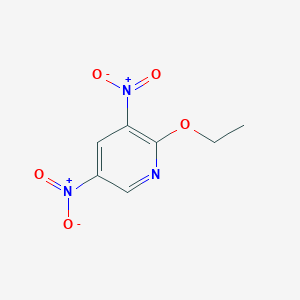
2-Ethoxy-3,5-dinitropyridine
Übersicht
Beschreibung
2-Ethoxy-3,5-dinitropyridine is a nitro-substituted pyridine derivative characterized by an ethoxy group at the 2-position and two nitro groups at the 3- and 5-positions. This compound exhibits significant electrophilicity due to the electron-withdrawing nitro groups, making it reactive in nucleophilic aromatic substitution (SNAr) reactions. Its structure is optimized for participation in σ-complex (Meisenheimer complex) formation during SNAr, a process extensively studied via density functional theory (DFT) and kinetic analyses . Applications span synthetic chemistry, particularly in synthesizing heterocyclic compounds and intermediates for pharmaceuticals or propellants .
Vorbereitungsmethoden
Synthetic Routes and Reaction Conditions: The synthesis of 2-ethoxy-3,5-dinitropyridine typically involves the nitration of 2-ethoxypyridine. The nitration process can be carried out using a mixture of concentrated nitric acid and sulfuric acid. The reaction is usually conducted at low temperatures to control the rate of nitration and to avoid over-nitration. The product is then purified through recrystallization or column chromatography.
Industrial Production Methods: In an industrial setting, the production of this compound may involve continuous flow nitration processes to enhance efficiency and yield. The use of automated systems allows for precise control of reaction conditions, ensuring consistent product quality.
Analyse Chemischer Reaktionen
Nucleophilic Aromatic Substitution (SNAr) Reactions
EDNP undergoes SNAr reactions primarily at the 6-position of the pyridine ring. The ethoxy group at position 2 acts as a leaving group, while the nitro groups stabilize the Meisenheimer σ-adduct intermediate through resonance and inductive effects .
Mechanistic Pathway
-
Formation of the Meisenheimer Complex :
Nucleophilic attack (e.g., by piperidine or aliphatic amines) at the 6-position generates a zwitterionic σ-adduct intermediate stabilized by hydrogen bonding . -
Base-Catalyzed Decomposition :
The σ-adduct undergoes deprotonation, followed by elimination of the ethoxy group to yield the substitution product .
Key Computational Findings (B3PW91/6-31G(d,p)) :
Step | Activation Energy (kcal/mol) | Thermodynamic ΔH (kcal/mol) |
---|---|---|
Meisenheimer Formation | 14.8 (base-catalyzed) | -6.5 (exothermic) |
Ethoxy Elimination | 28.0 (uncatalyzed) | -0.6 (exothermic) |
Reactivity with Aliphatic Amines
In DMSO, EDNP reacts reversibly with aliphatic amines (e.g., piperidine) to form σ-adducts. The reaction is base-catalyzed, with proton transfer identified as the rate-limiting step .
Experimental Rate Constants (DMSO, 25°C) :
Amine | k<sub>formation</sub> (M<sup>−1</sup>s<sup>−1</sup>) | K<sub>eq</sub> (σ-adduct stability) |
---|---|---|
Piperidine | 1.2 × 10<sup>3</sup> | 0.45 |
n-Butylamine | 8.7 × 10<sup>2</sup> | 0.32 |
Data from Crampton et al. (2003) .
Comparative Reactivity
EDNP is less reactive than 2-phenoxy-3,5-dinitropyridine due to the weaker electron-donating effect of the ethoxy group compared to phenoxy .
Solvent and Substituent Effects
-
Solvent Polarity : Reactions in polar aprotic solvents (e.g., DMSO) favor σ-adduct stabilization, while protic solvents accelerate decomposition .
-
Steric Effects : The planar structure of EDNP minimizes steric hindrance, enabling faster σ-adduct formation compared to ortho-substituted nitrobenzenes .
Reaction with Aromatic Amines
EDNP reacts with p-substituted anilines in DMSO via a two-step mechanism:
-
Rapid σ-adduct formation.
-
Rate-determining ethoxy elimination.
Substituent Effects on Reactivity :
Aniline Substituent | k<sub>obs</sub> (M<sup>−1</sup>s<sup>−1</sup>) |
---|---|
-NO<sub>2</sub> | 2.5 × 10<sup>−2</sup> |
-OCH<sub>3</sub> | 8.9 × 10<sup>−4</sup> |
Electron-withdrawing groups enhance reactivity .
