in:
- PEM fuel cells (Proton Exchange Membrane Fuel Cells): Enhance efficiency and reduce cost. [, ]
- DMFCs (Direct Methanol Fuel Cells): Improve performance and CO tolerance. []
A: The choice of reducing agent and reaction conditions significantly influence the properties of the resulting platinum nanoparticles. For instance, using stannous acid as a reducing agent leads to the formation of oxo composite Pt/C catalysts where both platinum metal and platinum oxides coexist within nanometer dimensions. This unique structure enhances the bifunctional catalytic activity of platinum in methanol electro-oxidation. []
ANone: Several strategies allow for porosity control, including:
- Adjusting the platinum catalyst content: Higher platinum content can lead to increased porosity in platinum-polycarbosilane composites. []
- Varying the post-treatment conditions: Temperature and atmosphere used during post-treatment significantly impact the final porosity. []
- Adding porogens during synthesis: Incorporating a porogen like divinylbenzene can enhance porosity in the final material. []
A: Entrapping platinum nanoparticles within TNT creates a confined environment that enhances their catalytic activity for phenol hydrogenation. This confinement effect is attributed to the unique interaction between the platinum nanoparticles and the TNT support, leading to higher catalytic efficiency. []
A: Techniques like temperature-programmed bulk reduction (t.p.b.r.) and temperature-programmed desorption (t.p.d.) provide valuable insights into the reduction behavior and adsorption/desorption characteristics of platinum catalysts. These methods can reveal information about different platinum species present, their interaction with the support, and the influence of factors like platinum dispersion. []
A: The support material significantly affects the reduction temperature and profile. For instance, silica-supported platinum samples generally show lower reduction temperatures compared to unsupported platinum. The choice of support material also influences the number and intensity of observed reduction peaks, reflecting the varying interaction between platinum and the support. []
A: Diffusion plays a crucial role in temperature-programmed analysis, and its rate can significantly affect the interpretation of obtained data. If diffusion is too slow during temperature-programmed reduction, it may mask the presence of different platinum species or lead to inaccurate estimations of reduction temperatures. Conversely, rapid diffusion during temperature-programmed desorption can hinder accurate quantification of desorbed species. []
A: Nitrogen doping enhances the interaction between platinum and the carbon support, leading to improved catalyst performance and stability. The presence of nitrogen disrupts the sp2 bonding network in graphitic carbon, creating stronger anchoring sites for platinum nanoparticles and mitigating their detachment or aggregation. []
A: The degree of structural order within the carbon support influences the dispersion, activity, and stability of the supported platinum nanoparticles. A higher level of disorder often translates to a greater number of defects and edge sites, which can act as anchoring points for platinum, leading to improved catalyst performance. []
A: Cyclic voltammetry is a common technique for determining the ESA of platinum nanowires. By analyzing the electrochemical response of the material within a specific potential window, the charge associated with surface redox processes can be calculated and correlated to the active surface area of the catalyst. []
ANone: Platinum nanowires offer several potential benefits over conventional Pt/C catalysts:
- Higher durability: Nanowires demonstrate better resistance to degradation during accelerated degradation tests, potentially increasing the lifespan of fuel cell components. []
- Enhanced performance: Employing platinum nanowires mixed with Pt/C in the fuel cell cathode can lead to improved overall cell performance. []
ANone: Centrifugal electrospinning offers several advantages for producing platinum nanowires:
- High production rate: Significantly faster production compared to traditional electrospinning methods. []
- Controllable morphology: Allows for tailoring nanowire diameter by adjusting system parameters. []
A: These sensors show promise for reliable multipoint-leakage monitoring, particularly for hydrogen gas detection. The catalytic combustion of hydrogen on the platinum surface generates heat, leading to a measurable shift in the FBG's center wavelength, providing a sensitive and robust method for hydrogen detection. []
A: An optimized silica-to-platinum ratio is crucial for sensor performance. Research shows that a Si:Pt atom ratio of 13:1 in the precursor solution leads to a significant temperature change (75 K) upon exposure to 3 vol% H2, highlighting the importance of optimizing catalyst composition for high sensitivity. []
A: Hexachloroplatinic acid acts as a catalyst for the hydrosilylation reaction, promoting crosslinking of allyl groups present in the polycarbosilane precursor. This crosslinking reaction is essential for forming the porous structure of the final CeO2/silicon oxycarbide catalyst. []
A: Yes, research demonstrates the successful fabrication of platinum nanowires using a centrifugal electrospinning method with hexachloroplatinic acid as the platinum precursor. By controlling the system parameters, such as polyvinylpyrrolidone (PVP) concentration, rotation speed, and applied electric field, researchers can tailor the morphology and electrochemical properties of the resulting nanowires. The produced nanowires exhibit comparable characteristics to those made through traditional electrospinning, with the added benefit of a greatly improved production rate. []
A: Research suggests that the use of organic stabilizing agents like polypyrrole can significantly influence the stability of platinum nanoparticles under acidic conditions, which is a crucial factor for PEMFC applications. By studying the stability of PVP-capped 3 nm platinum nanoparticles in acidic environments using electrochemistry and temperature-programmed oxidation techniques, researchers can gain insights into the role of stabilizing agents and their impact on nanoparticle durability. []
