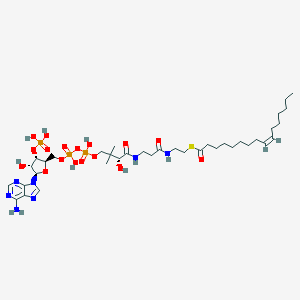
palmitoleoyl-CoA
Übersicht
Beschreibung
Palmitoleoyl-CoA (C16:1(n-7)) is a monounsaturated long-chain fatty acyl-CoA derived from the desaturation of palmitoyl-CoA (C16:0) by stearoyl-CoA desaturase 1 (SCD1) . This enzyme introduces a cis double bond at the Δ9 position, converting saturated palmitoyl-CoA into the monounsaturated this compound, which serves as a critical substrate for membrane phospholipids, triglycerides, and cholesterol esters . Its structural hallmark—a kinked conformation due to the cis double bond—enables unique interactions with enzymes and binding proteins, distinguishing it from saturated or polyunsaturated acyl-CoA counterparts .
Vorbereitungsmethoden
Chemical Synthesis of Palmitoleoyl-CoA
Acylimidazole Intermediate Method
The most widely cited chemical synthesis involves a two-step reaction using 1-acylimidazole intermediates. Developed by Kawaguchi et al., this method is adaptable to unsaturated fatty acids like palmitoleic acid (-9-hexadecenoic acid) and offers high yields (70–85%) even with small quantities of starting material .
Step 1: Formation of 1-Palmitoleoylimidazole
Palmitoleic acid reacts with carbonyldiimidazole (CDI) in anhydrous tetrahydrofuran (THF) under nitrogen atmosphere:
The reaction completes within 30 minutes at room temperature, with imidazole acting as a leaving group .
Step 2: Thioesterification with Coenzyme A
1-Palmitoleoylimidazole reacts with coenzyme A (CoA-SH) in a buffered aqueous solution (pH 8.0):
The product is isolated via high-performance liquid chromatography (HPLC) using a Shimadzu LC-PNH2 column with a potassium phosphate gradient (0.04–0.3 M, pH 3.3) .
Table 1: Yield Optimization for this compound Synthesis
Parameter | Optimal Condition | Yield (%) |
---|---|---|
Reaction Temperature | 25°C | 82 |
CoA-SH Molar Ratio | 1.2:1 (Acyl:CoA) | 78 |
Purification Method | HPLC (Phosphate Buffer) | 95 |
This method avoids enzymatic specificity issues and is ideal for synthesizing radiolabeled or rare acyl-CoA derivatives .
Enzymatic Synthesis Using Acyl-CoA Synthetases
ATP-Dependent Acyl Activation
This compound can be enzymatically synthesized using acyl-CoA synthetases (ACS), which catalyze the reaction:
The reaction proceeds via a palmitoleoyl-adenylate intermediate, with pyrophosphate hydrolysis driving completion .
Key Considerations:
-
Enzyme Source: Recombinant ACS from Escherichia coli (e.g., FadD) shows broad substrate specificity, including unsaturated C16 fatty acids .
-
Cofactors: Mg (5 mM) and ATP (10 mM) are required for activity .
-
Product Inhibition: High concentrations of this compound inhibit ACS, necessitating real-time monitoring using spectrophotometry (absorbance at 232 nm) .
Table 2: Enzymatic vs. Chemical Synthesis Comparison
Metric | Chemical Method | Enzymatic Method |
---|---|---|
Reaction Time | 2–3 hours | 30–60 minutes |
Purity | >95% (HPLC) | 80–90% (Centrifugation) |
Scalability | Microgram to gram scale | Limited by enzyme cost |
Purification and Quality Control
High-Performance Liquid Chromatography (HPLC)
HPLC remains the gold standard for purifying this compound. A Shimadzu LC-PNH2 column with a phosphate gradient (pH 3.3) resolves this compound from contaminants like unreacted CoA and imidazole . The elution profile shows a distinct peak at 12–14 minutes, confirmed by UV absorbance at 260 nm (CoA moiety) and 232 nm (thioester bond) .
