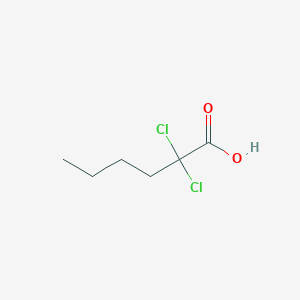
2,2-dichlorohexanoic Acid
Übersicht
Beschreibung
2,2-Dichlorohexanoic acid is an organic compound with the molecular formula C6H10Cl2O2 It is a derivative of hexanoic acid, where two chlorine atoms are substituted at the second carbon position
Vorbereitungsmethoden
Synthetic Routes and Reaction Conditions: The synthesis of 2,2-dichlorohexanoic acid typically involves the chlorination of hexanoic acid. One common method is the reaction of hexanoic acid with chlorine gas in the presence of a suitable catalyst, such as iron(III) chloride, under controlled temperature conditions. The reaction proceeds as follows:
C6H12O2+Cl2→C6H10Cl2O2+H2
Industrial Production Methods: In an industrial setting, the production of this compound can be scaled up using continuous flow reactors. This method ensures better control over reaction parameters, leading to higher yields and purity of the final product. The process involves the continuous feeding of hexanoic acid and chlorine gas into the reactor, with the reaction mixture being constantly stirred and maintained at an optimal temperature.
Analyse Chemischer Reaktionen
Types of Reactions: 2,2-Dichlorohexanoic acid undergoes various chemical reactions, including:
Oxidation: It can be oxidized to form corresponding ketones or carboxylic acids.
Reduction: Reduction reactions can convert it into less chlorinated derivatives.
Substitution: The chlorine atoms can be substituted with other functional groups through nucleophilic substitution reactions.
Common Reagents and Conditions:
Oxidation: Common oxidizing agents include potassium permanganate (KMnO4) and chromium trioxide (CrO3).
Reduction: Reducing agents such as lithium aluminum hydride (LiAlH4) are used.
Substitution: Nucleophiles like sodium hydroxide (NaOH) or ammonia (NH3) can be employed.
Major Products Formed:
Oxidation: Formation of hexanoic acid derivatives.
Reduction: Formation of partially chlorinated hexanoic acids.
Substitution: Formation of hexanoic acid derivatives with different functional groups.
Wissenschaftliche Forschungsanwendungen
2,2-Dichlorohexanoic acid has several applications in scientific research:
Chemistry: It is used as a reagent in organic synthesis and as an intermediate in the production of other chemicals.
Biology: It is studied for its potential effects on biological systems, including its role as a metabolic inhibitor.
Medicine: Research is ongoing to explore its potential therapeutic applications, particularly in the development of new drugs.
Industry: It is used in the manufacture of specialty chemicals and as a precursor for agrochemicals.
Wirkmechanismus
The mechanism of action of 2,2-dichlorohexanoic acid involves its interaction with specific molecular targets. It can inhibit certain enzymes by binding to their active sites, thereby affecting metabolic pathways. The presence of chlorine atoms enhances its reactivity, allowing it to participate in various biochemical reactions.
Vergleich Mit ähnlichen Verbindungen
- 2,2-Dichloropropanoic acid
- 2,2-Dichlorobutanoic acid
- 2,2-Dichloropentanoic acid
Comparison: Compared to these similar compounds, 2,2-dichlorohexanoic acid has a longer carbon chain, which can influence its physical and chemical properties. Its unique structure makes it more suitable for specific applications, particularly in industrial and research settings.
Biologische Aktivität
2,2-Dichlorohexanoic acid (DCHA) is a chlorinated organic compound with significant implications in environmental science and biological research. Its structure consists of a six-carbon chain with two chlorine atoms attached to the second carbon, which influences its reactivity and biological interactions. This article explores the biological activity of DCHA, focusing on its degradation pathways, toxicity, and potential applications in bioremediation.
- Molecular Formula : C₆H₈Cl₂O₂
- Molecular Weight : 183.04 g/mol
- CAS Number : 18240-70-5
Biological Activity Overview
DCHA exhibits various biological activities, primarily due to its halogenated structure, which can interact with biological systems in unique ways. The following sections detail its degradation by microorganisms, toxicity profiles, and potential for bioremediation.
Microbial Degradation
Research has shown that certain bacteria can utilize DCHA as a carbon source, leading to its biodegradation. A notable study isolated a bacterial strain identified as Enterobacter sp., which demonstrated the ability to degrade DCHA effectively. The strain was able to grow in minimal media containing DCHA, producing dehalogenase enzymes that facilitate the breakdown of the compound.
Key Findings :
- Enterobacter sp. showed a growth rate of 0.48 μmol Cl/mL when cultured with 20 mM DCHA.
- The strain was isolated from soil samples in agricultural areas where haloacids were previously used, indicating a natural adaptation to such environments .
Bacterial Strain | Growth Rate (μmol Cl/mL) | Optimal Concentration (mM) |
---|---|---|
Enterobacter sp. | 0.48 | 20 |
Toxicity and Environmental Impact
DCHA's toxicity has been a subject of concern due to its potential effects on human health and ecosystems. Studies indicate that exposure to DCHA can lead to metabolic disruptions in various organisms.
Toxicological Studies :
- In vitro studies have shown that DCHA can inhibit critical metabolic pathways in bacteria and higher organisms.
