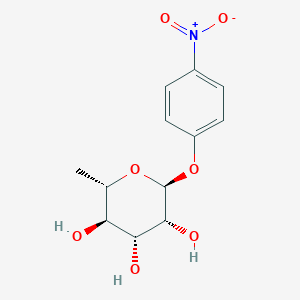
4-Nitrophenyl-α-L-rhamnopyranosid
Übersicht
Beschreibung
4-Nitrophenylrhamnoside is a chemical compound that belongs to the class of nitrophenyl glycosides. It is characterized by the presence of a nitro group attached to a phenyl ring, which is further linked to a rhamnose sugar moiety. This compound is often used as a substrate in enzymatic assays to measure the activity of specific enzymes, such as α-L-rhamnosidase .
Wissenschaftliche Forschungsanwendungen
4-Nitrophenylrhamnoside has a wide range of applications in scientific research:
Enzymatic Assays: Used as a substrate to measure the activity of α-L-rhamnosidase and other glycosidases.
Biotechnology: Employed in the biotransformation of flavonoids and other glycosides for improved bioavailability and therapeutic efficacy.
Pharmaceuticals: Utilized in the development of enzyme-activated prodrugs for targeted drug delivery.
Wirkmechanismus
Target of Action
4-Nitrophenylrhamnoside, also known as 4-Nitrophenyl-alpha-L-rhamnoside, is primarily a substrate for the enzyme α-L-rhamnosidase . This enzyme plays a crucial role in the hydrolysis of rhamnose residues in various natural compounds .
Mode of Action
The compound interacts with α-L-rhamnosidase, which catalyzes its hydrolysis. This enzymatic reaction results in the production of 4-nitrophenol .
Biochemical Pathways
It’s known that the compound serves as a substrate in the enzymatic reactions involving α-l-rhamnosidase .
Pharmacokinetics
Its solubility in ethanol is reported to be between 4900-5100 mg/mL , which may influence its bioavailability.
Result of Action
The primary result of the action of 4-Nitrophenylrhamnoside is the production of 4-nitrophenol, which can be measured spectrophotometrically at 405 nm . This makes 4-Nitrophenylrhamnoside a useful tool in biochemical assays to measure the activity of α-L-rhamnosidase .
Action Environment
It’s known that the compound is stable at a storage temperature of -20°c .
Vorbereitungsmethoden
Synthetic Routes and Reaction Conditions: The synthesis of 4-Nitrophenylrhamnoside typically involves the glycosylation of rhamnose with 4-nitrophenol. This reaction can be catalyzed by various enzymes or chemical catalysts under controlled conditions. The reaction conditions often include the use of solvents like ethanol and specific temperature and pH settings to optimize the yield .
Industrial Production Methods: In an industrial setting, the production of 4-Nitrophenylrhamnoside may involve large-scale enzymatic processes. Enzymes such as α-L-rhamnosidase from microbial sources like Aspergillus niger or Saccharomyces cerevisiae are commonly employed. These enzymes facilitate the glycosylation process, ensuring high efficiency and yield .
Analyse Chemischer Reaktionen
Types of Reactions: 4-Nitrophenylrhamnoside undergoes several types of chemical reactions, including:
Common Reagents and Conditions:
Hydrolysis: Enzymatic hydrolysis using α-L-rhamnosidase at optimal pH and temperature conditions.
Reduction: Sodium borohydride in an aqueous or alcoholic medium.
Major Products:
Hydrolysis: 4-Nitrophenol and rhamnose.
Reduction: 4-Aminophenylrhamnoside.
Vergleich Mit ähnlichen Verbindungen
4-Nitrophenyl α-L-rhamnopyranoside: Shares a similar structure and is used for similar enzymatic assays.
4-Nitrophenyl β-D-glucopyranoside: Another nitrophenyl glycoside used to measure β-glucosidase activity.
Uniqueness: 4-Nitrophenylrhamnoside is unique due to its specific interaction with α-L-rhamnosidase, making it a valuable tool in studying the activity and specificity of this enzyme. Its structural motif allows for targeted applications in biotechnology and pharmaceuticals, particularly in the development of enzyme-activated prodrugs .
