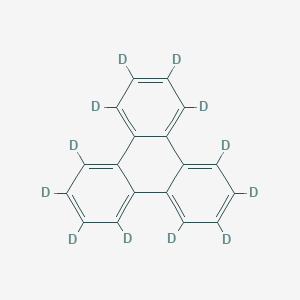
Triphenylen-d12
Übersicht
Beschreibung
Triphenylene-d12 is a deuterated form of triphenylene, a polycyclic aromatic hydrocarbon. The compound has the molecular formula C18D12 and a molecular weight of 240.36 g/mol . It consists of four fused benzene rings, forming a planar structure with delocalized 18-π-electron systems . This compound is often used in scientific research due to its unique properties and stability.
Wissenschaftliche Forschungsanwendungen
Material Science
1.1. Organic Light Emitting Diodes (OLEDs)
Triphenylene derivatives, including Triphenylene-d12, are utilized in the development of OLEDs due to their excellent luminescent properties and ability to form stable thin films. The incorporation of deuterated compounds can enhance the thermal and chemical stability of the materials used in OLEDs, leading to improved device performance and longevity .
1.2. Nanomaterials
Triphenylene-d12 is also significant in the synthesis of nanomaterials. Its planar structure allows for effective π-π stacking interactions, which are crucial for creating nanostructured materials with desirable electronic properties. Research has shown that using deuterated versions can influence the crystallinity and morphology of these materials, potentially leading to enhanced conductivity and mechanical strength .
Organic Electronics
2.1. Field-Effect Transistors (FETs)
In organic electronics, Triphenylene-d12 is explored as a semiconductor material in FETs. The presence of deuterium alters the vibrational modes of the molecules, which can lead to improved charge transport properties compared to their non-deuterated counterparts. Studies indicate that devices using Triphenylene-d12 exhibit higher mobility and stability under operational conditions .
2.2. Photovoltaics
The compound is being investigated for use in organic photovoltaic cells due to its ability to absorb light effectively and convert it into electrical energy. The deuterated form can enhance the photostability of the active layers, making it a promising candidate for next-generation solar cells .
Environmental Studies
3.1. Pollution Monitoring
Triphenylene-d12 serves as a tracer in environmental studies, particularly in monitoring polycyclic aromatic hydrocarbons (PAHs) in air and water samples. Its distinct isotopic signature allows researchers to track its behavior in various environmental conditions, providing insights into pollution sources and degradation pathways .
3.2. Toxicology Studies
Research involving Triphenylene-d12 has been pivotal in toxicology studies focused on PAHs' effects on human health and ecosystems. Its use as a model compound helps elucidate the mechanisms by which PAHs permeate biological membranes and their subsequent metabolic pathways within organisms .
Case Studies
Wirkmechanismus
Target of Action
Triphenylene-d12 is a deuterium-labeled version of triphenylene It’s known that deuterium-labeled compounds are often used as tracers in drug development processes .
Mode of Action
The incorporation of stable heavy isotopes like deuterium into drug molecules can potentially affect their interaction with targets . This can lead to changes in the pharmacokinetic and metabolic profiles of the drugs .
Biochemical Pathways
It’s worth noting that the parent compound, triphenylene, has been at the center of attention in unraveling the underlying molecular mass growth processes leading to complex polycyclic aromatic hydrocarbons (pahs) .
Pharmacokinetics
The deuterium substitution in drug molecules is known to potentially affect their pharmacokinetic profiles .
Result of Action
The use of deuterium-labeled compounds like triphenylene-d12 can provide valuable insights into the behavior of the parent compound in biological systems .
Action Environment
It’s important to note that the storage conditions can impact the stability of such compounds .
Vorbereitungsmethoden
Triphenylene-d12 can be synthesized through various methods. One common method involves the trimerization of benzyne . Another method includes trapping benzyne with a biphenyl derivative . Industrial production methods often involve the use of coal tar as a source of polycyclic aromatic hydrocarbons, from which triphenylene can be isolated .