Spectrophotometric Validation
This compound concentration is determined using:
{232} - A{260}}{4.5 \times 10^3 \, \text{M}^{-1}\text{cm}^{-1}}
Alkaline hydrolysis (0.1 N NaOH, 95°C, 1 minute) destroys the thioester bond, causing a >90% drop in , confirming product integrity .
Challenges and Innovations
Microscale Synthesis for Metabolic Studies
Recent advances enable synthesis of -labeled this compound using 1-acylimidazole methods, facilitating NMR-based metabolic tracing .
Analyse Chemischer Reaktionen
Types of Reactions: Palmitoleoyl-CoA undergoes various chemical reactions, including:
Oxidation: this compound can be oxidized to produce acetyl-CoA units through the process of β-oxidation.
Reduction: It can be reduced to form saturated fatty acyl-CoA derivatives.
Substitution: this compound can participate in substitution reactions, where the acyl group is transferred to other molecules.
Common Reagents and Conditions:
Oxidation: Requires enzymes such as acyl-CoA dehydrogenase, enoyl-CoA hydratase, and β-hydroxyacyl-CoA dehydrogenase.
Reduction: Involves the use of reducing agents like NADPH.
Substitution: Catalyzed by acyltransferases.
Major Products:
Oxidation: Acetyl-CoA units.
Reduction: Saturated fatty acyl-CoA derivatives.
Substitution: Various acylated molecules.
Wissenschaftliche Forschungsanwendungen
Metabolic Regulation
Palmitoleoyl-CoA is involved in metabolic regulation, particularly in the modulation of insulin sensitivity and glucose metabolism. Research indicates that palmitoleic acid, the fatty acid from which this compound is derived, enhances insulin sensitivity in adipocytes and liver cells. This effect is attributed to the activation of signaling pathways that promote glucose uptake and lipid metabolism.
Case Study: Insulin Sensitivity
- Study : A study demonstrated that palmitoleic acid supplementation improved insulin sensitivity in obese mice.
- Findings : Mice receiving palmitoleic acid showed increased glucose uptake and reduced plasma insulin levels compared to controls, indicating a potential role for this compound in metabolic health .
Cell Signaling
This compound acts as a signaling molecule influencing various cellular processes. It participates in the post-translational modification of proteins through a process known as palmitoylation, which affects protein localization and function.
Therapeutic Potential
The therapeutic applications of this compound are being explored in various contexts, particularly concerning metabolic disorders and inflammation.
Table 1: Therapeutic Applications of this compound
Inhibition Studies
Research has shown that this compound can inhibit specific enzymes involved in lipid metabolism. For instance, it has been observed to inhibit lipoxygenases (LOX), which are implicated in inflammatory processes.
Inhibition Data
- Study : this compound was tested against human lipoxygenases.
- Results : It exhibited an IC50 value of approximately 2.0 µM against h5-LOX, indicating its potential as an anti-inflammatory agent .
Lipid Metabolism
This compound plays a crucial role in lipid metabolism by participating in β-oxidation processes within mitochondria. It serves as a substrate for various acyl-CoA dehydrogenases involved in fatty acid oxidation.
Mechanistic Insights
Wirkmechanismus
Palmitoleoyl-CoA exerts its effects through its role as an acyl group carrier in fatty acid metabolism. It participates in the β-oxidation pathway, where it undergoes sequential cleavage reactions to generate acetyl-CoA units for energy production . Additionally, it acts as an allosteric regulator of key metabolic enzymes, influencing cellular signaling pathways and gene expression .
Vergleich Mit ähnlichen Verbindungen
Substrate Specificity in Enzymatic Reactions
Palmitoleoyl-CoA exhibits distinct substrate preferences compared to other acyl-CoAs in key metabolic pathways:
- Key Findings: PORCN Selectivity: Human PORCN (hPORCN) shows 3–5-fold higher activity for this compound (C16:1) over palmitoyl-CoA (C16:0) and oleoyl-CoA (C18:1) due to a bent binding cavity that accommodates the kinked C16:1 chain . SCD1 Dynamics: While SCD1 primarily uses palmitoyl-CoA and stearoyl-CoA as substrates, its activity is reduced by 35% in SCD1−/− mice, underscoring its role in this compound biosynthesis . Hhat Flexibility: Hedgehog acyltransferase (Hhat) utilizes this compound, palmitoyl-CoA, and oleoyl-CoA with comparable turnover rates, indicating broad substrate tolerance .
