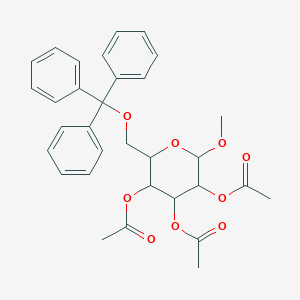
Methyl 2,3,4-tri-O-acetyl-6-O-trityl-A-D-glucopyranoside
- Klicken Sie auf QUICK INQUIRY, um ein Angebot von unserem Expertenteam zu erhalten.
- Mit qualitativ hochwertigen Produkten zu einem WETTBEWERBSFÄHIGEN Preis können Sie sich mehr auf Ihre Forschung konzentrieren.
Übersicht
Beschreibung
Methyl 2,3,4-tri-O-acetyl-6-O-trityl-A-D-glucopyranoside: is a derivative of glucopyranoside, a type of sugar molecule. This compound is characterized by the presence of acetyl and trityl groups, which are protective groups commonly used in organic synthesis to protect hydroxyl groups during chemical reactions. The molecular formula of this compound is C32H34O9 , and it has a molecular weight of 562.61 g/mol .
Vorbereitungsmethoden
Synthetic Routes and Reaction Conditions: The synthesis of Methyl 2,3,4-tri-O-acetyl-6-O-trityl-A-D-glucopyranoside typically involves the protection of the hydroxyl groups of glucopyranoside. The process begins with the acetylation of the hydroxyl groups at positions 2, 3, and 4 using acetic anhydride in the presence of a catalyst such as pyridine. The trityl group is then introduced at the 6-position using trityl chloride in the presence of a base like triethylamine .
Industrial Production Methods: Industrial production of this compound follows similar synthetic routes but on a larger scale. The reaction conditions are optimized to ensure high yield and purity. The use of automated reactors and continuous flow systems can enhance the efficiency and scalability of the production process.
Analyse Chemischer Reaktionen
Types of Reactions: Methyl 2,3,4-tri-O-acetyl-6-O-trityl-A-D-glucopyranoside undergoes various chemical reactions, including:
Hydrolysis: The acetyl and trityl protective groups can be removed through hydrolysis using acidic or basic conditions.
Oxidation: The compound can undergo oxidation reactions, particularly at the hydroxyl groups, leading to the formation of corresponding ketones or aldehydes.
Substitution: The acetyl groups can be substituted with other protective groups or functional groups through nucleophilic substitution reactions.
Common Reagents and Conditions:
Hydrolysis: Acidic hydrolysis can be performed using hydrochloric acid or sulfuric acid, while basic hydrolysis can be achieved using sodium hydroxide or potassium hydroxide.
Oxidation: Common oxidizing agents include potassium permanganate, chromium trioxide, and pyridinium chlorochromate.
Substitution: Nucleophiles such as amines, thiols, or alcohols can be used for substitution reactions under appropriate conditions.
Major Products Formed:
Hydrolysis: The major products are glucopyranoside and acetic acid.
Oxidation: The major products are ketones or aldehydes, depending on the specific oxidation conditions.
Substitution: The major products are glucopyranoside derivatives with new functional groups replacing the acetyl groups.
Wissenschaftliche Forschungsanwendungen
Methyl 2,3,4-tri-O-acetyl-6-O-trityl-A-D-glucopyranoside has several scientific research applications:
Chemistry: It is used as an intermediate in the synthesis of complex carbohydrates and glycosides. The protective groups facilitate selective reactions at specific hydroxyl positions.
Biology: The compound is used in the study of carbohydrate metabolism and enzyme interactions. It serves as a model compound for understanding the behavior of glucopyranosides in biological systems.
Medicine: It has potential applications in drug development, particularly in the design of prodrugs and drug delivery systems. The protective groups can be used to modify the pharmacokinetic properties of drugs.
Industry: The compound is used in the production of specialty chemicals and materials. It serves as a building block for the synthesis of various functionalized glucopyranosides.
Wirkmechanismus
The mechanism of action of Methyl 2,3,4-tri-O-acetyl-6-O-trityl-A-D-glucopyranoside involves the selective protection and deprotection of hydroxyl groups. The acetyl and trityl groups protect the hydroxyl groups from unwanted reactions during synthetic processes. The deprotection step, which involves the removal of these groups, allows for the subsequent functionalization of the hydroxyl groups. The molecular targets and pathways involved depend on the specific reactions and applications of the compound.