Analyse Chemischer Reaktionen
Triphenylene-d12 undergoes several types of chemical reactions, including:
Oxidation: This reaction typically involves the use of strong oxidizing agents such as potassium permanganate or chromic acid, leading to the formation of quinones.
Reduction: Reduction reactions can be carried out using hydrogen gas in the presence of a metal catalyst like palladium, resulting in the formation of partially hydrogenated derivatives.
Vergleich Mit ähnlichen Verbindungen
Triphenylene-d12 can be compared with other similar compounds such as:
Chrysene-d12: Another deuterated polycyclic aromatic hydrocarbon with a similar structure but different electronic properties.
Perylene-d12: A larger polycyclic aromatic hydrocarbon with additional fused benzene rings, leading to different chemical reactivity and applications.
Anthracene-d10: A smaller polycyclic aromatic hydrocarbon with three fused benzene rings, used in similar research applications but with distinct properties
Triphenylene-d12 stands out due to its unique combination of stability, planar structure, and delocalized electron systems, making it a valuable compound in various scientific and industrial applications.
Biologische Aktivität
Triphenylene-d12 is a deuterated form of triphenylene, a polycyclic aromatic hydrocarbon (PAH) known for its complex biological interactions. This article aims to provide a comprehensive overview of the biological activity associated with Triphenylene-d12, including its toxicity, metabolic pathways, and potential environmental impacts.
Overview of Triphenylene-d12
Triphenylene is composed of four fused benzene rings, making it a member of the PAH family. The introduction of deuterium atoms in Triphenylene-d12 alters its physical and chemical properties, which can influence its biological behavior. The compound is often studied for its environmental persistence and potential toxicological effects.
Acute and Chronic Toxicity
Research indicates that PAHs, including Triphenylene-d12, can exhibit significant toxicity through various mechanisms:
- Genotoxicity : Triphenylene and its derivatives can cause DNA damage and mutations. This is primarily due to their metabolic activation, which produces reactive intermediates capable of forming DNA adducts .
- Carcinogenicity : The carcinogenic potential of PAHs is well-documented. Triphenylene has been implicated in cancer development through mechanisms involving oxidative stress and DNA adduct formation .
- Developmental and Reproductive Toxicity : Studies have shown that exposure to PAHs can adversely affect developmental processes in aquatic organisms, leading to teratogenic effects .
Metabolic Pathways
The metabolism of Triphenylene-d12 involves several enzymatic pathways, primarily mediated by cytochrome P450 enzymes. These enzymes facilitate the conversion of Triphenylene-d12 into more water-soluble metabolites, which can be excreted from organisms. However, this metabolic process can also lead to the formation of toxic intermediates that interact with cellular macromolecules:
- Activation : Initial oxidation by cytochrome P450 results in the formation of epoxides.
- Detoxification : Subsequent conjugation with glutathione or glucuronic acid aids in detoxifying these intermediates .
- Reactive Intermediates : Certain metabolites may escape detoxification and bind to DNA, resulting in mutagenic changes.
Environmental Impact
Triphenylene-d12's stability and resistance to degradation make it a persistent environmental contaminant. Its presence in sediments and biota raises concerns regarding ecological health:
- Bioaccumulation : Studies indicate that PAHs can accumulate in aquatic organisms, leading to increased toxicity at higher trophic levels .
- Sediment Quality : The concentration of Triphenylene-d12 in sediments has been correlated with adverse biological effects on benthic organisms, indicating its role as a pollutant .
Case Study 1: Sediment Analysis
A study conducted on high mountain lakes revealed significant levels of PAHs, including Triphenylene-d12. The research assessed the toxic effects based on sediment quality guidelines:
Compound | Concentration (ng/g) | Toxicity Contribution (%) |
---|---|---|
Chrysene | 250 | 14 |
Triphenylene | 150 | 17 |
Dibenz[a,h]anthracene | 100 | 22 |
The findings indicated that these compounds significantly contributed to sediment toxicity and potential ecological risks .