Structural and Functional Differences
The cis double bond in this compound (C16:1) induces a 90° kink, contrasting with the linear structure of saturated acyl-CoAs (e.g., palmitoyl-CoA) or the single double bond in oleoyl-CoA (C18:1(n-9)). This structural feature:
- Enhances Binding to Proteins: PORCN and Notum recognize the kinked conformation via bent cavities, enabling selective interactions .
- Affects Membrane Fluidity: As a monounsaturated fatty acid (MUFA), this compound increases membrane fluidity compared to saturated palmitoyl-CoA .
Metabolic Roles and Regulatory Effects
- Diabetes Studies : In diabetic mice, rosiglitazone reduced this compound levels to 29% of controls, while LG268 increased it to 231%, highlighting its role in insulin signaling .
- Cellular Concentrations : In HeLa cells, this compound concentrations nearly match palmitoyl-CoA levels, despite being a poorer substrate for certain enzymes (e.g., 15% activity relative to palmitoyl-CoA in one assay) .
Inhibition and Competition in Metabolic Pathways
- Condensation Reactions: this compound noncompetitively inhibits palmitoyl-CoA condensation in hepatic microsomes, suggesting separate enzymes for saturated vs. unsaturated acyl-CoA processing .
- Dietary Effects : High-carbohydrate diets increase this compound condensation activity 2.5-fold, whereas di-(2-ethylhexyl)phthalate (DEHP) reduces it to 63% .
Biologische Aktivität
Palmitoleoyl-CoA, a long-chain unsaturated fatty acyl-CoA, plays a significant role in various metabolic pathways and cellular functions. This article delves into its biological activity, focusing on mechanisms of action, physiological effects, and its implications in health and disease.
Overview of this compound
This compound (C16:1) is formed from palmitoleic acid and coenzyme A. It is involved in lipid metabolism and serves as a substrate for various enzymatic reactions, including fatty acid elongation and desaturation. Its structural formula is represented as follows:
-
AMP-Activated Protein Kinase (AMPK) Activation :
- This compound has been shown to activate AMPK, a crucial energy sensor in cells. Studies indicate that it increases AMPK activity by approximately threefold at physiological concentrations (around 1 μM) without requiring the presence of AMP, suggesting a unique activation mechanism distinct from traditional AMPK activators .
- The activation occurs independently of the γ-subunit AMP binding sites, indicating that this compound may bind to the allosteric activation site (ADaM site) on the β1-subunit of AMPK .
-
Regulation of Lipid Metabolism :
- As an acyl-CoA derivative, this compound plays a pivotal role in the regulation of lipid metabolism. It influences the activity of enzymes such as carnitine palmitoyltransferase I (CPT I), which is essential for fatty acid oxidation .
- The compound has been implicated in the modulation of lipogenesis and lipolysis in adipose tissue, thereby affecting overall energy homeostasis.
Physiological Effects
This compound exhibits several physiological effects that are critical for maintaining metabolic balance:
- Insulin Sensitivity : Research indicates that this compound may enhance insulin sensitivity in muscle and liver tissues, potentially offering protective effects against insulin resistance .
- Inflammation Modulation : It has been suggested that this compound can modulate inflammatory responses through its interaction with various signaling pathways, including those involving cyclooxygenases (COX) and lipoxygenases (LOX) .
Case Studies
- Study on Hepatocytes :
- Impact on Mitochondrial Function :
Comparative Analysis
The following table summarizes the biological activities and effects of various acyl-CoAs compared to this compound:
Acyl-CoA | AMPK Activation | Insulin Sensitivity | Inflammatory Modulation | Notes |
---|---|---|---|---|
This compound | Yes | Yes | Yes | Unique binding to AMPK β1-subunit |
Palmitoyl-CoA | Yes | Moderate | Yes | More potent than this compound |
Oleoyl-CoA | Yes | High | Moderate | Stronger anti-inflammatory properties |
Stearoyl-CoA | Moderate | Low | Low | Less effective in metabolic regulation |
Q & A
Basic Research Questions
Q. What is the role of palmitoleoyl-CoA in unsaturated fatty acid biosynthesis, and how can researchers experimentally validate its involvement?