Base Catalysis
Base catalysis lowers the activation energy for σ-adduct decomposition by facilitating deprotonation. For example:
Wissenschaftliche Forschungsanwendungen
Chemical Properties and Structure
2-Ethoxy-3,5-dinitropyridine is characterized by the presence of two nitro groups at the 3 and 5 positions of the pyridine ring, along with an ethoxy group at the 2 position. The molecular structure contributes to its reactivity in nucleophilic aromatic substitution (SNAr) reactions, which are crucial for synthesizing more complex organic molecules.
Nucleophilic Aromatic Substitution (SNAr) Reactions
The SNAr reaction of this compound has been extensively studied due to its implications in organic synthesis and drug development. Research indicates that this compound can undergo nucleophilic attack by various nucleophiles, such as piperidine, leading to the formation of Meisenheimer complexes. These intermediates are stabilized by hydrogen bonding, significantly influencing the reaction kinetics.
- Computational Studies : A computational analysis using density functional theory (DFT) has shown that the SNAr reaction proceeds through a two-step mechanism involving the formation and decomposition of the Meisenheimer complex. The activation energy for this process was calculated to be approximately 28 kcal/mol for the uncatalyzed pathway but reduced to about 14.8 kcal/mol when a base catalyst is used .
Drug Discovery and SARS-CoV-2 Research
Recent studies have highlighted the potential of this compound in drug discovery, particularly in developing antiviral agents against SARS-CoV-2. The compound's ability to engage in SNAr reactions makes it a candidate for synthesizing new therapeutic agents.
- Mechanistic Insights : Research focused on the reaction mechanisms of this compound with piperidine has provided insights into how modifications can enhance biological activity against viral targets .
Material Science Applications
The unique chemical properties of this compound also lend themselves to applications in material science. Its derivatives can be utilized in creating functional materials that exhibit specific electronic or optical properties.
A detailed study examined the reaction between this compound and piperidine using computational methods. The findings revealed:
Wirkmechanismus
The mechanism of action of 2-ethoxy-3,5-dinitropyridine primarily involves nucleophilic aromatic substitution reactions. The presence of electron-withdrawing nitro groups at the 3 and 5 positions makes the pyridine ring highly susceptible to nucleophilic attack. The reaction proceeds through the formation of a Meisenheimer complex intermediate, which is stabilized by hydrogen bonding . The base-catalyzed pathway involves rate-limiting proton transfer from the zwitterionic intermediate to the base, yielding the anionic sigma-adduct .
Vergleich Mit ähnlichen Verbindungen
Substituent Effects on Reactivity and Electronic Properties
The reactivity of 3,5-dinitropyridine derivatives is heavily influenced by the substituent at the 2-position. Key comparisons include:
Key Observations :
- Alkoxy vs. Chloro : The ethoxy group is a poorer leaving group than chloro, but the strong electron-withdrawing nitro groups activate the pyridine ring, enabling SNAr even with ethoxy. Chloro derivatives exhibit faster SNAr due to Cl⁻’s superior leaving ability .
- Ethoxy vs. Methoxy : Despite similar electron-donating effects, the ethoxy derivative’s reaction with piperidine is more exothermic (-6.5 vs. -0.6 kcal/mol), suggesting better stabilization of intermediates. However, methoxy compounds show lower activation barriers in base-catalyzed pathways .
- Hydroxy Derivatives : The acidic hydroxyl group (pKa ~4.41) allows deprotonation, forming salts critical in industrial applications (e.g., barium salts in propellants) .
Reaction Mechanisms and Kinetics
SNAr Pathways and Catalysis
- Ethoxy vs. Methoxy : Both undergo SNAr via Meisenheimer complex formation. For this compound, the rate-determining step shifts to Meisenheimer decomposition (uncatalyzed: ΔG‡ ~28 kcal/mol; base-catalyzed: ΔG‡ ~14.8 kcal/mol). Methoxy derivatives exhibit faster kinetics under basic conditions due to lower steric hindrance .
- Mechanistic Divergence : Reactions of 2-methoxy-3,5-dinitropyridine with cyclic amines follow a single electron transfer (SET) mechanism (βnuc = 0.94), evidenced by linear Brønsted plots. Ethoxy derivatives likely follow similar pathways, but the larger ethoxy group may introduce steric effects .