Case Study 2: Aquatic Toxicity Assessment
In another study focusing on aquatic organisms exposed to sediment contaminated with PAHs, including Triphenylene-d12, researchers observed:
- Increased Mortality Rates : Fish exposed to contaminated sediments showed higher mortality rates compared to control groups.
- Behavioral Changes : Altered swimming patterns were noted, indicative of neurotoxic effects.
These studies underscore the importance of monitoring PAH levels in aquatic environments to mitigate ecological risks .
Eigenschaften
IUPAC Name |
1,2,3,4,5,6,7,8,9,10,11,12-dodecadeuteriotriphenylene | |
---|---|---|
Source | PubChem | |
URL | https://pubchem.ncbi.nlm.nih.gov | |
Description | Data deposited in or computed by PubChem | |
InChI |
InChI=1S/C18H12/c1-2-8-14-13(7-1)15-9-3-4-11-17(15)18-12-6-5-10-16(14)18/h1-12H/i1D,2D,3D,4D,5D,6D,7D,8D,9D,10D,11D,12D | |
Source | PubChem | |
URL | https://pubchem.ncbi.nlm.nih.gov | |
Description | Data deposited in or computed by PubChem | |
InChI Key |
SLGBZMMZGDRARJ-AQZSQYOVSA-N | |
Source | PubChem | |
URL | https://pubchem.ncbi.nlm.nih.gov | |
Description | Data deposited in or computed by PubChem | |
Canonical SMILES |
C1=CC=C2C(=C1)C3=CC=CC=C3C4=CC=CC=C24 | |
Source | PubChem | |
URL | https://pubchem.ncbi.nlm.nih.gov | |
Description | Data deposited in or computed by PubChem | |
Isomeric SMILES |
[2H]C1=C(C(=C2C(=C1[2H])C3=C(C(=C(C(=C3C4=C(C(=C(C(=C24)[2H])[2H])[2H])[2H])[2H])[2H])[2H])[2H])[2H])[2H] | |
Source | PubChem | |
URL | https://pubchem.ncbi.nlm.nih.gov | |
Description | Data deposited in or computed by PubChem | |
Molecular Formula |
C18H12 | |
Source | PubChem | |
URL | https://pubchem.ncbi.nlm.nih.gov | |
Description | Data deposited in or computed by PubChem | |
Molecular Weight |
240.4 g/mol | |
Source | PubChem | |
URL | https://pubchem.ncbi.nlm.nih.gov | |
Description | Data deposited in or computed by PubChem | |
Synthesis routes and methods I
Procedure details
Synthesis routes and methods II
Procedure details
Synthesis routes and methods III
Procedure details
Retrosynthesis Analysis
AI-Powered Synthesis Planning: Our tool employs the Template_relevance Pistachio, Template_relevance Bkms_metabolic, Template_relevance Pistachio_ringbreaker, Template_relevance Reaxys, Template_relevance Reaxys_biocatalysis model, leveraging a vast database of chemical reactions to predict feasible synthetic routes.
One-Step Synthesis Focus: Specifically designed for one-step synthesis, it provides concise and direct routes for your target compounds, streamlining the synthesis process.
Accurate Predictions: Utilizing the extensive PISTACHIO, BKMS_METABOLIC, PISTACHIO_RINGBREAKER, REAXYS, REAXYS_BIOCATALYSIS database, our tool offers high-accuracy predictions, reflecting the latest in chemical research and data.
Strategy Settings
Precursor scoring | Relevance Heuristic |
---|---|
Min. plausibility | 0.01 |
Model | Template_relevance |
Template Set | Pistachio/Bkms_metabolic/Pistachio_ringbreaker/Reaxys/Reaxys_biocatalysis |
Top-N result to add to graph | 6 |
Feasible Synthetic Routes
Q1: What spectroscopic techniques are particularly insightful when studying triphenylene-d12, and what information can be gained?
