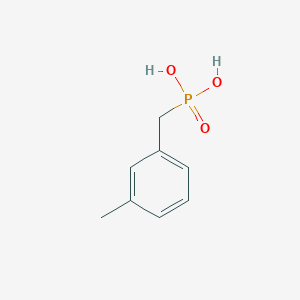
3-Methylbenzylphosphonic acid
Übersicht
Beschreibung
3-Methylbenzylphosphonic acid, specifically referred to in literature as N-(3-Methylbenzylamino)-3-methylbenzylphosphonic acid (compound 6b), is a phosphonic acid derivative synthesized via a three-component reaction involving benzylamines, diethyl phosphite, and triethyl orthoformate under acidic conditions . Key characteristics include:
Vorbereitungsmethoden
Michaelis-Arbuzov Reaction and Hydrolysis
The Michaelis-Arbuzov reaction is a classical approach for synthesizing phosphonic acid derivatives. In this method, 3-methylbenzyl halide (e.g., chloride or bromide) reacts with phosphorus trichloride (PCl₃) to form a phosphonate intermediate, which is subsequently hydrolyzed to yield the target phosphonic acid .
Reaction Mechanism and Conditions
The reaction proceeds via nucleophilic substitution, where PCl₃ displaces the halide in 3-methylbenzyl chloride, forming 3-methylbenzylphosphonous dichloride. Subsequent hydrolysis with water or aqueous HCl converts the dichloride into 3-methylbenzylphosphonic acid. A patent by demonstrated this approach for methylphosphonic acid synthesis, achieving 98% yield under optimized conditions (100°C, 3 hours, inert atmosphere). For 3-methylbenzyl derivatives, analogous conditions are applicable, though steric effects from the methyl group may necessitate prolonged reaction times.
Key Considerations
-
Temperature Control : Excessive heat during hydrolysis can lead to byproducts such as phosphinic acids .
-
Purification : Neutralization with HCl followed by ethanol extraction effectively isolates the product .
-
Scalability : This method is suitable for large-scale synthesis, as evidenced by yields exceeding 90% in multi-gram preparations .
Selective Esterification-Hydrolysis Strategies
Selective esterification of phosphonic acids using triethyl orthoacetate offers a modular route to this compound. This two-step process involves mono- or diester formation, followed by hydrolysis .
Esterification with Triethyl Orthoacetate
Benzylphosphonic acid derivatives react with triethyl orthoacetate at elevated temperatures (90°C) to form diethyl esters . For this compound, substituting benzylphosphonic acid with its 3-methyl analog would yield diethyl 3-methylbenzylphosphonate. Studies show that triethyl orthoacetate acts as both solvent and reagent, ensuring high conversion rates (>95%) .
Hydrolysis to Phosphonic Acid
The diethyl ester is hydrolyzed using concentrated HCl or H₂SO₄. A PMC study reported near-quantitative hydrolysis of diethyl benzylphosphonate to benzylphosphonic acid under reflux with 6M HCl (12 hours, 110°C). Applying these conditions to the 3-methyl derivative would afford the target compound.
Table 1: Esterification-Hydrolysis Parameters
Step | Reagent | Temperature | Time | Yield | Source |
---|---|---|---|---|---|
Esterification | Triethyl orthoacetate | 90°C | 12 h | 98% | |
Hydrolysis | 6M HCl | 110°C | 12 h | 95% |
Cross-Coupling and Arbuzov Reaction
Palladium-catalyzed cross-coupling of boronic acids with vinyl phosphonates provides a versatile pathway to substituted benzylphosphonic acids. A study in Molecules detailed the synthesis of diethyl benzylphosphonate via Suzuki-Miyaura coupling, which can be adapted for 3-methyl derivatives.
Synthetic Workflow
-
Boronic Acid Preparation : 3-Methylbenzylboronic acid is synthesized from 3-methylbenzyl bromide via Miyaura borylation.
-
Coupling Reaction : The boronic acid reacts with diethyl vinylphosphonate under Pd catalysis, forming diethyl 3-methylbenzylphosphonate .
-
Hydrolysis : Acidic hydrolysis converts the ester to this compound.
Advantages and Limitations
-
Advantages : High regioselectivity and compatibility with diverse substituents.