this compound (C16:1-CoA) is a key intermediate in the biosynthesis of unsaturated fatty acids. In humans, it participates in the hsa01040 pathway (unsaturated fatty acid biosynthesis) via the enzymatic desaturation of palmitoyl-CoA by Δ9-desaturases (e.g., stearoyl-CoA desaturase). Researchers can validate its role by:
- Enzyme activity assays : Measuring Δ9-desaturase activity using radiolabeled palmitoyl-CoA and tracking the conversion to this compound via thin-layer chromatography (TLC) or mass spectrometry (LC-MS/MS) .
- Gene knockdown/knockout models : Silencing SCD1 (stearoyl-CoA desaturase 1) in cell lines and quantifying this compound levels using targeted metabolomics .
Q. What are the most reliable methods for detecting and quantifying this compound in cellular samples?
Detection requires specialized techniques due to its labile thioester bond and low abundance:
- Liquid chromatography-tandem mass spectrometry (LC-MS/MS) : Optimize extraction with acidic conditions (e.g., 0.1% formic acid in methanol) to stabilize the CoA-thioester. Use reverse-phase columns (C18) and multiple reaction monitoring (MRM) for specificity .
- Isotope dilution assays : Spike samples with deuterated this compound as an internal standard to correct for matrix effects .
Advanced Research Questions
Q. How can researchers experimentally resolve contradictions in this compound’s role across different metabolic models (e.g., in vitro vs. in vivo studies)?
Discrepancies often arise due to differences in substrate availability, compartmentalization, or enzyme kinetics. Mitigate these by:
- Compartment-specific profiling : Isolate mitochondria, ER, or cytosol to measure localized this compound pools .
- Tracer studies : Use -labeled palmitate to track flux through desaturation pathways in live cells or animal models .
- Computational modeling : Integrate enzyme kinetics (e.g., Km for Δ9-desaturase) with tissue-specific lipidomics data to predict in vivo behavior .
Q. What experimental strategies can elucidate the structural basis of this compound’s interaction with enzymes like PORCN in Wnt signaling?
PORCN catalyzes Wnt protein acylation using this compound as a substrate. Key approaches include:
- Cryo-EM studies : Resolve the ternary complex of PORCN, Wnt3a, and this compound to identify binding interfaces and catalytic residues (e.g., histidine-mediated hydroxyl activation) .
- Site-directed mutagenesis : Test mutations in PORCN’s catalytic pocket (e.g., H341A) to disrupt this compound binding and validate via acyltransferase activity assays .
- Competitive inhibition assays : Co-incubate PORCN with inhibitors like LGK974 and this compound to quantify displacement kinetics .
Q. How should researchers design experiments to investigate this compound’s regulatory effects on lipid metabolism in disease models (e.g., cancer or metabolic syndrome)?
A robust design includes:
- Disease-relevant models : Use SCD1<sup>-/-</sup> mice or cancer xenografts with altered Wnt signaling to study this compound’s impact on tumor growth or insulin resistance .
- Multi-omics integration : Combine lipidomics (this compound levels), transcriptomics (Δ9-desaturase expression), and proteomics (Wnt pathway activation) .
- Pharmacological modulation : Treat models with Δ9-desaturase inhibitors (e.g., A939572) or PORCN inhibitors (e.g., LGK974) to dissect pathway dependencies .
Q. What methodological considerations are critical when analyzing contradictory data on this compound’s pro- vs. anti-inflammatory roles?
Contradictions may stem from cell-type specificity or concentration-dependent effects. Address this by:
- Dose-response profiling : Titrate this compound in macrophage or adipocyte cultures and measure cytokine output (e.g., IL-6, TNF-α) .
- Receptor interaction studies : Use surface plasmon resonance (SPR) to test binding to receptors like GPR120, which mediates anti-inflammatory effects .
- In vivo validation : Administer this compound to GPR120<sup>-/-</sup> mice and assess inflammation markers .
Q. Methodological Best Practices
- Sample preparation : Avoid freeze-thaw cycles and use antioxidants (e.g., butylated hydroxytoluene) to prevent CoA-thioester degradation .