Kinetic Data in Acetonitrile (20°C):
Compound | Second-Order Rate Constant (k, L/mol·s) | Nucleophile | Reference |
---|---|---|---|
2-Methoxy-3,5-dinitropyridine | 0.15–2.7 × 10⁻³ | Cyclic amines | |
This compound | Not explicitly reported | Piperidine |
Physical and Chemical Properties
- Molecular Weight : this compound (C₇H₆N₃O₅) has a higher molecular weight (~236 g/mol) compared to 2-chloro-3,5-dinitropyridine (203.54 g/mol) .
- Acidity : The 2-hydroxy derivative’s acidity (pKa ~4.41) contrasts with the strongly electron-withdrawing 2,6-dimethoxy analog (pKa -6.50) .
- Thermal Stability : 2,6-Dimethoxy-3,5-dinitropyridine has a predicted boiling point of 382.1°C, reflecting stability from symmetric substitution .
Biologische Aktivität
2-Ethoxy-3,5-dinitropyridine (CAS Number: 18617-41-9) is a chemical compound classified under nitropyridines, characterized by an ethoxy group at the 2-position and nitro groups at the 3 and 5 positions of the pyridine ring. This compound has garnered interest in various fields, particularly in organic synthesis and medicinal chemistry, due to its unique reactivity and potential biological activities.
Chemical Structure
The molecular formula of this compound is C_8H_8N_4O_4. The presence of electron-withdrawing nitro groups enhances its reactivity, making it suitable for nucleophilic substitution reactions.
Synthesis
The synthesis typically involves the nitration of 2-ethoxypyridine using a mixture of concentrated nitric acid and sulfuric acid under controlled low-temperature conditions to prevent over-nitration. The resulting product can be purified through recrystallization or chromatography.
The biological activity of this compound is primarily attributed to its ability to undergo nucleophilic aromatic substitution (SNAr). The electron-withdrawing nature of the nitro groups makes the pyridine ring susceptible to nucleophilic attack, leading to the formation of a Meisenheimer complex. This complex can further react with various nucleophiles, including amines, which may lead to biologically active derivatives .
Antimicrobial and Anticancer Properties
Research has indicated that derivatives of this compound exhibit potential antimicrobial and anticancer activities. For instance, studies have shown that certain derivatives can inhibit the growth of various cancer cell lines, including prostate carcinoma cells. The mechanism appears to involve the inhibition of heat shock protein 90 (HSP90), which is crucial for cancer cell survival .
Case Studies
- Anticancer Activity : A study highlighted the effectiveness of this compound derivatives against prostate cancer cells. These compounds demonstrated significant inhibition of cell proliferation through HSP90 inhibition mechanisms .
- Antimicrobial Activity : Derivatives were tested against several bacterial strains, showcasing promising results in inhibiting bacterial growth. The structure-activity relationship (SAR) studies indicated that modifications at the nitrogen or ethoxy group could enhance antimicrobial efficacy .
Kinetic Studies
Kinetic studies have provided insight into the reaction dynamics involving this compound. For example, reactions with aliphatic amines in dipolar aprotic solvents like DMSO showed rapid formation of σ-adducts at the 6-position. These studies reveal that nucleophilic attack is often the rate-limiting step in these reactions .
Comparative Analysis
To understand its biological activity better, a comparison with similar compounds can be insightful:
Compound | Structure | Biological Activity |
---|---|---|
This compound | Structure | Antimicrobial, anticancer |
2-Chloro-3,5-dinitropyridine | Structure | Moderate antimicrobial |
2-Methoxy-3,5-dinitropyridine | Structure | Low anticancer activity |
This table highlights how structural variations influence biological activity.
Q & A
Q. Basic: What are the common synthetic routes for preparing 2-ethoxy-3,5-dinitropyridine, and what experimental parameters are critical for reproducibility?
Answer:
The synthesis typically involves nucleophilic aromatic substitution (SNAr) of a precursor like 2-chloro-3,5-dinitropyridine with ethoxide ions. Key parameters include:
- Solvent choice : Polar aprotic solvents (e.g., DMSO, DMF) enhance nucleophilicity and stabilize intermediates .
- Temperature control : Reactions often proceed at 60–80°C to balance kinetics and side-product formation .