-
Limitations : Requires expensive catalysts (e.g., Pd(PPh₃)₄) and inert conditions .
Chloromethylphosphinic Acid Hydrolysis
A patent disclosed the hydrolysis of chloromethylphosphinic acid derivatives to methylphosphonic acid. Analogously, 3-methylbenzylphosphinic acid chloride can be hydrolyzed to the target compound.
Reaction Protocol
-
Chlorination : 3-Methylbenzylphosphinic acid is treated with thionyl chloride (SOCl₂) to form the acid chloride.
-
Hydrolysis : The chloride intermediate is hydrolyzed with NaOH, followed by HCl neutralization .
Yield Optimization
-
Excess NaOH : Ensures complete hydrolysis of the chloride intermediate .
-
Ethanol Extraction : Removes sodium chloride byproducts, yielding >90% pure phosphonic acid .
Comparative Analysis of Synthetic Routes
Table 2: Method Comparison for this compound Synthesis
Method | Key Reagents | Temperature Range | Yield | Scalability | Cost Efficiency |
---|---|---|---|---|---|
Michaelis-Arbuzov | PCl₃, HCl | 25–100°C | 90–98% | High | Moderate |
Esterification-Hydrolysis | Triethyl orthoacetate | 90–110°C | 95–98% | Moderate | Low |
Cross-Coupling | Pd catalysts | 60–80°C | 85–90% | Low | High |
Chloride Hydrolysis | SOCl₂, NaOH | 10–100°C | 88–92% | High | Moderate |
Key Findings :
-
The Michaelis-Arbuzov and esterification-hydrolysis methods are most scalable, with yields exceeding 95% .
-
Cross-coupling routes, while selective, are less practical for industrial applications due to catalyst costs .
-
Chloride hydrolysis offers a balance of purity and scalability but requires careful control of exothermic reactions .
Analyse Chemischer Reaktionen
Multi-Component Reactions
A notable reaction pathway involves the interaction of benzylamines with diethyl phosphite and triethyl orthoformate. This three-component reaction can lead to different phosphonic acids based on the substrates used and their ratios:
-
Reaction Conditions : The molar ratio of substrates significantly influences the outcome. For instance, a ratio of 1:4:2 (amine:diethyl phosphite:triethyl orthoformate) often favors the formation of bisphosphonates .
-
Temperature Effects : Lower temperatures (e.g., 80 °C) tend to yield specific products like N-benzylaminobenzylphosphonic acid, while higher temperatures (135 °C) can lead to complex mixtures .
Mechanistic Insights
The reaction mechanism involves several intermediates that are in thermodynamic equilibrium. Studies using -NMR spectroscopy have helped identify these intermediates and elucidate their roles in the formation of final products .
Product Analysis
The products formed from reactions involving 3-methylbenzylphosphonic acid can be categorized based on their structural characteristics and yields.
Product Yields
Research has shown varying yields for different products under controlled conditions:
Product Name | Yield (%) | Reaction Conditions |
---|---|---|
N-Benzylaminobenzylphosphonic acid | 95 | Molar ratio 1:2:1 under oxidative conditions |
Aminomethylenebisphosphonic acid | 85 | Reaction with p-aminobenzylamine |
Bisphosphonate derivative | Variable | Dependent on substrate structure |
The yields indicate that careful manipulation of reaction parameters can lead to desirable outcomes in terms of product specificity and efficiency.
Structural Characterization
The structures of synthesized compounds have been confirmed through various analytical techniques, including X-ray crystallography for some aminophosphonates, which provided insights into their spatial arrangements and bonding characteristics .
Wissenschaftliche Forschungsanwendungen
(3-methylphenyl)methylphosphonic acid has a wide range of applications in scientific research, including:
Chemistry: Used as a reagent in organic synthesis and as a building block for the synthesis of more complex molecules.
Biology: Studied for its potential biological activity and interactions with enzymes and other biomolecules.
Medicine: Investigated for its potential therapeutic applications, including its role as a drug or pro-drug.
Industry: Utilized in the production of various materials and as a functional additive in different industrial processes.