- Data interpretation : Normalize this compound levels to total CoASH or protein content to account for batch variability .
- Ethical compliance : For animal studies, adhere to protocols for humane endpoints and metabolic phenotyping (e.g., indirect calorimetry) .
Eigenschaften
IUPAC Name |
S-[2-[3-[[(2R)-4-[[[(2R,3S,4R,5R)-5-(6-aminopurin-9-yl)-4-hydroxy-3-phosphonooxyoxolan-2-yl]methoxy-hydroxyphosphoryl]oxy-hydroxyphosphoryl]oxy-2-hydroxy-3,3-dimethylbutanoyl]amino]propanoylamino]ethyl] (Z)-hexadec-9-enethioate | |
---|---|---|
Source | PubChem | |
URL | https://pubchem.ncbi.nlm.nih.gov | |
Description | Data deposited in or computed by PubChem | |
InChI |
InChI=1S/C37H64N7O17P3S/c1-4-5-6-7-8-9-10-11-12-13-14-15-16-17-28(46)65-21-20-39-27(45)18-19-40-35(49)32(48)37(2,3)23-58-64(55,56)61-63(53,54)57-22-26-31(60-62(50,51)52)30(47)36(59-26)44-25-43-29-33(38)41-24-42-34(29)44/h9-10,24-26,30-32,36,47-48H,4-8,11-23H2,1-3H3,(H,39,45)(H,40,49)(H,53,54)(H,55,56)(H2,38,41,42)(H2,50,51,52)/b10-9-/t26-,30-,31-,32+,36-/m1/s1 | |
Source | PubChem | |
URL | https://pubchem.ncbi.nlm.nih.gov | |
Description | Data deposited in or computed by PubChem | |
InChI Key |
QBYOCCWNZAOZTL-MDMKAECGSA-N | |
Source | PubChem | |
URL | https://pubchem.ncbi.nlm.nih.gov | |
Description | Data deposited in or computed by PubChem | |
Canonical SMILES |
CCCCCCC=CCCCCCCCC(=O)SCCNC(=O)CCNC(=O)C(C(C)(C)COP(=O)(O)OP(=O)(O)OCC1C(C(C(O1)N2C=NC3=C(N=CN=C32)N)O)OP(=O)(O)O)O | |
Source | PubChem | |
URL | https://pubchem.ncbi.nlm.nih.gov | |
Description | Data deposited in or computed by PubChem | |
Isomeric SMILES |
CCCCCC/C=C\CCCCCCCC(=O)SCCNC(=O)CCNC(=O)[C@@H](C(C)(C)COP(=O)(O)OP(=O)(O)OC[C@@H]1[C@H]([C@H]([C@@H](O1)N2C=NC3=C(N=CN=C32)N)O)OP(=O)(O)O)O | |
Source | PubChem | |
URL | https://pubchem.ncbi.nlm.nih.gov | |
Description | Data deposited in or computed by PubChem | |
Molecular Formula |
C37H64N7O17P3S | |
Source | PubChem | |
URL | https://pubchem.ncbi.nlm.nih.gov | |
Description | Data deposited in or computed by PubChem | |
DSSTOX Substance ID |
DTXSID801316783 | |
Record name | Palmitoleoyl-CoA | |
Source | EPA DSSTox | |
URL | https://comptox.epa.gov/dashboard/DTXSID801316783 | |
Description | DSSTox provides a high quality public chemistry resource for supporting improved predictive toxicology. | |
Molecular Weight |
1003.9 g/mol | |
Source | PubChem | |
URL | https://pubchem.ncbi.nlm.nih.gov | |
Description | Data deposited in or computed by PubChem | |
Physical Description |
Solid | |
Record name | Palmitoleyl-CoA | |
Source | Human Metabolome Database (HMDB) | |
URL | http://www.hmdb.ca/metabolites/HMDB0006532 | |
Description | The Human Metabolome Database (HMDB) is a freely available electronic database containing detailed information about small molecule metabolites found in the human body. | |
Explanation | HMDB is offered to the public as a freely available resource. Use and re-distribution of the data, in whole or in part, for commercial purposes requires explicit permission of the authors and explicit acknowledgment of the source material (HMDB) and the original publication (see the HMDB citing page). We ask that users who download significant portions of the database cite the HMDB paper in any resulting publications. | |
CAS No. |
18198-76-0 | |
Record name | Palmitoleoyl-CoA | |
Source | CAS Common Chemistry | |
URL | https://commonchemistry.cas.org/detail?cas_rn=18198-76-0 | |
