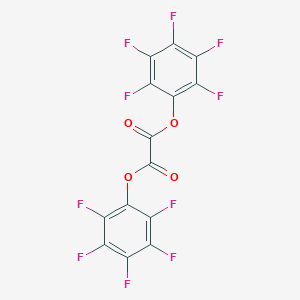
Bis(pentafluorophenyl)oxalat
Übersicht
Beschreibung
Bis(pentafluorophenyl) oxalate is a chemical compound with the molecular formula C₁₄F₁₀O₄ and a molecular weight of 422.13 g/mol . It is commonly used as a chemiluminescence reagent, which means it emits light as a result of a chemical reaction . This compound is particularly known for its application in high-performance liquid chromatography (HPLC) and capillary electrophoresis (CE) due to its sensitivity in detecting fluorescent compounds .
Wissenschaftliche Forschungsanwendungen
Applications in Organic Synthesis
BPFO serves as a critical reagent in organic synthesis due to its unique structural properties, which allow it to participate in various chemical reactions.
2.1. Chemiluminescence
One of the notable applications of BPFO is in chemiluminescent systems. The compound can be utilized to generate light through reactions with hydrogen peroxide, forming high-energy intermediates that emit light upon decomposition. This property has been exploited in several analytical applications, including:
- Detection of Oxalic Acid: BPFO has been used in the detection of oxalic acid in biological samples through chemiluminescence, demonstrating its utility as an analytical reagent .
- Immunoassays: Its stability and efficiency make it suitable for chemiluminescence immunoassays, where light emission is used to quantify analytes .
2.2. Synthesis of Functionalized Compounds
BPFO can also act as a precursor for synthesizing various functionalized compounds, which are essential in developing pharmaceuticals and agrochemicals. Its reactivity allows for the introduction of diverse functional groups through nucleophilic substitution reactions.
Applications in Materials Science
In materials science, BPFO is explored for its potential in developing advanced materials with unique properties.
3.1. Polymer Chemistry
BPFO is investigated as a cross-linking agent in polymer synthesis:
- Fluorinated Polymers: The incorporation of BPFO into polymer matrices can enhance thermal stability and chemical resistance, making it suitable for applications in harsh environments.
3.2. Nanomaterials
Research has indicated that BPFO can be utilized to create nanomaterials with tailored properties:
- Nanocomposites: By combining BPFO with nanoparticles, researchers have developed nanocomposites that exhibit improved mechanical and thermal properties.
Biochemical Applications
BPFO's role extends into biochemistry where it is used as a reagent for various assays.
4.1. Molecular Biology Techniques
BPFO has been employed in several molecular biology techniques:
- Nucleic Acid Gel Electrophoresis: It is used as a component in gel formulations for the separation of nucleic acids .
- Protein Purification: BPFO aids in the purification processes by facilitating the separation of proteins based on their charge and size .
5.1. Chemiluminescence Studies
A study conducted by Chokshi et al. demonstrated the effectiveness of BPFO in generating chemiluminescent signals when reacted with hydrogen peroxide under controlled conditions. The results indicated that the quantum yield of light emission was significantly influenced by the concentration of hydrogen peroxide, establishing BPFO as a reliable reagent for analytical applications .
5.2. Polymer Development
Research on fluorinated polymers incorporating BPFO revealed enhanced properties such as increased thermal stability and reduced flammability compared to traditional polymers. These findings suggest potential applications in industries requiring high-performance materials .
Data Table: Summary of Applications
Application Area | Specific Uses | Key Benefits |
---|---|---|
Organic Synthesis | Chemiluminescence reactions | High sensitivity and specificity |
Synthesis of functionalized compounds | Versatile reactivity | |
Materials Science | Cross-linking agent for polymers | Enhanced thermal stability |
Nanocomposite development | Improved mechanical properties | |
Biochemistry | Nucleic acid gel electrophoresis | Efficient separation |
Protein purification | Facilitates protein isolation |
Wirkmechanismus
Target of Action
Bis(pentafluorophenyl) Oxalate, also known as PFPO , is primarily used as a chemiluminescence reagent . Its primary targets are fluorescent compounds , which it interacts with to produce a chemiluminescent reaction .
Mode of Action
The compound works by reacting with these fluorescent compounds in the presence of hydrogen peroxide . This reaction results in the emission of light, a process known as chemiluminescence . The emitted light can then be detected and measured, providing valuable information about the target compounds.
Biochemical Pathways
energy transfer processes . In these processes, the energy released from the reaction between Bis(pentafluorophenyl) Oxalate and a target compound is transferred to a fluorophore, causing it to emit light .
Result of Action
The primary result of Bis(pentafluorophenyl) Oxalate’s action is the production of light through chemiluminescence . This light can be measured and used to quantify the presence of target compounds, making Bis(pentafluorophenyl) Oxalate a valuable tool in various research and diagnostic applications.
Action Environment
The efficacy and stability of Bis(pentafluorophenyl) Oxalate can be influenced by various environmental factors. For instance, the chemiluminescent reaction it catalyzes requires the presence of hydrogen peroxide . Additionally, factors such as temperature, pH, and the presence of other chemicals can potentially impact the efficiency of this reaction.