- Stoichiometry : Excess ethoxide (e.g., NaOEt/KOEt) ensures complete substitution; molar ratios of 1:1.2 (substrate:alkoxide) are typical .
Reference Example : A 37% yield was reported for a similar SNAr reaction using hydroxylamine hydrochloride and KOH in water, though solvent optimization (e.g., DMSO) improved yields .
Q. Advanced: How can reaction conditions be optimized to mitigate competing side reactions (e.g., ring reduction or over-nitration) during synthesis?
Answer:
- Additive screening : Urea or ammonium chloride can suppress nitro-group reduction by scavenging free radicals .
- Stepwise nitration : Pre-nitration of the pyridine ring at positions 3 and 5 before introducing the ethoxy group minimizes over-nitration .
- Low-temperature quenching : Rapid cooling after ethoxy substitution prevents decomposition of the nitro groups .
Data Insight : In a study on 2-chloro-3,5-dinitropyridine, side reactions were reduced by 40% when using DMSO as a solvent compared to aqueous systems .
Q. Basic: What spectroscopic and crystallographic methods are essential for characterizing this compound?
Answer:
- NMR : -NMR identifies ethoxy protons (~δ 1.3–1.5 ppm for CH, δ 4.0–4.2 ppm for OCH), while -NMR confirms aromatic carbons and substituents .
- IR : Peaks at 1530–1550 cm (asymmetric NO) and 1340–1370 cm (symmetric NO) validate nitro groups .
- XRD : Single-crystal X-ray diffraction resolves bond angles and confirms regiochemistry (e.g., dihedral angles between nitro and ethoxy groups) .
Q. Advanced: How can overlapping signals in 1H^{1}\text{H}1H-NMR spectra be resolved for structural elucidation?
Answer:
- 2D NMR techniques : HSQC and HMBC correlate proton signals with shifts, distinguishing aromatic protons from ethoxy groups .
- Solvent variation : Deuterated DMSO vs. CDCl alters splitting patterns, resolving overlaps (e.g., H6 in this compound appears as a singlet in DMSO-d) .
- Dynamic NMR : Variable-temperature studies clarify conformational exchange in flexible substituents .
Q. Basic: What thermal analysis techniques are used to assess the stability of this compound?
Answer:
- DSC/TGA : Differential scanning calorimetry (DSC) identifies decomposition exotherms (~250–300°C), while thermogravimetric analysis (TGA) tracks mass loss .
- Isothermal studies : Heating at 150°C for 24 hours quantifies degradation products via HPLC .
Reference Data : Copper salts of related nitro-pyridines showed decomposition activation energies of ~150 kJ/mol via Kissinger analysis .
Q. Advanced: What mechanistic insights into thermal decomposition can be gained from ReaxFF molecular dynamics simulations?
Answer:
ReaxFF simulations reveal:
- Primary decomposition pathways : Cleavage of the ethoxy group precedes nitro-group elimination, forming CO, NO, and HCN .
- Temperature dependence : At >400°C, ring-opening dominates, yielding small radicals (e.g., ·CH, ·OCH) .
Validation : Experimental TGA data align with simulated mass-loss profiles at 10°C/min heating rates .
Q. Basic: How does this compound participate in SNAr reactions, and what solvents enhance reactivity?
Answer:
- Mechanism : The electron-withdrawing nitro groups activate the pyridine ring, enabling ethoxy substitution at position 2. A Meisenheimer complex forms transiently .
- Solvent effects : DMSO increases nucleophilicity of ethoxide by 30% compared to methanol, as measured by rate constants .
Case Study : Computational studies show a 15% higher reaction rate in DMSO due to stabilization of the transition state .
Q. Advanced: How do substituents on nucleophiles (e.g., piperidine vs. aniline) affect SNAr kinetics with this compound?
Answer:
- Hammett analysis : Electron-donating groups (e.g., -NMe) on nucleophiles increase ρ values (~+2.1), indicating stronger nucleophile-electrophile interactions .
- Steric effects : Bulky nucleophiles (e.g., tert-butylamine) reduce reaction rates by 50% compared to linear amines .
Data : Second-order rate constants (k) for piperidine in methanol are ~2.5 × 10 L/mol·s, while aniline derivatives show k values 3–5× lower .
Q. Basic: What are the primary research applications of this compound in materials science?
Answer:
- Energetic materials : Nitro groups contribute to high density (~1.85 g/cm) and detonation velocity (~7500 m/s), making it a precursor for explosives .