Description | CAS Common Chemistry is an open community resource for accessing chemical information. Nearly 500,000 chemical substances from CAS REGISTRY cover areas of community interest, including common and frequently regulated chemicals, and those relevant to high school and undergraduate chemistry classes. This chemical information, curated by our expert scientists, is provided in alignment with our mission as a division of the American Chemical Society. | |
Explanation | The data from CAS Common Chemistry is provided under a CC-BY-NC 4.0 license, unless otherwise stated. | |
Record name | Palmitoleoyl-CoA | |
Source | EPA DSSTox | |
URL | https://comptox.epa.gov/dashboard/DTXSID801316783 | |
Description | DSSTox provides a high quality public chemistry resource for supporting improved predictive toxicology. | |
Record name | Palmitoleyl-CoA | |
Source | Human Metabolome Database (HMDB) | |
URL | http://www.hmdb.ca/metabolites/HMDB0006532 | |
Description | The Human Metabolome Database (HMDB) is a freely available electronic database containing detailed information about small molecule metabolites found in the human body. | |
Explanation | HMDB is offered to the public as a freely available resource. Use and re-distribution of the data, in whole or in part, for commercial purposes requires explicit permission of the authors and explicit acknowledgment of the source material (HMDB) and the original publication (see the HMDB citing page). We ask that users who download significant portions of the database cite the HMDB paper in any resulting publications. | |
Retrosynthesis Analysis
AI-Powered Synthesis Planning: Our tool employs the Template_relevance Pistachio, Template_relevance Bkms_metabolic, Template_relevance Pistachio_ringbreaker, Template_relevance Reaxys, Template_relevance Reaxys_biocatalysis model, leveraging a vast database of chemical reactions to predict feasible synthetic routes.
One-Step Synthesis Focus: Specifically designed for one-step synthesis, it provides concise and direct routes for your target compounds, streamlining the synthesis process.
Accurate Predictions: Utilizing the extensive PISTACHIO, BKMS_METABOLIC, PISTACHIO_RINGBREAKER, REAXYS, REAXYS_BIOCATALYSIS database, our tool offers high-accuracy predictions, reflecting the latest in chemical research and data.
Strategy Settings
Precursor scoring | Relevance Heuristic |
---|---|
Min. plausibility | 0.01 |
Model | Template_relevance |
Template Set | Pistachio/Bkms_metabolic/Pistachio_ringbreaker/Reaxys/Reaxys_biocatalysis |
Top-N result to add to graph | 6 |
Feasible Synthetic Routes
Haftungsausschluss und Informationen zu In-Vitro-Forschungsprodukten
Bitte beachten Sie, dass alle Artikel und Produktinformationen, die auf BenchChem präsentiert werden, ausschließlich zu Informationszwecken bestimmt sind. Die auf BenchChem zum Kauf angebotenen Produkte sind speziell für In-vitro-Studien konzipiert, die außerhalb lebender Organismen durchgeführt werden. In-vitro-Studien, abgeleitet von dem lateinischen Begriff "in Glas", beinhalten Experimente, die in kontrollierten Laborumgebungen unter Verwendung von Zellen oder Geweben durchgeführt werden. Es ist wichtig zu beachten, dass diese Produkte nicht als Arzneimittel oder Medikamente eingestuft sind und keine Zulassung der FDA für die Vorbeugung, Behandlung oder Heilung von medizinischen Zuständen, Beschwerden oder Krankheiten erhalten haben. Wir müssen betonen, dass jede Form der körperlichen Einführung dieser Produkte in Menschen oder Tiere gesetzlich strikt untersagt ist. Es ist unerlässlich, sich an diese Richtlinien zu halten, um die Einhaltung rechtlicher und ethischer Standards in Forschung und Experiment zu gewährleisten.