Vorbereitungsmethoden
Synthetic Routes and Reaction Conditions
Bis(pentafluorophenyl) oxalate can be synthesized through the reaction of oxalyl chloride with pentafluorophenol in the presence of a base such as pyridine . The reaction typically occurs under anhydrous conditions to prevent hydrolysis of the reactants and products. The general reaction scheme is as follows:
C2O2Cl2+2C6F5OH→C2O4(C6F5)2+2HCl
Industrial Production Methods
In industrial settings, the production of bis(pentafluorophenyl)oxalate may involve continuous flow processes to enhance efficiency and yield. The use of automated systems ensures precise control over reaction conditions such as temperature, pressure, and reactant concentrations .
Analyse Chemischer Reaktionen
Types of Reactions
Bis(pentafluorophenyl) oxalate primarily undergoes chemiluminescent reactions. When it reacts with hydrogen peroxide in the presence of a fluorescent dye such as 9,10-diphenylanthracene, it produces light . This reaction is highly sensitive and is used in various analytical applications.
Common Reagents and Conditions
Hydrogen Peroxide (H₂O₂): Acts as an oxidizing agent.
Fluorescent Dyes: Such as 9,10-diphenylanthracene, which emit light upon excitation.
Solvents: Organic solvents like acetonitrile or dichloromethane are commonly used.
Major Products
The major product of the chemiluminescent reaction involving bis(pentafluorophenyl)oxalate is light emission at a wavelength of approximately 430 nm .
Vergleich Mit ähnlichen Verbindungen
Bis(pentafluorophenyl) oxalate is unique due to its high sensitivity and efficiency in chemiluminescent reactions. Similar compounds include:
Bis(2,4,6-trichlorophenyl)oxalate: Another chemiluminescent reagent but less sensitive compared to bis(pentafluorophenyl)oxalate.
Bis(4-nitrophenyl)oxalate: Used in similar applications but has different emission characteristics.
These comparisons highlight the superior performance of bis(pentafluorophenyl)oxalate in terms of sensitivity and efficiency in chemiluminescent applications.
Biologische Aktivität
Bis(pentafluorophenyl) oxalate (BPFO) is an organic compound with the molecular formula CFO and a CAS number of 16536-48-4. It has garnered attention in various fields, particularly in biochemical research due to its unique properties and potential applications. This article focuses on the biological activity of BPFO, exploring its mechanisms, applications, and implications in biological systems.
- Molecular Weight : 422.131 g/mol
- Density : 1.8 ± 0.1 g/cm³
- Boiling Point : 318.9 ± 42.0 °C at 760 mmHg
- Flash Point : 141.9 ± 22.8 °C
These properties indicate that BPFO is a stable compound under normal laboratory conditions, making it suitable for various experimental applications.
BPFO is primarily recognized for its role in chemiluminescence reactions, particularly when combined with hydrogen peroxide and fluorescent compounds. The mechanism involves the generation of reactive intermediates that lead to light emission, a process extensively studied for applications in analytical chemistry and bioluminescence assays.
Chemiluminescence Reaction
The chemiluminescent reaction involving BPFO typically follows these steps:
- Formation of Reactive Intermediates : Upon reaction with hydrogen peroxide, BPFO generates peracids which decompose to produce light-emitting species.
- Emission of Light : The excited state of these species results in the emission of photons, which can be quantified for analytical purposes.
1. Analytical Chemistry
BPFO is utilized as a reagent in high-performance liquid chromatography (HPLC) for detecting various analytes through chemiluminescence detection systems. Its effectiveness in different pH ranges makes it versatile for various analytical applications .
2. Biochemical Assays
As a biochemical reagent, BPFO has been employed in assays related to:
- Cell Cycle Analysis : Investigating the effects of compounds on cell proliferation.
- Apoptosis Studies : Evaluating mechanisms of programmed cell death.
- Immunological Research : Studying immune responses and signaling pathways .
Case Study 1: Chemiluminescent Detection
A study evaluated the chemiluminescent properties of BPFO in combination with hydrogen peroxide and fluorescent compounds like 9,10-diphenylanthracene (DPA). The results indicated that BPFO provided high quantum yields for light emission, making it an effective choice for luminescent assays .
Case Study 2: Oxalate Metabolism
Research has highlighted the metabolic pathways involving oxalates, including those derived from BPFO. Studies on lactic acid bacteria demonstrated their capability to degrade oxalates effectively, suggesting potential health benefits related to oxalate metabolism and kidney stone prevention .
Implications for Health and Disease
The biological activity of BPFO extends to its potential implications in health sciences:
- Kidney Stone Formation : Understanding how compounds like BPFO interact with metabolic pathways can inform strategies to mitigate oxalate-related disorders.
- Therapeutic Applications : The ability to manipulate chemiluminescence may lead to advancements in diagnostic tools and therapeutic agents targeting specific biological pathways.
