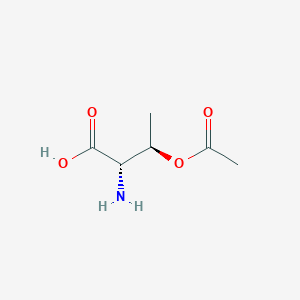
(2S,3R)-3-Acetoxy-2-aminobutansäure
Übersicht
Beschreibung
O-Acetyl-L-Threonine is a useful research compound. Its molecular formula is C6H11NO4 and its molecular weight is 161.16 g/mol. The purity is usually 95%.
BenchChem offers high-quality O-Acetyl-L-Threonine suitable for many research applications. Different packaging options are available to accommodate customers' requirements. Please inquire for more information about O-Acetyl-L-Threonine including the price, delivery time, and more detailed information at info@benchchem.com.
Wissenschaftliche Forschungsanwendungen
Hydroxylierung von Pipecolsäure
Diese Verbindung spielt eine Rolle bei der Hydroxylierung von Pipecolsäure. Fe(II)/α-Ketoglutarsäure (Fe(II)/2-OG)-abhängige Dioxygenasen können diesen Prozess katalysieren .
Produktion von Homoserinlacton, Methionin, 1,4-Butandiol und 1,3-Propandiol
“(2S,3R)-3-Acetoxy-2-aminobutansäure” ist ein potenziell wichtiges Plattformmetabolit für die Produktion von Homoserinlacton, Methionin, 1,4-Butandiol und 1,3-Propandiol .
Produktion von O-Acetyl-L-Homoserin
Diese Verbindung wurde in der Stoffwechseltechnik und im Pfadaufbau für die Produktion von O-Acetyl-L-Homoserin in Escherichia coli verwendet . Der gentechnisch veränderte Stamm produzierte 24,33 g/L OAH mit einer Ausbeute von 0,23 g/g Glucose in der Fed-Batch-Fermentation .
Produktion von hochwertigen Verbindungen
O-Acetyl-L-Homoserin (OAH) ist ein potenzieller Plattformstoff für die Produktion von hochwertigen Verbindungen wie L-Methionin . Die Produktion von OAH ist für die industrielle Produktion von L-Methionin sehr wichtig .
Anwendung in AC-Mikronetzen
Obwohl es nicht direkt mit der Verbindung selbst zusammenhängt, kann die Abkürzung „AC“ in „H-THR(AC)-OH“ auch für Wechselstrom stehen, der in AC-Mikronetzen verwendet wird . Dies ist eher ein sprachlicher Zufall als eine wissenschaftliche Anwendung, aber es ist dennoch eine interessante Verbindung.
Wirkmechanismus
Target of Action
Similar compounds have been known to interact with various enzymes and receptors in the body .
Mode of Action
It’s plausible that it interacts with its targets in a manner similar to other amino acids, possibly acting as a substrate, inhibitor, or modulator .
Biochemical Pathways
It’s reasonable to assume that it may be involved in amino acid metabolism or other related pathways .
Pharmacokinetics
As with many other compounds, factors such as solubility, stability, and molecular size would likely influence its bioavailability .
Result of Action
Given its structure, it might influence protein synthesis or other cellular processes involving amino acids .
Action Environment
Environmental factors such as pH, temperature, and the presence of other molecules could potentially influence the action, efficacy, and stability of (2S,3R)-3-Acetoxy-2-aminobutanoic acid .
Biologische Aktivität
O-Acetyl-L-Threonine (O-Ac-Thr) is a derivative of the amino acid threonine, which plays a crucial role in various biological processes. This compound has garnered attention due to its potential applications in metabolic engineering and its implications in nutrition and health. This article explores the biological activity of O-Acetyl-L-Threonine, focusing on its physiological roles, metabolic pathways, and research findings.
O-Acetyl-L-Threonine is a modified form of threonine, characterized by the addition of an acetyl group. Its chemical structure can be represented as follows:
- Molecular Formula : C₆H₁₁NO₄
- CAS Number : 16718064
Physiological Functions of Threonine
Threonine is an essential amino acid that serves multiple functions in animal physiology:
- Protein Synthesis : Threonine is a precursor for the synthesis of proteins, particularly mucins, which are critical for gut health .
- Metabolic Regulation : It influences metabolic pathways related to energy metabolism and protein biosynthesis. Threonine supplementation has been shown to enhance growth performance in livestock by improving protein synthesis in skeletal muscles .
- Immune Function : Threonine plays a role in modulating the intestinal immune system through signaling pathways such as MAPK and TOR .
Metabolism and Pathways
The metabolism of O-Acetyl-L-Threonine involves several key enzymatic reactions. It can be synthesized from threonine through acetylation processes, which may involve enzymes such as threonine aldolase. This enzyme catalyzes the reversible cleavage of threonine to produce glycine and aldehydes, highlighting the potential biotechnological applications of O-Ac-Thr in synthesizing various compounds .
