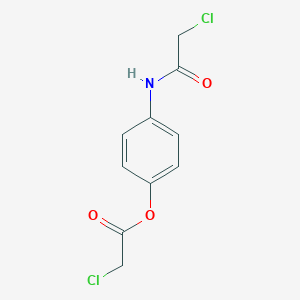
p-Chloroacetoxy-2-chloroacetanilide
Übersicht
Beschreibung
P-Chloroacetoxy-2-chloroacetanilide is a chemical compound with the molecular formula C10H9Cl2NO3 . It is also known by other names such as 2-Chloro-4’-chloroacetoxyacetanilide, 4-[(2-Chloroacetyl)amino]phenyl chloroacetate, and 2-chloroacetic acid [4-[(2-chloroacetyl)amino]phenyl] ester .
Chemical Reactions Analysis
A study on the degradation mechanism of chloroacetanilide herbicides in the presence of four different nucleophiles, namely: Br−, I−, HS−, and S2O32−, was theoretically evaluated . The results suggest that the best nucleophiles are those where a sulfur atom performs the nucleophilic attack, whereas the other species are less reactive .Wissenschaftliche Forschungsanwendungen
Degradation of Chloroacetanilide Herbicides
The compound is used in the degradation of chloroacetanilide herbicides in natural waters using UV activated hydrogen peroxide, persulfate and peroxymonosulfate processes . The UV/S 2 O 82− and UV/H 2 O 2 processes proved to be most effective under acidic conditions (pH 5), while the UV/HSO 5− process showed the highest degradation efficacy under basic conditions (pH > 8) .
2. Removal of Chloroacetanilide Herbicides from Water Chloroacetic acid 4-[(chloroacetyl)amino]phenyl ester is used in the removal of chloroacetanilide herbicides from water using heterogeneous photocatalysis with TiO2/UV-A . The maximum removal efficiency for alachlor, acetochlor, and metolachlor was 97.5%, 93.1%, and 98.2%, respectively .
Residue and Soil Dissipation Kinetics
The compound is used in the study of residue and soil dissipation kinetics of chloroacetanilide . The enzyme acid phosphatase showed a reverse trend to those of enzyme dehydrogenase and urease .
Production of Thickening Agents
Chloroacetic acid, a related compound, is used to prepare the thickening agent carboxymethyl cellulose and carboxymethyl starch .
Production of Phenoxy Herbicides
Chloroacetic acid is also used in the production of phenoxy herbicides by etherification with chlorophenols .
Antibacterial Activity
Chloroacetic acid immobilized on chitosan (CS) coated iron oxide decorated by silver nanoparticles (Fe 3 O 4 @CS@Ag@CH 2 COOH) was synthesized as a biocompatible magnetic material . The Fe 3 O 4 @CS@Ag@CH 2 COOH nanocomposite presented good antibacterial performance toward gram-negative Escherichia coli and gram-positive S. aureus .
Synthesis of Hexahydroquinoline-3-Carboxamides
The compound is used as an efficient catalyst for the synthesis of hexahydroquinoline-3-carboxamide derivatives .
Organic Intermediates
Chloroacetic acid and its derivatives, such as salts, esters, anhydrides, acyl chlorides, amides, and hydrazides, are widely used as synthetic organic intermediates . Industrial applications of chloroacetic acid include reactions with inorganic and organic bases, which yield salts .
Safety and Hazards
The safety data sheet for 2’-Chloroacetanilide, a related compound, indicates that it causes skin irritation, serious eye irritation, and may cause respiratory irritation . It is recommended to avoid breathing dust/fume/gas/mist/vapors/spray and to use only outdoors or in a well-ventilated area . In case of contact with skin or eyes, it is advised to wash with plenty of soap and water and get medical attention .
