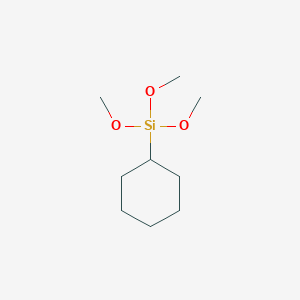
Cyclohexyltrimethoxysilane
Übersicht
Beschreibung
Cyclohexyltrimethoxysilane is an organosilicon compound with the chemical formula C₉H₂₀O₃Si. It is a colorless to almost colorless liquid that is used in various industrial and scientific applications. This compound is known for its ability to act as a coupling agent, which helps in bonding organic materials to inorganic surfaces.
Vorbereitungsmethoden
Cyclohexyltrimethoxysilane can be synthesized through several methods. One common method involves the reaction of cyclohexyltrichlorosilane with methanol in the presence of a base such as pyridine. The reaction is typically carried out under an inert atmosphere to prevent moisture from interfering with the process . The general reaction is as follows:
[ \text{Cyclohexyltrichlorosilane} + 3 \text{Methanol} \rightarrow \text{this compound} + 3 \text{Hydrochloric Acid} ]
Industrial production methods often involve the hydrolysis and condensation of alkoxysilanes under controlled conditions. This process can be catalyzed by acids or bases and is carried out under a nitrogen atmosphere to ensure the stability of the product .
Analyse Chemischer Reaktionen
Cyclohexyltrimethoxysilane undergoes several types of chemical reactions, including:
Hydrolysis and Condensation: In the presence of water, this compound hydrolyzes to form silanols, which can further condense to form siloxane bonds.
Substitution Reactions: The methoxy groups in this compound can be substituted with other nucleophiles, such as amines or alcohols, under appropriate conditions.
Common reagents used in these reactions include water, methanol, and various acids or bases. The major products formed from these reactions are silanols and siloxanes.
Wissenschaftliche Forschungsanwendungen
Chemical Synthesis
Polysilsesquioxanes Production
Cyclohexyltrimethoxysilane is utilized as a precursor for synthesizing polysilsesquioxanes, which are important due to their thermal and mechanical stability. These materials are synthesized through sol-gel processes involving hydrolysis and condensation reactions of alkoxysilanes, including this compound. The resulting polysilsesquioxanes find applications in coatings and advanced materials due to their robust properties .
Oligosilsesquioxanes Formation
This compound is also used in the preparation of oligosilsesquioxanes, which serve as organic-inorganic fillers. The incorporation of these fillers into polymers, such as polymethyl methacrylate (PMMA), enhances thermal and mechanical properties through intermolecular interactions.
Coatings and Adhesives
Coupling Agent
In the coatings and adhesives industry, this compound acts as a coupling agent that improves adhesion between organic materials and inorganic substrates. Its ability to form stable bonds enhances the performance of coatings and adhesives, making them more effective for various applications .
Cross-Linking Agent
This compound is also employed as a cross-linking agent in epoxy and polyurethane resins. The cross-linking process significantly improves the durability and chemical resistance of these materials, which are widely used in construction and automotive industries .
Construction Industry
Water Repellent Agent
this compound is used as a water repellent agent for concrete and other building materials. It enhances the hydrophobic properties of surfaces, reducing water absorption and improving longevity . This application is particularly beneficial for structures exposed to harsh environmental conditions.
Concrete Additive
Research has shown that incorporating this compound into concrete formulations can enhance compressive strength and modify setting times, making it suitable for various structural applications .
Pharmaceuticals and Biotechnology
Drug Delivery Systems
In the pharmaceutical field, this compound is utilized in developing drug delivery systems. Its ability to bond with various substrates allows for the creation of stable formulations that can improve bioavailability and efficacy of therapeutic agents .
Surface Modification
This compound can modify the surfaces of biological materials, enhancing their compatibility with inorganic substrates. This property is crucial in biomedical applications where integration with biological tissues is required.