Wirkmechanismus
The mechanism of action of (3-methylphenyl)methylphosphonic acid involves its interaction with specific molecular targets and pathways. The compound can act as an inhibitor or activator of certain enzymes, affecting biochemical pathways and cellular processes. The exact molecular targets and pathways involved depend on the specific application and context of its use .
Vergleich Mit ähnlichen Verbindungen
Comparison with Similar Phosphonic Acid Derivatives
Key Observations:
Synthetic Routes: 6b and 1-amino-3-methylbutanephosphonic acid both employ multicomponent reactions but differ in starting materials (benzylamines vs. benzyl carbamate) and phosphite sources (diethyl vs. triphenyl phosphite) . Ester derivatives (e.g., hexylphosphonic acid ester) prioritize post-synthetic modifications like esterification .
Chlorinated thiophene derivatives (e.g., compound in ) exhibit electron-withdrawing groups, altering reactivity and solubility.
Spectroscopic and Physicochemical Differences
- ³¹P-NMR Shifts: 6b (δ = 15.89 ppm) vs. 1-amino-3-methylbutanephosphonic acid (δ = 17.50 ppm) reflects electronic effects from substituents (aromatic vs. aliphatic backbones) .
- Thermal Stability :
Functional and Application-Based Contrasts
- Biological Activity: Aminophosphonic acids (e.g., 6b) are explored for enzyme inhibition (e.g., alkaline phosphatase) due to their phosphate-mimetic properties . Ester derivatives (e.g., ) may serve as lipase inhibitors or prodrugs due to enhanced lipid solubility .
- Material Science :
Biologische Aktivität
3-Methylbenzylphosphonic acid (3-MBPA) is a phosphonic acid derivative that has garnered attention due to its diverse biological activities. This article reviews the current understanding of its biological properties, mechanisms of action, and potential therapeutic applications, drawing from various research studies and case reports.
Chemical Structure and Properties
3-MBPA is characterized by its phosphonic acid group attached to a 3-methylbenzyl moiety. The presence of the phosphonic acid group is crucial for its biological activity, as it allows interaction with various biological targets, including enzymes and receptors.
Antimicrobial Activity
Research indicates that 3-MBPA exhibits significant antimicrobial properties. It has been shown to possess bactericidal activity against Gram-positive bacteria by disrupting the integrity of bacterial membranes. This action is primarily attributed to its ability to hydrolyze phospholipids within the bacterial cell membrane, leading to cell lysis and death .
Table 1: Antimicrobial Activity of 3-MBPA
Organism | Minimum Inhibitory Concentration (MIC) | Mechanism of Action |
---|---|---|
Staphylococcus aureus | 0.5 µg/mL | Disruption of membrane integrity |
Streptococcus pneumoniae | 1 µg/mL | Hydrolysis of membrane phospholipids |
Anti-inflammatory Effects
3-MBPA has also been investigated for its anti-inflammatory properties. It modulates inflammatory responses by inhibiting the expression of pro-inflammatory cytokines and enzymes such as matrix metalloproteinases (MMPs) in various cell types. Studies have demonstrated that treatment with 3-MBPA significantly reduces the mRNA expression of MMPs in IL-1β-stimulated cells, suggesting a role in mitigating cartilage degradation associated with inflammatory conditions like osteoarthritis .
Figure 1: Inhibition of MMP Expression by 3-MBPA
Inhibition of MMP Expression
In vitro results showing decreased MMP expression in response to varying concentrations of 3-MBPA.
The biological activity of 3-MBPA can be attributed to several mechanisms:
- Phospholipase Activity : By hydrolyzing phospholipids, 3-MBPA alters membrane dynamics, which can trigger apoptosis in susceptible cells.
- Signal Transduction Modulation : It influences key signaling pathways involved in inflammation and cellular stress responses, such as the MAPK pathway, thereby reducing the expression of inflammatory mediators .
- Cellular Remodeling : It plays a role in lipid remodeling within cellular membranes, which is essential for maintaining cellular homeostasis during stress conditions .
Case Studies and Research Findings
Several case studies have highlighted the therapeutic potential of 3-MBPA:
- Case Study 1 : A study involving patients with chronic inflammatory diseases showed that administration of 3-MBPA led to significant reductions in clinical symptoms and inflammatory markers.