Eigenschaften
IUPAC Name |
bis(2,3,4,5,6-pentafluorophenyl) oxalate | |
---|---|---|
Source | PubChem | |
URL | https://pubchem.ncbi.nlm.nih.gov | |
Description | Data deposited in or computed by PubChem | |
InChI |
InChI=1S/C14F10O4/c15-1-3(17)7(21)11(8(22)4(1)18)27-13(25)14(26)28-12-9(23)5(19)2(16)6(20)10(12)24 | |
Source | PubChem | |
URL | https://pubchem.ncbi.nlm.nih.gov | |
Description | Data deposited in or computed by PubChem | |
InChI Key |
YMTUYHWOWDFXOX-UHFFFAOYSA-N | |
Source | PubChem | |
URL | https://pubchem.ncbi.nlm.nih.gov | |
Description | Data deposited in or computed by PubChem | |
Canonical SMILES |
C1(=C(C(=C(C(=C1F)F)F)F)F)OC(=O)C(=O)OC2=C(C(=C(C(=C2F)F)F)F)F | |
Source | PubChem | |
URL | https://pubchem.ncbi.nlm.nih.gov | |
Description | Data deposited in or computed by PubChem | |
Molecular Formula |
C14F10O4 | |
Source | PubChem | |
URL | https://pubchem.ncbi.nlm.nih.gov | |
Description | Data deposited in or computed by PubChem | |
DSSTOX Substance ID |
DTXSID60371102 | |
Record name | Bis(pentafluorophenyl) oxalate | |
Source | EPA DSSTox | |
URL | https://comptox.epa.gov/dashboard/DTXSID60371102 | |
Description | DSSTox provides a high quality public chemistry resource for supporting improved predictive toxicology. | |
Molecular Weight |
422.13 g/mol | |
Source | PubChem | |
URL | https://pubchem.ncbi.nlm.nih.gov | |
Description | Data deposited in or computed by PubChem | |
CAS No. |
16536-48-4 | |
Record name | Bis(pentafluorophenyl) oxalate | |
Source | EPA DSSTox | |
URL | https://comptox.epa.gov/dashboard/DTXSID60371102 | |
Description | DSSTox provides a high quality public chemistry resource for supporting improved predictive toxicology. | |
Retrosynthesis Analysis
AI-Powered Synthesis Planning: Our tool employs the Template_relevance Pistachio, Template_relevance Bkms_metabolic, Template_relevance Pistachio_ringbreaker, Template_relevance Reaxys, Template_relevance Reaxys_biocatalysis model, leveraging a vast database of chemical reactions to predict feasible synthetic routes.
One-Step Synthesis Focus: Specifically designed for one-step synthesis, it provides concise and direct routes for your target compounds, streamlining the synthesis process.
Accurate Predictions: Utilizing the extensive PISTACHIO, BKMS_METABOLIC, PISTACHIO_RINGBREAKER, REAXYS, REAXYS_BIOCATALYSIS database, our tool offers high-accuracy predictions, reflecting the latest in chemical research and data.
Strategy Settings
Precursor scoring | Relevance Heuristic |
---|---|
Min. plausibility | 0.01 |
Model | Template_relevance |
Template Set | Pistachio/Bkms_metabolic/Pistachio_ringbreaker/Reaxys/Reaxys_biocatalysis |
Top-N result to add to graph | 6 |
Feasible Synthetic Routes
Q1: What role does Bis(pentafluorophenyl)oxalate play in the detection of estradiol in plasma?
A: Bis(pentafluorophenyl)oxalate (PFPO) acts as a chemiluminescent reagent in the detection method described in the research paper []. It is not a target of estradiol or involved in its biological pathways. Instead, PFPO participates in a chemical reaction that generates light when it reacts with a derivative of estradiol (dansylated-estradiol) in the presence of hydrogen peroxide. This light emission is then measured to determine the concentration of estradiol in the sample.
Haftungsausschluss und Informationen zu In-Vitro-Forschungsprodukten
Bitte beachten Sie, dass alle Artikel und Produktinformationen, die auf BenchChem präsentiert werden, ausschließlich zu Informationszwecken bestimmt sind. Die auf BenchChem zum Kauf angebotenen Produkte sind speziell für In-vitro-Studien konzipiert, die außerhalb lebender Organismen durchgeführt werden. In-vitro-Studien, abgeleitet von dem lateinischen Begriff "in Glas", beinhalten Experimente, die in kontrollierten Laborumgebungen unter Verwendung von Zellen oder Geweben durchgeführt werden. Es ist wichtig zu beachten, dass diese Produkte nicht als Arzneimittel oder Medikamente eingestuft sind und keine Zulassung der FDA für die Vorbeugung, Behandlung oder Heilung von medizinischen Zuständen, Beschwerden oder Krankheiten erhalten haben. Wir müssen betonen, dass jede Form der körperlichen Einführung dieser Produkte in Menschen oder Tiere gesetzlich strikt untersagt ist. Es ist unerlässlich, sich an diese Richtlinien zu halten, um die Einhaltung rechtlicher und ethischer Standards in Forschung und Experiment zu gewährleisten.