Textile Industry
This compound serves as a sizing agent in textiles, improving water repellency and durability of fabrics. This application is critical in producing high-performance textiles that require enhanced resistance to moisture .
Electronics Industry
In electronics, this compound is used as a surface modifier for electronic components. It enhances adhesion properties and provides water resistance, which are essential for ensuring the reliability and longevity of electronic devices .
Summary Table of Applications
Application Area | Specific Use | Benefits |
---|---|---|
Chemical Synthesis | Precursor for polysilsesquioxanes | Thermal/mechanical stability |
Formation of oligosilsesquioxanes | Enhanced polymer properties | |
Coatings & Adhesives | Coupling agent | Improved adhesion |
Cross-linking agent | Increased durability | |
Construction | Water repellent agent | Reduced water absorption |
Concrete additive | Enhanced compressive strength | |
Pharmaceuticals | Drug delivery systems | Improved bioavailability |
Surface modification | Better compatibility | |
Textile | Sizing agent | Improved water repellency |
Electronics | Surface modifier | Enhanced adhesion/water resistance |
Case Studies
-
Polymethyl Methacrylate Enhancement
A study demonstrated that incorporating oligosilsesquioxanes derived from this compound into PMMA significantly improved its thermal stability and mechanical properties through effective intermolecular interactions during melt blending processes. -
Concrete Performance Improvement
Research indicated that adding this compound to concrete mixtures resulted in a measurable increase in compressive strength while also modifying the setting time, making it advantageous for large-scale construction projects.
Wirkmechanismus
The primary mechanism by which cyclohexyltrimethoxysilane exerts its effects is through the formation of siloxane bonds. When it hydrolyzes, it forms silanols, which can then condense to form siloxane bonds. These bonds are responsible for the compound’s ability to act as a coupling agent, enhancing the adhesion between organic and inorganic materials .
Vergleich Mit ähnlichen Verbindungen
Cyclohexyltrimethoxysilane can be compared with other alkoxysilanes such as methyltrimethoxysilane and phenyltrimethoxysilane. While all these compounds can form siloxane bonds, this compound is unique due to its cyclohexyl group, which provides different steric and electronic properties. This makes it particularly useful in applications where a more rigid or bulky substituent is required .
Similar Compounds
- Methyltrimethoxysilane
- Phenyltrimethoxysilane
- Ethyltrimethoxysilane
This compound stands out due to its unique combination of properties, making it a versatile compound in various fields of research and industry.
Biologische Aktivität
Cyclohexyltrimethoxysilane (CHTMS) is a silane compound that has garnered attention for its diverse applications in various fields, including chemistry, biology, and medicine. This article explores its biological activity, focusing on its mechanisms of action, applications in drug delivery systems, and its role in modifying biological materials.
This compound is characterized by its ability to undergo hydrolysis and condensation reactions, leading to the formation of siloxane networks. The primary mechanism involves the reaction of CHTMS with water, resulting in the release of methanol and the formation of silanol groups, which can further react to create siloxane bonds. This process is crucial for its applications in surface modification and material synthesis.
Hydrolysis-Condensation Reaction
The hydrolysis-condensation reaction can be summarized as follows:
This reaction is influenced by environmental factors such as pH and temperature, affecting the rate of siloxane bond formation and the properties of the resultant materials .
Applications in Drug Delivery Systems
One significant application of CHTMS is in drug delivery systems, particularly for anticancer drugs. A study demonstrated that CHTMS-modified mesoporous silica could encapsulate 5-fluorouracil (5-FU), a common chemotherapy agent. The encapsulation efficiency and drug release profiles were significantly affected by the presence of cyclohexyl groups, which enhanced drug interaction with the silica matrix .
Table 1: Encapsulation Efficiency of 5-FU in Modified Silica
Sample | Encapsulation Efficiency (%) | Loading Capacity (mg 5-FU/g) |
---|---|---|
Unmodified SBA-15 | 14.23 | 142.3 |
Cyclohexyl-SBA-15 | 14.6 | 146 |
Amino-SBA-15 | 18.425 | 184.25 |
The study concluded that the hydrophobic nature of cyclohexyl groups contributed to a slower release rate of the drug compared to amino-modified silica .