- The compound has been associated with dermal absorption risks, particularly among agricultural workers exposed to chlorinated herbicides .
Case Studies
- Bacterial Isolation and Characterization : A study conducted by Edbeib et al. (2020) focused on isolating bacteria capable of degrading haloalkanoic acids, including DCHA. The isolated Enterobacter sp. demonstrated significant potential for bioremediation applications due to its efficiency in degrading chlorinated compounds .
- Environmental Monitoring : Research highlighted the persistence of chlorinated compounds like DCHA in groundwater systems, emphasizing the need for effective biodegradation strategies to mitigate long-term environmental impacts .
Potential Applications
The ability of specific bacterial strains to degrade DCHA presents opportunities for bioremediation strategies aimed at detoxifying contaminated environments. Utilizing Enterobacter sp. as a bioremediation agent could enhance the detoxification processes for areas impacted by agricultural runoff containing chlorinated compounds.
Eigenschaften
IUPAC Name |
2,2-dichlorohexanoic acid | |
---|---|---|
Source | PubChem | |
URL | https://pubchem.ncbi.nlm.nih.gov | |
Description | Data deposited in or computed by PubChem | |
InChI |
InChI=1S/C6H10Cl2O2/c1-2-3-4-6(7,8)5(9)10/h2-4H2,1H3,(H,9,10) | |
Source | PubChem | |
URL | https://pubchem.ncbi.nlm.nih.gov | |
Description | Data deposited in or computed by PubChem | |
InChI Key |
CNWRQGGFKSSJSS-UHFFFAOYSA-N | |
Source | PubChem | |
URL | https://pubchem.ncbi.nlm.nih.gov | |
Description | Data deposited in or computed by PubChem | |
Canonical SMILES |
CCCCC(C(=O)O)(Cl)Cl | |
Source | PubChem | |
URL | https://pubchem.ncbi.nlm.nih.gov | |
Description | Data deposited in or computed by PubChem | |
Molecular Formula |
C6H10Cl2O2 | |
Source | PubChem | |
URL | https://pubchem.ncbi.nlm.nih.gov | |
Description | Data deposited in or computed by PubChem | |
DSSTOX Substance ID |
DTXSID10369175 | |
Record name | 2,2-dichlorohexanoic Acid | |
Source | EPA DSSTox | |
URL | https://comptox.epa.gov/dashboard/DTXSID10369175 | |
Description | DSSTox provides a high quality public chemistry resource for supporting improved predictive toxicology. | |
Molecular Weight |
185.05 g/mol | |
Source | PubChem | |
URL | https://pubchem.ncbi.nlm.nih.gov | |
Description | Data deposited in or computed by PubChem | |
CAS No. |
18240-70-5 | |
Record name | 2,2-dichlorohexanoic Acid | |
Source | EPA DSSTox | |
URL | https://comptox.epa.gov/dashboard/DTXSID10369175 | |
Description | DSSTox provides a high quality public chemistry resource for supporting improved predictive toxicology. | |
Retrosynthesis Analysis
AI-Powered Synthesis Planning: Our tool employs the Template_relevance Pistachio, Template_relevance Bkms_metabolic, Template_relevance Pistachio_ringbreaker, Template_relevance Reaxys, Template_relevance Reaxys_biocatalysis model, leveraging a vast database of chemical reactions to predict feasible synthetic routes.
One-Step Synthesis Focus: Specifically designed for one-step synthesis, it provides concise and direct routes for your target compounds, streamlining the synthesis process.
Accurate Predictions: Utilizing the extensive PISTACHIO, BKMS_METABOLIC, PISTACHIO_RINGBREAKER, REAXYS, REAXYS_BIOCATALYSIS database, our tool offers high-accuracy predictions, reflecting the latest in chemical research and data.
Strategy Settings
Precursor scoring | Relevance Heuristic |
---|---|
Min. plausibility | 0.01 |
Model | Template_relevance |
Template Set | Pistachio/Bkms_metabolic/Pistachio_ringbreaker/Reaxys/Reaxys_biocatalysis |
Top-N result to add to graph | 6 |
Feasible Synthetic Routes
Haftungsausschluss und Informationen zu In-Vitro-Forschungsprodukten
Bitte beachten Sie, dass alle Artikel und Produktinformationen, die auf BenchChem präsentiert werden, ausschließlich zu Informationszwecken bestimmt sind. Die auf BenchChem zum Kauf angebotenen Produkte sind speziell für In-vitro-Studien konzipiert, die außerhalb lebender Organismen durchgeführt werden. In-vitro-Studien, abgeleitet von dem lateinischen Begriff "in Glas", beinhalten Experimente, die in kontrollierten Laborumgebungen unter Verwendung von Zellen oder Geweben durchgeführt werden. Es ist wichtig zu beachten, dass diese Produkte nicht als Arzneimittel oder Medikamente eingestuft sind und keine Zulassung der FDA für die Vorbeugung, Behandlung oder Heilung von medizinischen Zuständen, Beschwerden oder Krankheiten erhalten haben. Wir müssen betonen, dass jede Form der körperlichen Einführung dieser Produkte in Menschen oder Tiere gesetzlich strikt untersagt ist. Es ist unerlässlich, sich an diese Richtlinien zu halten, um die Einhaltung rechtlicher und ethischer Standards in Forschung und Experiment zu gewährleisten.