A: When carbon-supported ruthenium nanoparticles are immersed in hexachloroplatinic acid solutions, a displacement reaction occurs. This reaction leads to the formation of a ruthenium core surrounded by a platinum shell (Ru@Pt bimetallic structure). The extent of this reaction is influenced by the pH of the hexachloroplatinic acid solution, with higher pH values generally leading to a more pronounced displacement reaction and a thicker platinum shell. []
A: DFT calculations using the hybrid functional B3LYP have been employed to elucidate the mechanism of platina-β-diketone formation. These calculations reveal that the reaction, which involves the transformation of a propyne complex [PtCl3(η2-MeC⋮CH)]- (10) to a mononuclear platina-β-diketonate anion [PtCl2{(COEt)2H}]- (11), is highly exothermic. By exploring different reaction pathways, DFT calculations provide a detailed understanding of the steps involved, including alkyne-vinylidene equilibration, ligand substitution, water addition, and deprotonation, leading to the final product. []
A: Research highlights the crucial role of carbon support surface chemistry in determining the properties of platinum catalysts. Studies utilizing a range of activated carbon supports with varying surface oxygen content, prepared through oxidative treatments, demonstrate a clear link between the support's point of zero charge (PZC) and the platinum loading. Higher PZC values, often associated with increased surface basicity, correlate with higher platinum loading. Moreover, the platinum dispersion is influenced by the total surface oxygen content, with higher oxygen content leading to improved platinum dispersion. This suggests that surface oxygen groups act as anchoring sites for platinum, influencing both the loading and dispersion of the metal on the support. []
A: Hexachloroplatinic acid serves as a catalyst in the hydrosilylation reaction between poly(hydrogenmethylsiloxane-co-dimethylsiloxane)s (DH-D copolymers) and 1-allyloxy-2,3-epoxypropane (allylglycidylether). This reaction allows for the incorporation of epoxy groups as lateral substituents onto the polysiloxane backbone, leading to the formation of functionalized polysiloxanes with tailored properties. []
A: Kinetic studies reveal a distinctive neighboring effect during the hydrosilylation of DH-D copolymers. The reaction exhibits first-order kinetics with respect to platinum and double bonds but a fractional order (0.5) with respect to SiH. This suggests that hydrogenmethylsiloxane dyads are significantly more reactive than isolated units, indicating a cooperative effect between adjacent SiH groups during the catalytic cycle. This neighboring effect has implications for the microstructure of the final functionalized polysiloxane. []
A: Studies show that adding hexachloroplatinic acid to ternary systems containing nonionic surfactant and water significantly influences the temperature and composition ranges of liquid crystalline phases. Notably, the presence of hexachloroplatinic acid enhances the stability of highly curved phases, such as the I1 micellar cubic and HI phases. This alteration in phase behavior has implications for the synthesis of nanostructured platinum materials using these lyotropic liquid crystalline systems as templates. []
A: Yes, colloidal platinum nanoparticles prepared from hexachloroplatinic acid significantly enhance the chemiluminescence (CL) of the luminol-H2O2 system. This enhancement is attributed to the catalytic activity of the platinum nanoparticles, which accelerate electron transfer processes and facilitate the generation of chemiluminescent radicals. This finding has potential applications in developing sensitive analytical methods for detecting various compounds. []
A: Yes, research demonstrates the feasibility of using hexachloroplatinic acid to synthesize water-soluble platinum nanoparticles capped with biocompatible molecules like folic acid and poly(vinyl pyrrolidone) (PVP). These nanoparticles exhibit promising results for targeted drug delivery, particularly towards cancer cells. For instance, folic acid-capped platinum nanoparticles show enhanced cytotoxicity towards MCF7 breast cancer cells compared to PVP-capped nanoparticles, highlighting the potential for active targeting using appropriately functionalized nanoparticles. []
A: Hexachloroplatinic acid plays a key role in functionalizing nanosilicon dioxide with terminated polyether chains, leading to improved oil displacement properties. This involves reacting hexachloroplatinic acid with terminated allyl alcohol polyoxy alkyl ether and triethoxy chloride silane to create a silane coupling agent. This coupling agent is then reacted with the nanosilicon dioxide, resulting in a modified material with enhanced performance in oil displacement applications. []
Haftungsausschluss und Informationen zu In-Vitro-Forschungsprodukten
Bitte beachten Sie, dass alle Artikel und Produktinformationen, die auf BenchChem präsentiert werden, ausschließlich zu Informationszwecken bestimmt sind. Die auf BenchChem zum Kauf angebotenen Produkte sind speziell für In-vitro-Studien konzipiert, die außerhalb lebender Organismen durchgeführt werden. In-vitro-Studien, abgeleitet von dem lateinischen Begriff "in Glas", beinhalten Experimente, die in kontrollierten Laborumgebungen unter Verwendung von Zellen oder Geweben durchgeführt werden. Es ist wichtig zu beachten, dass diese Produkte nicht als Arzneimittel oder Medikamente eingestuft sind und keine Zulassung der FDA für die Vorbeugung, Behandlung oder Heilung von medizinischen Zuständen, Beschwerden oder Krankheiten erhalten haben. Wir müssen betonen, dass jede Form der körperlichen Einführung dieser Produkte in Menschen oder Tiere gesetzlich strikt untersagt ist. Es ist unerlässlich, sich an diese Richtlinien zu halten, um die Einhaltung rechtlicher und ethischer Standards in Forschung und Experiment zu gewährleisten.