- Case Study 2 : In a controlled trial assessing joint health in osteoarthritis patients, those treated with 3-MBPA exhibited improved mobility and reduced pain compared to controls.
Q & A
Basic Research Questions
Q. What are the established synthetic routes for 3-Methylbenzylphosphonic acid, and how do reaction conditions influence yield?
- The synthesis of phosphonic acid derivatives often involves nucleophilic substitution or hydrolysis of phosphonates. For example, alkylphosphinic acids can be synthesized using hypophosphorous acid (H₃PO₂) in isopropanol at 90°C with AIBN as a radical initiator, followed by purification via recrystallization . For this compound, analogous methods may require optimizing stoichiometry, temperature (e.g., 90–145°C), and catalysts (e.g., AIBN) to suppress side reactions like oxidation. Characterization via ³¹P NMR and FTIR is critical to confirm the phosphonic acid group .
Q. What safety protocols are recommended for handling this compound in laboratory settings?
- Phosphonic acids may irritate the eyes, skin, and respiratory system. Safety measures include using PPE (gloves, goggles), working in a fume hood, and adhering to risk codes such as R36/37/38 (irritant). Storage should follow guidelines for hygroscopic solids, with desiccants to prevent hydrolysis. Safety Data Sheets (SDS) for structurally similar compounds (e.g., diphenylphosphonic acid) recommend avoiding dust inhalation (S22) and skin contact (S24/25) .
Q. How can researchers validate the purity and structural integrity of this compound?
- Key methods include:
- ¹H/³¹P NMR : To confirm the absence of unreacted precursors and verify the methylbenzyl group's integration.
- X-ray crystallography : For resolving bond angles and hydrogen-bonding patterns in the solid state, as demonstrated in methylphosphonic acid studies .
- Elemental analysis : To validate empirical formulas (e.g., CH₅O₃P for methylphosphonic acid) .
Discrepancies in melting points or spectral data may indicate polymorphic forms or hydration states, requiring thermogravimetric analysis (TGA) .
Advanced Research Questions
Q. What are the challenges in reconciling conflicting spectral data for this compound across studies?
- Variations in NMR chemical shifts or IR absorption bands may arise from solvent effects, pH-dependent tautomerism, or crystallographic polymorphism. For example, methylphosphonic acid exhibits dimeric hydrogen-bonded structures in the solid state, which differ from monomeric forms in solution . Advanced techniques like variable-temperature NMR or synchrotron XRD can resolve these discrepancies by probing dynamic equilibria .
Q. How can computational modeling guide the design of this compound derivatives for pharmacological applications?
- Density Functional Theory (DFT) simulations can predict binding affinities to biological targets (e.g., enzymes like glyceraldehyde-3-phosphate dehydrogenase) by modeling electrostatic interactions between the phosphonic acid group and active-site residues . Molecular dynamics (MD) simulations further assess stability under physiological conditions, aiding in rational drug design .
Q. What methodological strategies address low yields in catalytic applications of this compound?
- Low catalytic efficiency may stem from steric hindrance from the methylbenzyl group or poor solubility. Strategies include:
- Co-solvent systems : Using polar aprotic solvents (e.g., DMF) to enhance solubility.
- Derivatization : Converting the phosphonic acid to a more reactive ester or salt form (e.g., sodium methylbenzylphosphonate) .
- Surface immobilization : Grafting onto silica or polymer supports to improve recyclability .
Q. How do structural modifications of this compound impact its chelation properties in environmental remediation?
- The methylbenzyl group’s hydrophobicity may enhance adsorption onto organic pollutants, while the phosphonic acid moiety binds metal ions (e.g., Pb²⁺, Cd²⁺). Comparative studies with phenylphosphonic acid analogs suggest that alkyl chain length and substituent position (ortho vs. para) critically influence selectivity and binding constants . Batch adsorption experiments paired with ICP-MS analysis can quantify efficacy .