Surface Modification for Biocompatibility
CHTMS is also utilized to enhance the biocompatibility of inorganic materials. By modifying surfaces with CHTMS, researchers have improved the compatibility of implants with biological tissues. This modification facilitates better integration with surrounding tissues and reduces adverse reactions.
Case Study: Dental Adhesives
In dental applications, CHTMS has been incorporated into methacrylate-based adhesives. A study showed that introducing CHTMS improved mechanical properties and reduced leaching of methacrylate monomers in wet environments. The adhesive formulations demonstrated enhanced durability and bonding strength under oral conditions .
Safety and Toxicology
While CHTMS has beneficial applications, safety considerations are paramount. It is classified as a combustible liquid that can cause skin and eye irritation. Proper handling and safety measures should be implemented when working with this compound.
Eigenschaften
IUPAC Name |
cyclohexyl(trimethoxy)silane | |
---|---|---|
Source | PubChem | |
URL | https://pubchem.ncbi.nlm.nih.gov | |
Description | Data deposited in or computed by PubChem | |
InChI |
InChI=1S/C9H20O3Si/c1-10-13(11-2,12-3)9-7-5-4-6-8-9/h9H,4-8H2,1-3H3 | |
Source | PubChem | |
URL | https://pubchem.ncbi.nlm.nih.gov | |
Description | Data deposited in or computed by PubChem | |
InChI Key |
MEWFSXFFGFDHGV-UHFFFAOYSA-N | |
Source | PubChem | |
URL | https://pubchem.ncbi.nlm.nih.gov | |
Description | Data deposited in or computed by PubChem | |
Canonical SMILES |
CO[Si](C1CCCCC1)(OC)OC | |
Source | PubChem | |
URL | https://pubchem.ncbi.nlm.nih.gov | |
Description | Data deposited in or computed by PubChem | |
Molecular Formula |
C9H20O3Si | |
Source | PubChem | |
URL | https://pubchem.ncbi.nlm.nih.gov | |
Description | Data deposited in or computed by PubChem | |
DSSTOX Substance ID |
DTXSID20451277 | |
Record name | Cyclohexyltrimethoxysilane | |
Source | EPA DSSTox | |
URL | https://comptox.epa.gov/dashboard/DTXSID20451277 | |
Description | DSSTox provides a high quality public chemistry resource for supporting improved predictive toxicology. | |
Molecular Weight |
204.34 g/mol | |
Source | PubChem | |
URL | https://pubchem.ncbi.nlm.nih.gov | |
Description | Data deposited in or computed by PubChem | |
CAS No. |
17865-54-2 | |
Record name | Cyclohexyltrimethoxysilane | |
Source | CAS Common Chemistry | |
URL | https://commonchemistry.cas.org/detail?cas_rn=17865-54-2 | |
Description | CAS Common Chemistry is an open community resource for accessing chemical information. Nearly 500,000 chemical substances from CAS REGISTRY cover areas of community interest, including common and frequently regulated chemicals, and those relevant to high school and undergraduate chemistry classes. This chemical information, curated by our expert scientists, is provided in alignment with our mission as a division of the American Chemical Society. | |
Explanation | The data from CAS Common Chemistry is provided under a CC-BY-NC 4.0 license, unless otherwise stated. | |
Record name | Cyclohexyltrimethoxysilane | |
Source | EPA DSSTox | |
URL | https://comptox.epa.gov/dashboard/DTXSID20451277 | |
Description | DSSTox provides a high quality public chemistry resource for supporting improved predictive toxicology. | |
Retrosynthesis Analysis
AI-Powered Synthesis Planning: Our tool employs the Template_relevance Pistachio, Template_relevance Bkms_metabolic, Template_relevance Pistachio_ringbreaker, Template_relevance Reaxys, Template_relevance Reaxys_biocatalysis model, leveraging a vast database of chemical reactions to predict feasible synthetic routes.