Biologische Aktivität
4-Nitrophenylrhamnoside, specifically 4-nitrophenyl α-L-rhamnopyranoside, is a compound of significant interest in biochemical research due to its role as a substrate for various enzymes and its potential biological activities. This article provides a detailed overview of its biological activity, including enzymatic interactions, antioxidant properties, and implications in medical research.
Chemical Structure and Synthesis
4-Nitrophenyl α-L-rhamnopyranoside is synthesized through a two-step process involving the acetylation and chlorination of L-rhamnose. The final product is characterized by its chromogenic property, which allows for the detection of enzyme activity through the release of para-nitrophenol upon hydrolysis. The crystal structure has been determined, revealing important stereochemical information that aids in understanding its interactions with enzymes .
Enzymatic Activity
Substrate for α-L-Rhamnosidase:
4-Nitrophenyl α-L-rhamnopyranoside serves as an important substrate for α-L-rhamnosidase, an enzyme that catalyzes the hydrolysis of rhamnosides. The enzymatic activity can be quantitatively measured by monitoring the release of para-nitrophenol, which exhibits a characteristic absorbance at 405 nm. This property is utilized in various assays to assess enzyme kinetics and substrate specificity .
Kinetic Studies:
Kinetic studies have shown that different rhamnosidases exhibit varying affinities towards 4-nitrophenyl α-L-rhamnopyranoside. For instance, the enzyme KoRha from the GH78 family has been characterized using this substrate, providing insights into its optimal pH and temperature conditions for maximum activity .
Antioxidant Activity
Recent studies have highlighted the antioxidant properties of compounds related to 4-nitrophenylrhamnoside. Antioxidant assays such as ABTS and FRAP demonstrate that derivatives of this compound can effectively scavenge free radicals and reduce oxidative stress markers. These properties suggest potential applications in preventing oxidative damage in biological systems .
Case Studies and Research Findings
Case Study on Enzyme Activity:
A notable case study involved the use of 4-nitrophenyl α-L-rhamnopyranoside to investigate the enzymatic activity of naringinase from various bacterial sources. The study found that different strains exhibited unique hydrolytic capabilities towards this substrate, emphasizing its utility in microbial enzyme characterization .
Research Findings:
Research has also focused on the implications of 4-nitrophenylrhamnoside in pharmacological contexts, particularly concerning glycosidase inhibition. Inhibitory assays have demonstrated its potential as a lead compound for developing treatments targeting hyperglycemia through α-glucosidase inhibition .
Summary of Biological Activities
Activity | Description |
---|---|
Enzymatic Substrate | Acts as a substrate for α-L-rhamnosidase; used to measure enzyme kinetics. |
Antioxidant Properties | Demonstrates significant radical scavenging ability; potential health benefits. |
Inhibition Studies | Shows promise in inhibiting glycosidases; potential applications in diabetes. |
Eigenschaften
IUPAC Name |
(2S,3R,4R,5R,6S)-2-methyl-6-(4-nitrophenoxy)oxane-3,4,5-triol | |
---|---|---|
Source | PubChem | |
URL | https://pubchem.ncbi.nlm.nih.gov | |
Description | Data deposited in or computed by PubChem | |
InChI |
InChI=1S/C12H15NO7/c1-6-9(14)10(15)11(16)12(19-6)20-8-4-2-7(3-5-8)13(17)18/h2-6,9-12,14-16H,1H3/t6-,9-,10+,11+,12-/m0/s1 | |
Source | PubChem | |
URL | https://pubchem.ncbi.nlm.nih.gov | |
Description | Data deposited in or computed by PubChem | |
InChI Key |
YILIDCGSXCGACV-UOAXWKJLSA-N | |
Source | PubChem | |
URL | https://pubchem.ncbi.nlm.nih.gov | |
Description | Data deposited in or computed by PubChem | |