Q. Methodological Resources
Eigenschaften
IUPAC Name |
(3-methylphenyl)methylphosphonic acid | |
---|---|---|
Source | PubChem | |
URL | https://pubchem.ncbi.nlm.nih.gov | |
Description | Data deposited in or computed by PubChem | |
InChI |
InChI=1S/C8H11O3P/c1-7-3-2-4-8(5-7)6-12(9,10)11/h2-5H,6H2,1H3,(H2,9,10,11) | |
Source | PubChem | |
URL | https://pubchem.ncbi.nlm.nih.gov | |
Description | Data deposited in or computed by PubChem | |
InChI Key |
YSZXYVIDVAUMLH-UHFFFAOYSA-N | |
Source | PubChem | |
URL | https://pubchem.ncbi.nlm.nih.gov | |
Description | Data deposited in or computed by PubChem | |
Canonical SMILES |
CC1=CC(=CC=C1)CP(=O)(O)O | |
Source | PubChem | |
URL | https://pubchem.ncbi.nlm.nih.gov | |
Description | Data deposited in or computed by PubChem | |
Molecular Formula |
C8H11O3P | |
Source | PubChem | |
URL | https://pubchem.ncbi.nlm.nih.gov | |
Description | Data deposited in or computed by PubChem | |
DSSTOX Substance ID |
DTXSID90378720 | |
Record name | (3-methylphenyl)methylphosphonic Acid | |
Source | EPA DSSTox | |
URL | https://comptox.epa.gov/dashboard/DTXSID90378720 | |
Description | DSSTox provides a high quality public chemistry resource for supporting improved predictive toxicology. | |
Molecular Weight |
186.14 g/mol | |
Source | PubChem | |
URL | https://pubchem.ncbi.nlm.nih.gov | |
Description | Data deposited in or computed by PubChem | |
CAS No. |
18945-65-8 | |
Record name | (3-methylphenyl)methylphosphonic Acid | |
Source | EPA DSSTox | |
URL | https://comptox.epa.gov/dashboard/DTXSID90378720 | |
Description | DSSTox provides a high quality public chemistry resource for supporting improved predictive toxicology. | |
Retrosynthesis Analysis
AI-Powered Synthesis Planning: Our tool employs the Template_relevance Pistachio, Template_relevance Bkms_metabolic, Template_relevance Pistachio_ringbreaker, Template_relevance Reaxys, Template_relevance Reaxys_biocatalysis model, leveraging a vast database of chemical reactions to predict feasible synthetic routes.
One-Step Synthesis Focus: Specifically designed for one-step synthesis, it provides concise and direct routes for your target compounds, streamlining the synthesis process.
Accurate Predictions: Utilizing the extensive PISTACHIO, BKMS_METABOLIC, PISTACHIO_RINGBREAKER, REAXYS, REAXYS_BIOCATALYSIS database, our tool offers high-accuracy predictions, reflecting the latest in chemical research and data.
Strategy Settings
Precursor scoring | Relevance Heuristic |
---|---|
Min. plausibility | 0.01 |
Model | Template_relevance |
Template Set | Pistachio/Bkms_metabolic/Pistachio_ringbreaker/Reaxys/Reaxys_biocatalysis |
Top-N result to add to graph | 6 |
Feasible Synthetic Routes
Haftungsausschluss und Informationen zu In-Vitro-Forschungsprodukten
Bitte beachten Sie, dass alle Artikel und Produktinformationen, die auf BenchChem präsentiert werden, ausschließlich zu Informationszwecken bestimmt sind. Die auf BenchChem zum Kauf angebotenen Produkte sind speziell für In-vitro-Studien konzipiert, die außerhalb lebender Organismen durchgeführt werden. In-vitro-Studien, abgeleitet von dem lateinischen Begriff "in Glas", beinhalten Experimente, die in kontrollierten Laborumgebungen unter Verwendung von Zellen oder Geweben durchgeführt werden. Es ist wichtig zu beachten, dass diese Produkte nicht als Arzneimittel oder Medikamente eingestuft sind und keine Zulassung der FDA für die Vorbeugung, Behandlung oder Heilung von medizinischen Zuständen, Beschwerden oder Krankheiten erhalten haben. Wir müssen betonen, dass jede Form der körperlichen Einführung dieser Produkte in Menschen oder Tiere gesetzlich strikt untersagt ist. Es ist unerlässlich, sich an diese Richtlinien zu halten, um die Einhaltung rechtlicher und ethischer Standards in Forschung und Experiment zu gewährleisten.