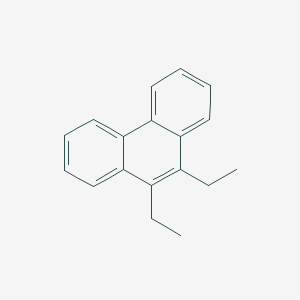
9,10-Diethylphenanthrene
Übersicht
Beschreibung
9,10-Diethylphenanthrene: is an organic compound with the molecular formula C18H18 . It belongs to the class of polycyclic aromatic hydrocarbons, specifically phenanthrenes, which are known for their three fused benzene rings arranged in a unique angular configuration. This compound is characterized by the presence of two ethyl groups attached to the 9th and 10th positions of the phenanthrene core. The molecular weight of this compound is 234.3355 g/mol .
Vorbereitungsmethoden
Synthetic Routes and Reaction Conditions: The synthesis of 9,10-Diethylphenanthrene can be achieved through various methods. One common approach involves the alkylation of phenanthrene using ethyl halides in the presence of a strong base such as sodium hydride or potassium tert-butoxide. The reaction typically occurs under reflux conditions in an inert solvent like tetrahydrofuran or dimethylformamide. The resulting product is then purified through recrystallization or column chromatography.
Industrial Production Methods: In an industrial setting, the production of this compound may involve the catalytic hydrogenation of 9,10-phenanthrenequinone followed by alkylation. This method ensures high yields and purity of the final product. The use of palladium or platinum catalysts in the hydrogenation step is common, and the reaction is carried out under controlled temperature and pressure conditions.
Analyse Chemischer Reaktionen
Types of Reactions: 9,10-Diethylphenanthrene undergoes various chemical reactions, including:
Oxidation: The compound can be oxidized using strong oxidizing agents such as potassium permanganate or chromium trioxide, leading to the formation of phenanthrenequinone derivatives.
Reduction: Reduction reactions can be performed using reducing agents like lithium aluminum hydride or sodium borohydride, resulting in the formation of dihydrophenanthrene derivatives.
Substitution: Electrophilic substitution reactions, such as nitration and halogenation, can occur at the aromatic ring positions.
Common Reagents and Conditions:
Oxidation: Potassium permanganate in acidic or neutral medium.
Reduction: Lithium aluminum hydride in dry ether.
Substitution: Fuming nitric acid in dichloromethane for nitration.
Major Products:
Oxidation: Phenanthrenequinone derivatives.
Reduction: Dihydrophenanthrene derivatives.
Substitution: Nitro and halogenated phenanthrene derivatives.
Wissenschaftliche Forschungsanwendungen
Chemistry: 9,10-Diethylphenanthrene is used as a building block in the synthesis of various organic compounds. Its unique structure makes it valuable in the study of aromaticity and electronic properties of polycyclic aromatic hydrocarbons.
Biology and Medicine: While specific biological applications of this compound are limited, its derivatives are often explored for their potential biological activities, including anticancer and antimicrobial properties.
Industry: In the industrial sector, this compound is used in the production of dyes, pigments, and organic semiconductors. Its stability and electronic properties make it suitable for use in organic light-emitting diodes (OLEDs) and other electronic devices .
Wirkmechanismus
The mechanism of action of 9,10-Diethylphenanthrene is primarily related to its electronic structure and aromaticity. The compound can interact with various molecular targets through π-π stacking interactions, hydrogen bonding, and van der Waals forces. These interactions can influence the electronic properties of the compound, making it useful in electronic and photonic applications. Additionally, the presence of ethyl groups can affect the compound’s reactivity and stability, further enhancing its utility in various chemical reactions .
Vergleich Mit ähnlichen Verbindungen
Phenanthrene: The parent compound of 9,10-Diethylphenanthrene, lacking the ethyl substituents.
9,10-Dimethylphenanthrene: Similar structure with methyl groups instead of ethyl groups.
9-Methyl-10-ethylphenanthrene: Contains one methyl and one ethyl group at the 9th and 10th positions, respectively.
Uniqueness: this compound is unique due to the presence of two ethyl groups, which can significantly influence its physical and chemical properties. The ethyl groups increase the compound’s hydrophobicity and can affect its solubility in various solvents. Additionally, the electronic effects of the ethyl groups can alter the compound’s reactivity compared to its methyl-substituted analogs .