One-Step Synthesis Focus: Specifically designed for one-step synthesis, it provides concise and direct routes for your target compounds, streamlining the synthesis process.
Accurate Predictions: Utilizing the extensive PISTACHIO, BKMS_METABOLIC, PISTACHIO_RINGBREAKER, REAXYS, REAXYS_BIOCATALYSIS database, our tool offers high-accuracy predictions, reflecting the latest in chemical research and data.
Strategy Settings
Precursor scoring | Relevance Heuristic |
---|---|
Min. plausibility | 0.01 |
Model | Template_relevance |
Template Set | Pistachio/Bkms_metabolic/Pistachio_ringbreaker/Reaxys/Reaxys_biocatalysis |
Top-N result to add to graph | 6 |
Feasible Synthetic Routes
Q1: How does Cyclohexyltrimethoxysilane interact with surfaces like glass fibers and what happens to this interaction in water?
A1: this compound (CHTMS) forms a strong bond with glass fibers through a process called hydrolysis and condensation. The methoxy groups (–OCH3) of CHTMS react with hydroxyl groups (–OH) present on the glass surface, releasing methanol and forming siloxane bonds (Si–O–Si). This creates a durable organic-inorganic interface. []
Q2: How does the incorporation of this compound impact the high-temperature friction properties of organosilica networks?
A2: Organosilica networks containing CHTMS exhibit promising high-temperature lubrication properties. When incorporated into these networks, CHTMS contributes to a reduction in friction coefficient, particularly at elevated temperatures. Studies have shown that materials derived from CHTMS start to soften above 120°C, leading to significantly reduced friction coefficients, reaching values as low as 0.01. This surpasses the performance of even commonly used solid lubricants like graphite. [] The cyclohexyl group in CHTMS likely plays a crucial role in this behavior, providing flexibility and facilitating movement within the material at high temperatures.
Q3: Are there any alternative organosilica precursors with comparable or superior performance to this compound in high-temperature lubrication applications?
A3: Research suggests that Phenyltrimethoxysilane (PTMS) exhibits comparable, and in some cases, superior performance to CHTMS in high-temperature lubrication applications. [] Similar to CHTMS, materials derived from PTMS also demonstrate softening behavior above 120°C and achieve similarly low friction coefficients (around 0.01). [] This suggests that the phenyl group in PTMS might offer similar or even enhanced flexibility and mobility at high temperatures compared to the cyclohexyl group in CHTMS. Further investigations into the specific structural features and their influence on high-temperature lubrication properties could provide valuable insights for designing even more effective solid lubricants.
Haftungsausschluss und Informationen zu In-Vitro-Forschungsprodukten
Bitte beachten Sie, dass alle Artikel und Produktinformationen, die auf BenchChem präsentiert werden, ausschließlich zu Informationszwecken bestimmt sind. Die auf BenchChem zum Kauf angebotenen Produkte sind speziell für In-vitro-Studien konzipiert, die außerhalb lebender Organismen durchgeführt werden. In-vitro-Studien, abgeleitet von dem lateinischen Begriff "in Glas", beinhalten Experimente, die in kontrollierten Laborumgebungen unter Verwendung von Zellen oder Geweben durchgeführt werden. Es ist wichtig zu beachten, dass diese Produkte nicht als Arzneimittel oder Medikamente eingestuft sind und keine Zulassung der FDA für die Vorbeugung, Behandlung oder Heilung von medizinischen Zuständen, Beschwerden oder Krankheiten erhalten haben. Wir müssen betonen, dass jede Form der körperlichen Einführung dieser Produkte in Menschen oder Tiere gesetzlich strikt untersagt ist. Es ist unerlässlich, sich an diese Richtlinien zu halten, um die Einhaltung rechtlicher und ethischer Standards in Forschung und Experiment zu gewährleisten.