A1: Both Electric Field Nuclear Magnetic Resonance (EFNMR) and fluorescence spectroscopy provide valuable information about triphenylene-d12. EFNMR studies on triphenylene-d12 successfully determined the anisotropy (Δα) of its static electric polarizability tensor to be -(14.4 ± 1.4) × 10-24 cm3. [] This technique reveals information about the molecule's response to electric fields. On the other hand, fluorescence spectroscopy, specifically comparing the spectra of triphenylene-d12 with its non-deuterated counterpart, helps understand the impact of deuteration on excited state dynamics. For instance, researchers observed a significant weakening of vibronic bands in the high energy region of the fluorescence excitation spectrum of triphenylene-d12, suggesting enhanced radiationless transitions, potentially due to internal conversion to the ground state. []
Q2: How does deuteration in triphenylene-d12 influence its photophysical properties compared to triphenylene?
A2: Deuteration in triphenylene-d12 plays a crucial role in altering its excited state behavior. While both triphenylene and its deuterated form share a symmetry-allowed S1 (1A1) ← S0 (1A1) transition, the deuterated variant exhibits weaker vibronic bands at higher energy levels. [] This difference points towards faster radiationless decay in triphenylene-d12, likely due to a more efficient internal conversion process facilitated by the presence of deuterium. This insight highlights the impact of isotopic substitution on the photophysics of aromatic hydrocarbons.
Q3: Can you elaborate on the significance of studying triplet state kinetics in triphenylene-d12?
A3: Investigating the kinetics of energy transfer from the triplet state of triphenylene-d12 to an acceptor molecule like Rhodamine B provides valuable insights into the nature of the interaction governing the energy transfer process. [] By analyzing the decay of triphenylene-d12's phosphorescence in the presence of Rhodamine B, researchers can assess the "purity" of the dipole-dipole interaction, typically assumed to drive triplet-singlet energy transfer. This analysis relies on fitting the decay data to theoretical models, allowing for the determination of the distance dependence parameter (n) in the energy transfer process.
Q4: What kind of information can Electron Paramagnetic Resonance (EPR) spectroscopy provide about triphenylene-d12?
A4: EPR studies on triphenylene-d12, when oriented within a host crystal of sym-dodecahydrotriphenylene, reveal crucial details about its triplet state properties. [] These studies confirmed multiple orientations of triphenylene-d12 within the host matrix and identified distinct mixed crystal forms based on observed zero-field splitting parameters. This technique provides a deeper understanding of the molecule's electronic structure and its interaction with the surrounding environment in its excited triplet state.
Haftungsausschluss und Informationen zu In-Vitro-Forschungsprodukten
Bitte beachten Sie, dass alle Artikel und Produktinformationen, die auf BenchChem präsentiert werden, ausschließlich zu Informationszwecken bestimmt sind. Die auf BenchChem zum Kauf angebotenen Produkte sind speziell für In-vitro-Studien konzipiert, die außerhalb lebender Organismen durchgeführt werden. In-vitro-Studien, abgeleitet von dem lateinischen Begriff "in Glas", beinhalten Experimente, die in kontrollierten Laborumgebungen unter Verwendung von Zellen oder Geweben durchgeführt werden. Es ist wichtig zu beachten, dass diese Produkte nicht als Arzneimittel oder Medikamente eingestuft sind und keine Zulassung der FDA für die Vorbeugung, Behandlung oder Heilung von medizinischen Zuständen, Beschwerden oder Krankheiten erhalten haben. Wir müssen betonen, dass jede Form der körperlichen Einführung dieser Produkte in Menschen oder Tiere gesetzlich strikt untersagt ist. Es ist unerlässlich, sich an diese Richtlinien zu halten, um die Einhaltung rechtlicher und ethischer Standards in Forschung und Experiment zu gewährleisten.