Eigenschaften
IUPAC Name |
9,10-diethylphenanthrene | |
---|---|---|
Source | PubChem | |
URL | https://pubchem.ncbi.nlm.nih.gov | |
Description | Data deposited in or computed by PubChem | |
InChI |
InChI=1S/C18H18/c1-3-13-14(4-2)16-10-6-8-12-18(16)17-11-7-5-9-15(13)17/h5-12H,3-4H2,1-2H3 | |
Source | PubChem | |
URL | https://pubchem.ncbi.nlm.nih.gov | |
Description | Data deposited in or computed by PubChem | |
InChI Key |
JURXVMQHSBALPL-UHFFFAOYSA-N | |
Source | PubChem | |
URL | https://pubchem.ncbi.nlm.nih.gov | |
Description | Data deposited in or computed by PubChem | |
Canonical SMILES |
CCC1=C(C2=CC=CC=C2C3=CC=CC=C31)CC | |
Source | PubChem | |
URL | https://pubchem.ncbi.nlm.nih.gov | |
Description | Data deposited in or computed by PubChem | |
Molecular Formula |
C18H18 | |
Source | PubChem | |
URL | https://pubchem.ncbi.nlm.nih.gov | |
Description | Data deposited in or computed by PubChem | |
DSSTOX Substance ID |
DTXSID90423820 | |
Record name | 9,10-diethylphenanthrene | |
Source | EPA DSSTox | |
URL | https://comptox.epa.gov/dashboard/DTXSID90423820 | |
Description | DSSTox provides a high quality public chemistry resource for supporting improved predictive toxicology. | |
Molecular Weight |
234.3 g/mol | |
Source | PubChem | |
URL | https://pubchem.ncbi.nlm.nih.gov | |
Description | Data deposited in or computed by PubChem | |
CAS No. |
15810-14-7 | |
Record name | 9,10-diethylphenanthrene | |
Source | EPA DSSTox | |
URL | https://comptox.epa.gov/dashboard/DTXSID90423820 | |
Description | DSSTox provides a high quality public chemistry resource for supporting improved predictive toxicology. | |
Retrosynthesis Analysis
AI-Powered Synthesis Planning: Our tool employs the Template_relevance Pistachio, Template_relevance Bkms_metabolic, Template_relevance Pistachio_ringbreaker, Template_relevance Reaxys, Template_relevance Reaxys_biocatalysis model, leveraging a vast database of chemical reactions to predict feasible synthetic routes.
One-Step Synthesis Focus: Specifically designed for one-step synthesis, it provides concise and direct routes for your target compounds, streamlining the synthesis process.
Accurate Predictions: Utilizing the extensive PISTACHIO, BKMS_METABOLIC, PISTACHIO_RINGBREAKER, REAXYS, REAXYS_BIOCATALYSIS database, our tool offers high-accuracy predictions, reflecting the latest in chemical research and data.
Strategy Settings
Precursor scoring | Relevance Heuristic |
---|---|
Min. plausibility | 0.01 |
Model | Template_relevance |
Template Set | Pistachio/Bkms_metabolic/Pistachio_ringbreaker/Reaxys/Reaxys_biocatalysis |
Top-N result to add to graph | 6 |
Feasible Synthetic Routes
Haftungsausschluss und Informationen zu In-Vitro-Forschungsprodukten
Bitte beachten Sie, dass alle Artikel und Produktinformationen, die auf BenchChem präsentiert werden, ausschließlich zu Informationszwecken bestimmt sind. Die auf BenchChem zum Kauf angebotenen Produkte sind speziell für In-vitro-Studien konzipiert, die außerhalb lebender Organismen durchgeführt werden. In-vitro-Studien, abgeleitet von dem lateinischen Begriff "in Glas", beinhalten Experimente, die in kontrollierten Laborumgebungen unter Verwendung von Zellen oder Geweben durchgeführt werden. Es ist wichtig zu beachten, dass diese Produkte nicht als Arzneimittel oder Medikamente eingestuft sind und keine Zulassung der FDA für die Vorbeugung, Behandlung oder Heilung von medizinischen Zuständen, Beschwerden oder Krankheiten erhalten haben. Wir müssen betonen, dass jede Form der körperlichen Einführung dieser Produkte in Menschen oder Tiere gesetzlich strikt untersagt ist. Es ist unerlässlich, sich an diese Richtlinien zu halten, um die Einhaltung rechtlicher und ethischer Standards in Forschung und Experiment zu gewährleisten.