Case Studies
-
Nutritional Supplementation in Animals :
- A study demonstrated that dietary threonine supplementation improved intestinal health and protein synthesis in swine. The findings indicated that about 40-60% of dietary threonine was utilized for mucosal protein synthesis, emphasizing its importance in gut health .
- Another investigation into the effects of O-Ac-Thr on embryonic stem cells (ESCs) revealed that it serves as a metabolic fuel promoting self-renewal and differentiation processes. Threonine's role in ESCs suggests its potential therapeutic applications in regenerative medicine .
-
Metabolic Engineering :
- Research focused on the production of O-Acetyl-L-Homoserine (a related compound) using engineered strains of E. coli highlighted the significance of metabolic pathways involving threonine derivatives. The engineered strains achieved high yields of O-Ac-Homoserine, showcasing the potential for industrial applications .
Research Findings
Recent studies have elucidated various aspects of O-Acetyl-L-Threonine's biological activity:
- Cell Growth and Proliferation : Threonine has been linked to cell growth regulation through its involvement in signaling pathways that control cell cycle progression. For instance, it activates mTOR signaling, which is crucial for cell proliferation .
- Impact on Lipid Metabolism : Threonine supplementation has been associated with protective effects against lipid metabolic disorders by regulating lipogenesis signaling pathways .
Eigenschaften
IUPAC Name |
(2S,3R)-3-acetyloxy-2-aminobutanoic acid | |
---|---|---|
Source | PubChem | |
URL | https://pubchem.ncbi.nlm.nih.gov | |
Description | Data deposited in or computed by PubChem | |
InChI |
InChI=1S/C6H11NO4/c1-3(11-4(2)8)5(7)6(9)10/h3,5H,7H2,1-2H3,(H,9,10)/t3-,5+/m1/s1 | |
Source | PubChem | |
URL | https://pubchem.ncbi.nlm.nih.gov | |
Description | Data deposited in or computed by PubChem | |
InChI Key |
GOVSRIMJZNIFHS-WUJLRWPWSA-N | |
Source | PubChem | |
URL | https://pubchem.ncbi.nlm.nih.gov | |
Description | Data deposited in or computed by PubChem | |
Canonical SMILES |
CC(C(C(=O)O)N)OC(=O)C | |
Source | PubChem | |
URL | https://pubchem.ncbi.nlm.nih.gov | |
Description | Data deposited in or computed by PubChem | |
Isomeric SMILES |
C[C@H]([C@@H](C(=O)O)N)OC(=O)C | |
Source | PubChem | |
URL | https://pubchem.ncbi.nlm.nih.gov | |
Description | Data deposited in or computed by PubChem | |
Molecular Formula |
C6H11NO4 | |
Source | PubChem | |
URL | https://pubchem.ncbi.nlm.nih.gov | |
Description | Data deposited in or computed by PubChem | |
DSSTOX Substance ID |
DTXSID40587325 | |
Record name | O-Acetyl-L-threonine | |
Source | EPA DSSTox | |
URL | https://comptox.epa.gov/dashboard/DTXSID40587325 | |
Description | DSSTox provides a high quality public chemistry resource for supporting improved predictive toxicology. | |
Molecular Weight |
161.16 g/mol | |
Source | PubChem | |
URL | https://pubchem.ncbi.nlm.nih.gov | |
Description | Data deposited in or computed by PubChem | |
CAS No. |
17012-42-9 | |
Record name | O-Acetylthreonine | |
Source | ChemIDplus | |
URL | https://pubchem.ncbi.nlm.nih.gov/substance/?source=chemidplus&sourceid=0017012429 | |
Description | ChemIDplus is a free, web search system that provides access to the structure and nomenclature authority files used for the identification of chemical substances cited in National Library of Medicine (NLM) databases, including the TOXNET system. | |
Record name | O-Acetyl-L-threonine | |
Source | EPA DSSTox | |
URL | https://comptox.epa.gov/dashboard/DTXSID40587325 | |
Description | DSSTox provides a high quality public chemistry resource for supporting improved predictive toxicology. | |
Record name | O-ACETYLTHREONINE | |
Source | FDA Global Substance Registration System (GSRS) | |
URL | https://gsrs.ncats.nih.gov/ginas/app/beta/substances/I5ABK2S28W | |
Description | The FDA Global Substance Registration System (GSRS) enables the efficient and accurate exchange of information on what substances are in regulated products. Instead of relying on names, which vary across regulatory domains, countries, and regions, the GSRS knowledge base makes it possible for substances to be defined by standardized, scientific descriptions. | |
Explanation | Unless otherwise noted, the contents of the FDA website (www.fda.gov), both text and graphics, are not copyrighted. They are in the public domain and may be republished, reprinted and otherwise used freely by anyone without the need to obtain permission from FDA. Credit to the U.S. Food and Drug Administration as the source is appreciated but not required. | |
Retrosynthesis Analysis
AI-Powered Synthesis Planning: Our tool employs the Template_relevance Pistachio, Template_relevance Bkms_metabolic, Template_relevance Pistachio_ringbreaker, Template_relevance Reaxys, Template_relevance Reaxys_biocatalysis model, leveraging a vast database of chemical reactions to predict feasible synthetic routes.