Canonical SMILES |
CC1C(C(C(C(O1)OC2=CC=C(C=C2)[N+](=O)[O-])O)O)O | |
Source | PubChem | |
URL | https://pubchem.ncbi.nlm.nih.gov | |
Description | Data deposited in or computed by PubChem | |
Isomeric SMILES |
C[C@H]1[C@@H]([C@H]([C@H]([C@@H](O1)OC2=CC=C(C=C2)[N+](=O)[O-])O)O)O | |
Source | PubChem | |
URL | https://pubchem.ncbi.nlm.nih.gov | |
Description | Data deposited in or computed by PubChem | |
Molecular Formula |
C12H15NO7 | |
Source | PubChem | |
URL | https://pubchem.ncbi.nlm.nih.gov | |
Description | Data deposited in or computed by PubChem | |
DSSTOX Substance ID |
DTXSID20172314 | |
Record name | 4-Nitrophenylrhamnoside | |
Source | EPA DSSTox | |
URL | https://comptox.epa.gov/dashboard/DTXSID20172314 | |
Description | DSSTox provides a high quality public chemistry resource for supporting improved predictive toxicology. | |
Molecular Weight |
285.25 g/mol | |
Source | PubChem | |
URL | https://pubchem.ncbi.nlm.nih.gov | |
Description | Data deposited in or computed by PubChem | |
CAS No. |
18918-31-5 | |
Record name | 4-Nitrophenylrhamnoside | |
Source | ChemIDplus | |
URL | https://pubchem.ncbi.nlm.nih.gov/substance/?source=chemidplus&sourceid=0018918315 | |
Description | ChemIDplus is a free, web search system that provides access to the structure and nomenclature authority files used for the identification of chemical substances cited in National Library of Medicine (NLM) databases, including the TOXNET system. | |
Record name | 4-Nitrophenylrhamnoside | |
Source | EPA DSSTox | |
URL | https://comptox.epa.gov/dashboard/DTXSID20172314 | |
Description | DSSTox provides a high quality public chemistry resource for supporting improved predictive toxicology. | |
Retrosynthesis Analysis
AI-Powered Synthesis Planning: Our tool employs the Template_relevance Pistachio, Template_relevance Bkms_metabolic, Template_relevance Pistachio_ringbreaker, Template_relevance Reaxys, Template_relevance Reaxys_biocatalysis model, leveraging a vast database of chemical reactions to predict feasible synthetic routes.
One-Step Synthesis Focus: Specifically designed for one-step synthesis, it provides concise and direct routes for your target compounds, streamlining the synthesis process.
Accurate Predictions: Utilizing the extensive PISTACHIO, BKMS_METABOLIC, PISTACHIO_RINGBREAKER, REAXYS, REAXYS_BIOCATALYSIS database, our tool offers high-accuracy predictions, reflecting the latest in chemical research and data.
Strategy Settings
Precursor scoring | Relevance Heuristic |
---|---|
Min. plausibility | 0.01 |
Model | Template_relevance |
Template Set | Pistachio/Bkms_metabolic/Pistachio_ringbreaker/Reaxys/Reaxys_biocatalysis |
Top-N result to add to graph | 6 |
Feasible Synthetic Routes
Haftungsausschluss und Informationen zu In-Vitro-Forschungsprodukten
Bitte beachten Sie, dass alle Artikel und Produktinformationen, die auf BenchChem präsentiert werden, ausschließlich zu Informationszwecken bestimmt sind. Die auf BenchChem zum Kauf angebotenen Produkte sind speziell für In-vitro-Studien konzipiert, die außerhalb lebender Organismen durchgeführt werden. In-vitro-Studien, abgeleitet von dem lateinischen Begriff "in Glas", beinhalten Experimente, die in kontrollierten Laborumgebungen unter Verwendung von Zellen oder Geweben durchgeführt werden. Es ist wichtig zu beachten, dass diese Produkte nicht als Arzneimittel oder Medikamente eingestuft sind und keine Zulassung der FDA für die Vorbeugung, Behandlung oder Heilung von medizinischen Zuständen, Beschwerden oder Krankheiten erhalten haben. Wir müssen betonen, dass jede Form der körperlichen Einführung dieser Produkte in Menschen oder Tiere gesetzlich strikt untersagt ist. Es ist unerlässlich, sich an diese Richtlinien zu halten, um die Einhaltung rechtlicher und ethischer Standards in Forschung und Experiment zu gewährleisten.