Vergleich Mit ähnlichen Verbindungen
- Methyl 2,3,4-tri-O-acetyl-6-O-trityl-α-D-galactopyranoside
- Methyl 2,3,4-tri-O-acetyl-6-O-nitro-α-D-galactopyranoside
- Methyl 2,3,4-tri-O-benzyl-α-D-glucopyranoside
Comparison:
- Methyl 2,3,4-tri-O-acetyl-6-O-trityl-α-D-galactopyranoside: This compound is similar in structure but differs in the configuration of the sugar moiety. It has applications in biomedicine, particularly in the treatment of cancer and viral infections.
- Methyl 2,3,4-tri-O-acetyl-6-O-nitro-α-D-galactopyranoside: This compound contains a nitro group instead of a trityl group. It is used in chemical synthesis and has different reactivity and applications compared to the trityl derivative .
- Methyl 2,3,4-tri-O-benzyl-α-D-glucopyranoside: This compound has benzyl groups instead of acetyl groups. It is used in carbohydrate chemistry and has different protective properties compared to the acetyl derivative .
Methyl 2,3,4-tri-O-acetyl-6-O-trityl-A-D-glucopyranoside stands out due to its unique combination of acetyl and trityl protective groups, which provide selective protection and deprotection of hydroxyl groups, making it a valuable intermediate in synthetic chemistry.
Eigenschaften
IUPAC Name |
[(2R,3R,4S,5R,6S)-4,5-diacetyloxy-6-methoxy-2-(trityloxymethyl)oxan-3-yl] acetate |
Source
|
---|---|---|
Source | PubChem | |
URL | https://pubchem.ncbi.nlm.nih.gov | |
Description | Data deposited in or computed by PubChem | |
InChI |
InChI=1S/C32H34O9/c1-21(33)38-28-27(41-31(36-4)30(40-23(3)35)29(28)39-22(2)34)20-37-32(24-14-8-5-9-15-24,25-16-10-6-11-17-25)26-18-12-7-13-19-26/h5-19,27-31H,20H2,1-4H3/t27-,28-,29+,30-,31+/m1/s1 |
Source
|
Source | PubChem | |
URL | https://pubchem.ncbi.nlm.nih.gov | |
Description | Data deposited in or computed by PubChem | |
InChI Key |
CEIHIENXWVDRTC-SAEUYMBFSA-N |
Source
|
Source | PubChem | |
URL | https://pubchem.ncbi.nlm.nih.gov | |
Description | Data deposited in or computed by PubChem | |
Canonical SMILES |
CC(=O)OC1C(OC(C(C1OC(=O)C)OC(=O)C)OC)COC(C2=CC=CC=C2)(C3=CC=CC=C3)C4=CC=CC=C4 |
Source
|
Source | PubChem | |
URL | https://pubchem.ncbi.nlm.nih.gov | |
Description | Data deposited in or computed by PubChem | |
Isomeric SMILES |
CC(=O)O[C@@H]1[C@H](O[C@@H]([C@@H]([C@H]1OC(=O)C)OC(=O)C)OC)COC(C2=CC=CC=C2)(C3=CC=CC=C3)C4=CC=CC=C4 |
Source
|
Source | PubChem | |
URL | https://pubchem.ncbi.nlm.nih.gov | |
Description | Data deposited in or computed by PubChem | |
Molecular Formula |
C32H34O9 |
Source
|
Source | PubChem | |
URL | https://pubchem.ncbi.nlm.nih.gov | |
Description | Data deposited in or computed by PubChem | |
Molecular Weight |
562.6 g/mol |
Source
|
Source | PubChem | |
URL | https://pubchem.ncbi.nlm.nih.gov | |
Description | Data deposited in or computed by PubChem | |
Haftungsausschluss und Informationen zu In-Vitro-Forschungsprodukten
Bitte beachten Sie, dass alle Artikel und Produktinformationen, die auf BenchChem präsentiert werden, ausschließlich zu Informationszwecken bestimmt sind. Die auf BenchChem zum Kauf angebotenen Produkte sind speziell für In-vitro-Studien konzipiert, die außerhalb lebender Organismen durchgeführt werden. In-vitro-Studien, abgeleitet von dem lateinischen Begriff "in Glas", beinhalten Experimente, die in kontrollierten Laborumgebungen unter Verwendung von Zellen oder Geweben durchgeführt werden. Es ist wichtig zu beachten, dass diese Produkte nicht als Arzneimittel oder Medikamente eingestuft sind und keine Zulassung der FDA für die Vorbeugung, Behandlung oder Heilung von medizinischen Zuständen, Beschwerden oder Krankheiten erhalten haben. Wir müssen betonen, dass jede Form der körperlichen Einführung dieser Produkte in Menschen oder Tiere gesetzlich strikt untersagt ist. Es ist unerlässlich, sich an diese Richtlinien zu halten, um die Einhaltung rechtlicher und ethischer Standards in Forschung und Experiment zu gewährleisten.