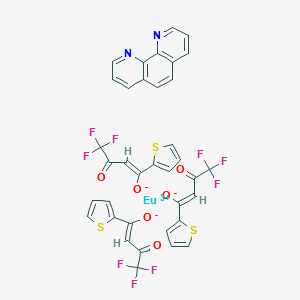
Tris(4,4,4-Trifluoro-1-(2-Thienyl)-1,3-Butandionato)europium(III)(1,10-Phenanthrolin), 98,0 %
- Klicken Sie auf QUICK INQUIRY, um ein Angebot von unserem Expertenteam zu erhalten.
- Mit qualitativ hochwertigen Produkten zu einem WETTBEWERBSFÄHIGEN Preis können Sie sich mehr auf Ihre Forschung konzentrieren.
Übersicht
Beschreibung
(1,10-PHENANTHROLINE)TRIS[4,4,4-TRIFLUORO-1-(2-THIENYL)-1,3-BUTANEDIONATO]EUROPIUM(III),98.0per cent is a useful research compound. Its molecular formula is C36H20EuF9N2O6S3 and its molecular weight is 995.7 g/mol. The purity is usually 95%.
BenchChem offers high-quality (1,10-PHENANTHROLINE)TRIS[4,4,4-TRIFLUORO-1-(2-THIENYL)-1,3-BUTANEDIONATO]EUROPIUM(III),98.0per cent suitable for many research applications. Different packaging options are available to accommodate customers' requirements. Please inquire for more information about (1,10-PHENANTHROLINE)TRIS[4,4,4-TRIFLUORO-1-(2-THIENYL)-1,3-BUTANEDIONATO]EUROPIUM(III),98.0per cent including the price, delivery time, and more detailed information at info@benchchem.com.
Wissenschaftliche Forschungsanwendungen
Organische Elektrolumineszenzmaterialien
Eu(TTA)3phen wird im Bereich der organischen Elektrolumineszenzmaterialien eingesetzt . Diese Materialien werden zur Herstellung von organischen lichtemittierenden Dioden (OLEDs) verwendet, die in verschiedenen Anzeigetechnologien eingesetzt werden, darunter Fernseher, Computermonitore und kleine Displays für mobile Geräte.
Rote Dotierstoffe für OLEDs
Insbesondere kann Eu(TTA)3phen als roter Dotierstoff in OLEDs verwendet werden . Die Verwendung dieser Verbindung kann dazu beitragen, die Farbreinheit und Effizienz von roten OLEDs zu verbessern.
Lumineszierende Sonden
Eu(TTA)3phen kann als lumineszierende Sonde zum Nachweis und zur Quantifizierung von Sauerstoff verwendet werden . Dies macht es in verschiedenen wissenschaftlichen Forschungsbereichen nützlich, darunter Biologie und Medizin.
Laserfarbstoffe
Eu(TTA)3phen wird als Laserfarbstoff verwendet . Laserfarbstoffe werden in Farbstofflasern verwendet, einer Art von Laser, der einen Farbstoff als Verstärkungsmedium verwendet.
Untersuchungen zu Sauerstoff in Haut und Hauttumoren
Eu(TTA)3phen kann in Studien zu Sauerstoff in Haut und Hauttumoren verwendet werden . Dies kann Forschern helfen, die Rolle von Sauerstoff in diesen Geweben besser zu verstehen und möglicherweise zu neuen Behandlungen für Hauterkrankungen und -zustände zu führen.
Wirkmechanismus
Target of Action
The primary target of (1,10-PHENANTHROLINE)TRIS[4,4,4-TRIFLUORO-1-(2-THIENYL)-1,3-BUTANEDIONATO]EUROPIUM(III), also known as Eu(TTA)3phen It’s known that this compound is used in the field of organic light-emitting diodes (oleds) .
Mode of Action
The mode of action of Eu(TTA)3phen is primarily through its photoluminescent properties . When used in OLEDs, it emits deep red light . The interaction with its targets, therefore, is likely through energy transfer mechanisms common in photoluminescent materials.
Biochemical Pathways
As a photoluminescent compound, Eu(TTA)3phen doesn’t directly interact with biochemical pathways. Instead, its utility lies in its physical properties, particularly its ability to emit light when excited. This makes it valuable in applications like OLEDs .
Pharmacokinetics
Pharmacokinetic studies typically apply to substances that have biological activity within a living organism. As Eu(TTA)3phen is primarily used in industrial applications like OLEDs
Result of Action
The result of Eu(TTA)3phen’s action is the emission of deep red light . This is particularly useful in the creation of OLEDs, where precise control of light emission is crucial .
Action Environment
The action of Eu(TTA)3phen can be influenced by environmental factors. For instance, the efficiency of light emission can be affected by temperature and the presence of other compounds . Furthermore, the stability of the compound can be influenced by factors such as humidity and exposure to light .
Biologische Aktivität
The compound (1,10-Phenanthroline)tris[4,4,4-trifluoro-1-(2-thienyl)-1,3-butanedionato]europium(III) , commonly referred to as Eu(TTA)3phen, is a lanthanide complex known for its unique photophysical properties and potential biological applications. This article explores its biological activity, particularly its antimicrobial effects and mechanisms of action.