Eigenschaften
IUPAC Name |
[4-[(2-chloroacetyl)amino]phenyl] 2-chloroacetate | |
---|---|---|
Source | PubChem | |
URL | https://pubchem.ncbi.nlm.nih.gov | |
Description | Data deposited in or computed by PubChem | |
InChI |
InChI=1S/C10H9Cl2NO3/c11-5-9(14)13-7-1-3-8(4-2-7)16-10(15)6-12/h1-4H,5-6H2,(H,13,14) | |
Source | PubChem | |
URL | https://pubchem.ncbi.nlm.nih.gov | |
Description | Data deposited in or computed by PubChem | |
InChI Key |
NCHAQEGVLDMYGR-UHFFFAOYSA-N | |
Source | PubChem | |
URL | https://pubchem.ncbi.nlm.nih.gov | |
Description | Data deposited in or computed by PubChem | |
Canonical SMILES |
C1=CC(=CC=C1NC(=O)CCl)OC(=O)CCl | |
Source | PubChem | |
URL | https://pubchem.ncbi.nlm.nih.gov | |
Description | Data deposited in or computed by PubChem | |
Molecular Formula |
C10H9Cl2NO3 | |
Source | PubChem | |
URL | https://pubchem.ncbi.nlm.nih.gov | |
Description | Data deposited in or computed by PubChem | |
DSSTOX Substance ID |
DTXSID00170131 | |
Record name | Acetanilide, 2-chloro-4'-hydroxy-, chloroacetate (ester) | |
Source | EPA DSSTox | |
URL | https://comptox.epa.gov/dashboard/DTXSID00170131 | |
Description | DSSTox provides a high quality public chemistry resource for supporting improved predictive toxicology. | |
Molecular Weight |
262.09 g/mol | |
Source | PubChem | |
URL | https://pubchem.ncbi.nlm.nih.gov | |
Description | Data deposited in or computed by PubChem | |
CAS RN |
17641-10-0 | |
Record name | Acetanilide, 2-chloro-4'-hydroxy-, chloroacetate (ester) | |
Source | ChemIDplus | |
URL | https://pubchem.ncbi.nlm.nih.gov/substance/?source=chemidplus&sourceid=0017641100 | |
Description | ChemIDplus is a free, web search system that provides access to the structure and nomenclature authority files used for the identification of chemical substances cited in National Library of Medicine (NLM) databases, including the TOXNET system. | |
Record name | Acetanilide, 2-chloro-4'-hydroxy-, chloroacetate (ester) | |
Source | EPA DSSTox | |
URL | https://comptox.epa.gov/dashboard/DTXSID00170131 | |
Description | DSSTox provides a high quality public chemistry resource for supporting improved predictive toxicology. | |
Retrosynthesis Analysis
AI-Powered Synthesis Planning: Our tool employs the Template_relevance Pistachio, Template_relevance Bkms_metabolic, Template_relevance Pistachio_ringbreaker, Template_relevance Reaxys, Template_relevance Reaxys_biocatalysis model, leveraging a vast database of chemical reactions to predict feasible synthetic routes.
One-Step Synthesis Focus: Specifically designed for one-step synthesis, it provides concise and direct routes for your target compounds, streamlining the synthesis process.
Accurate Predictions: Utilizing the extensive PISTACHIO, BKMS_METABOLIC, PISTACHIO_RINGBREAKER, REAXYS, REAXYS_BIOCATALYSIS database, our tool offers high-accuracy predictions, reflecting the latest in chemical research and data.
Strategy Settings
Precursor scoring | Relevance Heuristic |
---|---|
Min. plausibility | 0.01 |
Model | Template_relevance |
Template Set | Pistachio/Bkms_metabolic/Pistachio_ringbreaker/Reaxys/Reaxys_biocatalysis |
Top-N result to add to graph | 6 |
Feasible Synthetic Routes
Haftungsausschluss und Informationen zu In-Vitro-Forschungsprodukten
Bitte beachten Sie, dass alle Artikel und Produktinformationen, die auf BenchChem präsentiert werden, ausschließlich zu Informationszwecken bestimmt sind. Die auf BenchChem zum Kauf angebotenen Produkte sind speziell für In-vitro-Studien konzipiert, die außerhalb lebender Organismen durchgeführt werden. In-vitro-Studien, abgeleitet von dem lateinischen Begriff "in Glas", beinhalten Experimente, die in kontrollierten Laborumgebungen unter Verwendung von Zellen oder Geweben durchgeführt werden. Es ist wichtig zu beachten, dass diese Produkte nicht als Arzneimittel oder Medikamente eingestuft sind und keine Zulassung der FDA für die Vorbeugung, Behandlung oder Heilung von medizinischen Zuständen, Beschwerden oder Krankheiten erhalten haben. Wir müssen betonen, dass jede Form der körperlichen Einführung dieser Produkte in Menschen oder Tiere gesetzlich strikt untersagt ist. Es ist unerlässlich, sich an diese Richtlinien zu halten, um die Einhaltung rechtlicher und ethischer Standards in Forschung und Experiment zu gewährleisten.