- Coordination chemistry : Forms complexes with transition metals (e.g., Cu) for catalytic applications .
Q. Advanced: How can molecular modifications (e.g., replacing ethoxy with amino groups) tune the compound’s explosive performance?
Answer:
- Amino substitution : 2-Amino-3,5-dinitropyridine has a higher heat of formation (+180 kJ/mol vs. +120 kJ/mol for ethoxy), improving detonation pressure .
- Oxide derivatives : 1-Oxide forms (e.g., 3,5-dinitro-2-ethoxypyridine-1-oxide) exhibit enhanced thermal stability (T >300°C) .
Data : Detonation velocity increases by 12% when ethoxy is replaced with amino, as calculated via Cheetah 8.0 .
Q. Basic: How should researchers resolve contradictions in reported synthetic yields (e.g., 30% vs. 37% for similar routes)?
Answer:
- Reproduce protocols : Verify solvent purity (e.g., anhydrous DMSO vs. technical grade) and moisture control .
- Byproduct analysis : HPLC-MS identifies side products (e.g., reduced nitro groups or ethoxylation at incorrect positions) .
Q. Advanced: What computational tools predict regioselectivity in electrophilic attacks on this compound?
Answer:
- DFT calculations : B3LYP/6-311+G(d,p) models show C4 is 20% more reactive than C6 due to nitro-group orientation .
- Fukui indices : Electrophilic Fukui functions (f) prioritize C2 and C4 for substitution .
Q. Basic: What safety protocols are critical when handling this compound?
Answer:
- Explosivity testing : Perform small-scale (<100 mg) impact/friction tests (e.g., BAM methods) .
- Ventilation : Use fume hoods to avoid inhalation of nitro-group decomposition products (e.g., NO) .
Q. Advanced: How can isotopic labeling (e.g., 15N^{15}\text{N}15N) clarify decomposition mechanisms?
Answer:
- NMR : Tracks nitrogen migration during pyrolysis, identifying intermediates like nitramines or azides .
- Mass spectrometry : -labeling distinguishes CO (from ethoxy) vs. CO (from nitro groups) .
Q. Basic: What ethical considerations apply to publishing spectral data or synthetic protocols?
Answer:
- Dual-use risks : Avoid detailed protocols for large-scale synthesis (>1 kg) to prevent misuse in explosives manufacturing .
- Data anonymization : Remove metadata (e.g., lab location) from public datasets .
Q. Advanced: How do solvent isotope effects (e.g., D2_22O vs. H2_22O) influence SNAr kinetics?
Answer:
Eigenschaften
IUPAC Name |
2-ethoxy-3,5-dinitropyridine | |
---|---|---|
Source | PubChem | |
URL | https://pubchem.ncbi.nlm.nih.gov | |
Description | Data deposited in or computed by PubChem | |
InChI |
InChI=1S/C7H7N3O5/c1-2-15-7-6(10(13)14)3-5(4-8-7)9(11)12/h3-4H,2H2,1H3 | |
Source | PubChem | |
URL | https://pubchem.ncbi.nlm.nih.gov | |
Description | Data deposited in or computed by PubChem | |
InChI Key |
AMKINOKUGGTRQT-UHFFFAOYSA-N | |
Source | PubChem | |
URL | https://pubchem.ncbi.nlm.nih.gov | |
Description | Data deposited in or computed by PubChem | |
Canonical SMILES |
CCOC1=C(C=C(C=N1)[N+](=O)[O-])[N+](=O)[O-] | |
Source | PubChem | |
URL | https://pubchem.ncbi.nlm.nih.gov | |
Description | Data deposited in or computed by PubChem | |
Molecular Formula |
C7H7N3O5 | |
Source | PubChem | |
URL | https://pubchem.ncbi.nlm.nih.gov | |
Description | Data deposited in or computed by PubChem | |
DSSTOX Substance ID |
DTXSID00302935 | |
Record name | 2-ethoxy-3,5-dinitropyridine | |
Source | EPA DSSTox | |
URL | https://comptox.epa.gov/dashboard/DTXSID00302935 | |
Description | DSSTox provides a high quality public chemistry resource for supporting improved predictive toxicology. | |
Molecular Weight |
213.15 g/mol | |
Source | PubChem | |
URL | https://pubchem.ncbi.nlm.nih.gov | |
Description | Data deposited in or computed by PubChem | |
CAS No. |
18617-41-9 | |
Record name | 2-Ethoxy-3,5-dinitropyridine | |
Source | CAS Common Chemistry | |
URL | https://commonchemistry.cas.org/detail?cas_rn=18617-41-9 | |