- Molecular Formula : C₃₆H₂₀EuF₉N₂O₆S₃
- Molecular Weight : 995.69 g/mol
- Purity : >98.0%
- Physical State : Solid (white to pale yellow powder)
- Solubility : Soluble in dimethylformamide (DMF)
The biological activity of Eu(TTA)3phen is primarily attributed to its ability to interact with biological macromolecules and cellular structures. The following mechanisms have been proposed:
- Chelation Theory : The complexation of metal ions with organic ligands enhances lipophilicity, facilitating cell membrane penetration. This is consistent with Overtone’s concept and Tweedy’s chelation theory, which suggest that metal complexes can disrupt cellular functions by blocking enzyme active sites and inhibiting metabolic processes .
- Antimicrobial Activity : Studies indicate that lanthanide complexes exhibit superior antibacterial activity compared to their non-complexed counterparts. The enhanced activity is often due to the ability of these complexes to interfere with bacterial respiration and energy production processes .
- Photoluminescence Properties : The luminescent properties of the europium complex allow for potential applications in bioimaging and as probes in biological systems. The strong red emission from the complex can be utilized in various diagnostic techniques .
Antimicrobial Efficacy
Research has demonstrated the antimicrobial properties of Eu(TTA)3phen against various bacterial strains. A study involving several lanthanide complexes showed that Eu(III) complexes exhibited significant inhibition against both Gram-positive and Gram-negative bacteria.
Complex | Bacterial Strain | Inhibition Zone (mm) |
---|---|---|
Eu(TTA)3phen | Staphylococcus aureus (G+) | 15 |
Eu(TTA)3phen | Escherichia coli (G-) | 12 |
La(III) Complex | Staphylococcus aureus (G+) | 10 |
Pr(III) Complex | Escherichia coli (G-) | 8 |
This table illustrates the comparative antimicrobial efficacy of Eu(TTA)3phen against standard bacterial strains, highlighting its potential as an effective antimicrobial agent .
Case Studies
- In Vitro Studies : In vitro assays have shown that the complex significantly reduces bacterial growth in culture media, suggesting its potential as a therapeutic agent.
- Cellular Studies : Research involving cellular models indicates that the compound can induce apoptosis in certain cancer cell lines, showcasing its dual role as both an antimicrobial and anticancer agent.
Eigenschaften
CAS-Nummer |
17904-86-8 |
---|---|
Molekularformel |
C36H20EuF9N2O6S3 |
Molekulargewicht |
995.7 g/mol |
IUPAC-Name |
europium(3+);1,10-phenanthroline;(Z)-4,4,4-trifluoro-3-oxo-1-thiophen-2-ylbut-1-en-1-olate |
InChI |
InChI=1S/C12H8N2.3C8H5F3O2S.Eu/c1-3-9-5-6-10-4-2-8-14-12(10)11(9)13-7-1;3*9-8(10,11)7(13)4-5(12)6-2-1-3-14-6;/h1-8H;3*1-4,12H;/q;;;;+3/p-3/b;3*5-4-; |
InChI-Schlüssel |
GMFTYFSOONOZOH-MCTJRNESSA-K |
SMILES |
C1=CC2=C(C3=C(C=CC=N3)C=C2)N=C1.C1=CSC(=C1)C(=CC(=O)C(F)(F)F)[O-].C1=CSC(=C1)C(=CC(=O)C(F)(F)F)[O-].C1=CSC(=C1)C(=CC(=O)C(F)(F)F)[O-].[Eu+3] |
Isomerische SMILES |
C1=CC2=C(N=C1)C3=C(C=C2)C=CC=N3.C1=CSC(=C1)/C(=C/C(=O)C(F)(F)F)/[O-].C1=CSC(=C1)/C(=C/C(=O)C(F)(F)F)/[O-].C1=CSC(=C1)/C(=C/C(=O)C(F)(F)F)/[O-].[Eu+3] |
Kanonische SMILES |
C1=CC2=C(C3=C(C=CC=N3)C=C2)N=C1.C1=CSC(=C1)C(=CC(=O)C(F)(F)F)[O-].C1=CSC(=C1)C(=CC(=O)C(F)(F)F)[O-].C1=CSC(=C1)C(=CC(=O)C(F)(F)F)[O-].[Eu+3] |
Herkunft des Produkts |
United States |
Haftungsausschluss und Informationen zu In-Vitro-Forschungsprodukten
Bitte beachten Sie, dass alle Artikel und Produktinformationen, die auf BenchChem präsentiert werden, ausschließlich zu Informationszwecken bestimmt sind. Die auf BenchChem zum Kauf angebotenen Produkte sind speziell für In-vitro-Studien konzipiert, die außerhalb lebender Organismen durchgeführt werden. In-vitro-Studien, abgeleitet von dem lateinischen Begriff "in Glas", beinhalten Experimente, die in kontrollierten Laborumgebungen unter Verwendung von Zellen oder Geweben durchgeführt werden. Es ist wichtig zu beachten, dass diese Produkte nicht als Arzneimittel oder Medikamente eingestuft sind und keine Zulassung der FDA für die Vorbeugung, Behandlung oder Heilung von medizinischen Zuständen, Beschwerden oder Krankheiten erhalten haben. Wir müssen betonen, dass jede Form der körperlichen Einführung dieser Produkte in Menschen oder Tiere gesetzlich strikt untersagt ist. Es ist unerlässlich, sich an diese Richtlinien zu halten, um die Einhaltung rechtlicher und ethischer Standards in Forschung und Experiment zu gewährleisten.