One-Step Synthesis Focus: Specifically designed for one-step synthesis, it provides concise and direct routes for your target compounds, streamlining the synthesis process.
Accurate Predictions: Utilizing the extensive PISTACHIO, BKMS_METABOLIC, PISTACHIO_RINGBREAKER, REAXYS, REAXYS_BIOCATALYSIS database, our tool offers high-accuracy predictions, reflecting the latest in chemical research and data.
Strategy Settings
Precursor scoring | Relevance Heuristic |
---|---|
Min. plausibility | 0.01 |
Model | Template_relevance |
Template Set | Pistachio/Bkms_metabolic/Pistachio_ringbreaker/Reaxys/Reaxys_biocatalysis |
Top-N result to add to graph | 6 |
Feasible Synthetic Routes
Q1: What is the biological significance of O-Acetyl-L-Serine (OAS) and how does it relate to O-Acetyl-L-Threonine?
A: O-Acetyl-L-Serine (OAS) plays a crucial role as an extracellular signaling molecule in bacteria like Providencia stuartii and Escherichia coli []. It functions by activating specific genes, some of which are regulated by CysB, a transcriptional activator requiring N-acetyl-l-serine (NAS) as a coactivator []. Interestingly, research has shown that only OAS, and not O-Acetyl-L-Threonine or N-acetyl-l-threonine, could activate the cma37::lacZ fusion, indicating a high level of specificity []. This suggests a potential interaction between the OAS signaling pathway and threonine metabolism, warranting further investigation.
Q2: How does the structure of poly-O-acetyl-L-threonine relate to its optical properties?
A: Poly-O-acetyl-L-threonine adopts a β-conformation in various solvents and in the solid state []. This conformation contributes to its unique optical rotatory properties. For example, in a 2-chloroethanol–dichloromethane mixture, the β-form of poly-O-acetyl-L-threonine exhibits a Cotton effect at 230 mμ []. This specific optical behavior is attributed to the chiral centers within the poly-O-acetyl-L-threonine structure, highlighting the importance of stereochemistry in its physicochemical properties.
Q3: Can O-Acetyl-L-Threonine be synthesized from L-threonine, and if so, what is the significance of this reaction?
A: Yes, O-Acetyl-L-Threonine can be incorporated into peptides using L-threonine as a starting point []. This synthesis is particularly relevant in studying structure-activity relationships of biologically active peptides like bradykinin []. By substituting the native L-serine at the 6th position with O-Acetyl-L-Threonine, researchers can investigate how this modification affects the peptide's interaction with its target and its overall biological activity []. This approach is valuable for understanding the impact of subtle structural changes on peptide function.
Q4: Beyond its use in peptide synthesis, are there other applications for O-Acetyl-L-Threonine derivatives?
A: Yes, derivatives of O-Acetyl-L-Threonine, like N-Tosyl-O-acetyl-L-threonine anilide, have shown potential in organic synthesis []. This compound can be further modified through reactions with acetic acid or formaldehyde in the presence of boron trifluoride catalyst []. These reactions lead to the formation of 1,3-oxazolidine and 1,3-oxazine derivatives, which are valuable heterocyclic compounds with applications in medicinal chemistry and materials science []. This highlights the versatility of O-Acetyl-L-Threonine derivatives as building blocks for more complex molecules.
Haftungsausschluss und Informationen zu In-Vitro-Forschungsprodukten
Bitte beachten Sie, dass alle Artikel und Produktinformationen, die auf BenchChem präsentiert werden, ausschließlich zu Informationszwecken bestimmt sind. Die auf BenchChem zum Kauf angebotenen Produkte sind speziell für In-vitro-Studien konzipiert, die außerhalb lebender Organismen durchgeführt werden. In-vitro-Studien, abgeleitet von dem lateinischen Begriff "in Glas", beinhalten Experimente, die in kontrollierten Laborumgebungen unter Verwendung von Zellen oder Geweben durchgeführt werden. Es ist wichtig zu beachten, dass diese Produkte nicht als Arzneimittel oder Medikamente eingestuft sind und keine Zulassung der FDA für die Vorbeugung, Behandlung oder Heilung von medizinischen Zuständen, Beschwerden oder Krankheiten erhalten haben. Wir müssen betonen, dass jede Form der körperlichen Einführung dieser Produkte in Menschen oder Tiere gesetzlich strikt untersagt ist. Es ist unerlässlich, sich an diese Richtlinien zu halten, um die Einhaltung rechtlicher und ethischer Standards in Forschung und Experiment zu gewährleisten.