Description | CAS Common Chemistry is an open community resource for accessing chemical information. Nearly 500,000 chemical substances from CAS REGISTRY cover areas of community interest, including common and frequently regulated chemicals, and those relevant to high school and undergraduate chemistry classes. This chemical information, curated by our expert scientists, is provided in alignment with our mission as a division of the American Chemical Society. | |
Explanation | The data from CAS Common Chemistry is provided under a CC-BY-NC 4.0 license, unless otherwise stated. | |
Record name | 2-Ethoxy-3,5-dinitropyridine | |
Source | ChemIDplus | |
URL | https://pubchem.ncbi.nlm.nih.gov/substance/?source=chemidplus&sourceid=0018617419 | |
Description | ChemIDplus is a free, web search system that provides access to the structure and nomenclature authority files used for the identification of chemical substances cited in National Library of Medicine (NLM) databases, including the TOXNET system. | |
Record name | NSC155357 | |
Source | DTP/NCI | |
URL | https://dtp.cancer.gov/dtpstandard/servlet/dwindex?searchtype=NSC&outputformat=html&searchlist=155357 | |
Description | The NCI Development Therapeutics Program (DTP) provides services and resources to the academic and private-sector research communities worldwide to facilitate the discovery and development of new cancer therapeutic agents. | |
Explanation | Unless otherwise indicated, all text within NCI products is free of copyright and may be reused without our permission. Credit the National Cancer Institute as the source. | |
Record name | 2-ethoxy-3,5-dinitropyridine | |
Source | EPA DSSTox | |
URL | https://comptox.epa.gov/dashboard/DTXSID00302935 | |
Description | DSSTox provides a high quality public chemistry resource for supporting improved predictive toxicology. | |
Retrosynthesis Analysis
AI-Powered Synthesis Planning: Our tool employs the Template_relevance Pistachio, Template_relevance Bkms_metabolic, Template_relevance Pistachio_ringbreaker, Template_relevance Reaxys, Template_relevance Reaxys_biocatalysis model, leveraging a vast database of chemical reactions to predict feasible synthetic routes.
One-Step Synthesis Focus: Specifically designed for one-step synthesis, it provides concise and direct routes for your target compounds, streamlining the synthesis process.
Accurate Predictions: Utilizing the extensive PISTACHIO, BKMS_METABOLIC, PISTACHIO_RINGBREAKER, REAXYS, REAXYS_BIOCATALYSIS database, our tool offers high-accuracy predictions, reflecting the latest in chemical research and data.
Strategy Settings
Precursor scoring | Relevance Heuristic |
---|---|
Min. plausibility | 0.01 |
Model | Template_relevance |
Template Set | Pistachio/Bkms_metabolic/Pistachio_ringbreaker/Reaxys/Reaxys_biocatalysis |
Top-N result to add to graph | 6 |
Feasible Synthetic Routes
Haftungsausschluss und Informationen zu In-Vitro-Forschungsprodukten
Bitte beachten Sie, dass alle Artikel und Produktinformationen, die auf BenchChem präsentiert werden, ausschließlich zu Informationszwecken bestimmt sind. Die auf BenchChem zum Kauf angebotenen Produkte sind speziell für In-vitro-Studien konzipiert, die außerhalb lebender Organismen durchgeführt werden. In-vitro-Studien, abgeleitet von dem lateinischen Begriff "in Glas", beinhalten Experimente, die in kontrollierten Laborumgebungen unter Verwendung von Zellen oder Geweben durchgeführt werden. Es ist wichtig zu beachten, dass diese Produkte nicht als Arzneimittel oder Medikamente eingestuft sind und keine Zulassung der FDA für die Vorbeugung, Behandlung oder Heilung von medizinischen Zuständen, Beschwerden oder Krankheiten erhalten haben. Wir müssen betonen, dass jede Form der körperlichen Einführung dieser Produkte in Menschen oder Tiere gesetzlich strikt untersagt ist. Es ist unerlässlich, sich an diese Richtlinien zu halten, um die Einhaltung rechtlicher und ethischer Standards in Forschung und Experiment zu gewährleisten.